Moderately volatile element behaviour at high temperature determined from nuclear detonation
Affiliations | Corresponding Author | Cite as | Funding information- Share this article
Article views:1,335Cumulative count of HTML views and PDF downloads.
- Download Citation
- Rights & Permissions
top
Abstract
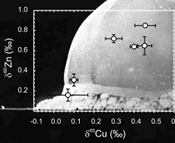
Figures and Tables
![]() Figure 1 Copper content versus δ65Cu in trinitite. Bulk silicate Earth (BSE) estimate from Savage et al. (2015). | ![]() Figure 2 (a) δ65Cu and (b) Cu abundance in trinitite with distance from the ‘Gadget’ compared with Zn, Pb and K contents from the same samples (Day et al., 2017; Chen et al., 2019). BSE is from Savage et al. (2015). | ![]() Figure 3 Copper versus (a) Zn and (b) K isotopes in trinitite. Zinc and K data for trinitites from Day et al. (2017) and Chen et al. (2019). BSE Cu, Zn and K values are from Moynier et al. (2017) and Wang and Jacobsen (2016). | ![]() Figure 4 Rayleigh distillation curves versus (a) Cu, (b) Zn and (c) K contents and isotopic compositions for trinitite glass, tektites and lunar samples. Curves are shown for different alpha fractionation (α) factors (1, 0.9998, 0.999, 0.998) established from trinitite, with 70 µg g-1 Cu, 30 µg g-1 Zn and 40000 µg g-1 K for estimated BSE isotopic compositions. Data are from this study, Day et al. (2017), and Chen et al. (2019) for trinitite. Tektite data are from Moynier et al. (2009, 2010), and Jiang et al. (2019), and lunar basalts data are from Paniello et al. (2012), Kato et al. (2015), and Wang and Jacobsen (2016) and references therein. |
Figure 1 | Figure 2 | Figure 3 | Figure 4 |
top
Introduction
The moderately volatile elements (MVE), including Cu, Zn and K, trace volatile behaviour in planetary bodies. The MVE have 50 % nebular condensation temperatures (TC50) at solar nebula conditions (10-4 bar) of between 1134 K and 665 K (Lodders, 2003
Lodders, K. (2003) Solar system abundances and condensation temperatures of the elements. The Astrophysical Journal 591, 1220.
), and have low element-element bond energies (Albarède et al., 2015Albarède, F., Albalat, E., Lee, C.T.A. (2015) An intrinsic volatility scale relevant to the Earth and Moon and the status of water in the Moon. Meteoritics and Planetary Science 50, 568-577.
). Stable isotope MVE compositions have been utilised to examine fractionation effects induced by evaporation or condensation, and to assess stable isotope differences between Earth and the Moon (e.g., Paniello et al., 2012Paniello, R.C., Day, J.M.D., Moynier, F. (2012) Zinc isotopic evidence for the origin of the Moon. Nature 490, 376-379.
; Day and Moynier, 2014Day, J.M.D., Moynier, F. (2014) Evaporative fractionation of volatile stable isotopes and their bearing on the origin of the Moon. Philosophical Transactions of the Royal Society of London A 372, 20130259.
; Boyce et al., 2015Boyce, J.W., Treiman, A.H., Guan, Y., Ma, C., Eiler, J.M., Gross J., Greenwood, J.P., Stolper, E.M. (2015) The chlorine isotope fngerprint of the lunar magma ocean. Science Advances 1, e1500380.
; Wang and Jacobsen, 2016Wang, K., Jacobsen, S.B. (2016) Potassium isotopic evidence for a high-energy giant impact origin of the Moon. Nature 538, 487-490.
; Dhaliwal et al., 2018Dhaliwal, J.K., Day, J.M.D., Moynier, F. (2018) Volatile element loss during planetary magma ocean phases. Icarus 300, 249-260.
).An advantage of examining the MVE relative to more volatile compounds such as H2O is that they generally occur in sufficient quantity within rock-forming minerals and glasses that their isotopic composition can be precisely measured. Copper (TC50 = 1037 K) is a useful tracer of planetary volatile histories, being both moderately volatile and chalcophile during planetary processes (e.g., Moynier et al., 2017
Moynier, F., Vance, D., Fujii, T., Savage, P. (2017) The isotope geochemistry of zinc and copper. Reviews in Mineralogy and Geochemistry 82, 543-600.
). Copper isotopic fractionations (expressed as δ65Cu = ([65Cu/63Cu)sample/[65Cu/63Cu]SRM976 - 1) × 1000) of planetary materials do not match predicted kinetic isotopic fractionation factors, but are instead consistent with subdued fractionation factors approaching unity (Moynier et al., 2017Moynier, F., Vance, D., Fujii, T., Savage, P. (2017) The isotope geochemistry of zinc and copper. Reviews in Mineralogy and Geochemistry 82, 543-600.
). A problem in interpreting volatile element isotope fractionation has been that laboratory experiments are unable to reproduce large scale volatile processes, making empirical assessment of volatile element loss during planet formation challenging. This is particularly troubling since debate surrounds the extent of volatile loss experienced by planets and when this occurred (Paniello et al., 2012Paniello, R.C., Day, J.M.D., Moynier, F. (2012) Zinc isotopic evidence for the origin of the Moon. Nature 490, 376-379.
; O’Brien et al., 2014O’Brien, D.P., Walsh, K.J., Morbidelli, A., Raymond, S.N., Mandell, A.M. (2014) Water delivery and giant impacts in the ‘Grand Tack’ scenario. Icarus 239, 74-84.
; Wang and Jacobsen, 2016Wang, K., Jacobsen, S.B. (2016) Potassium isotopic evidence for a high-energy giant impact origin of the Moon. Nature 538, 487-490.
).To examine the behaviour of Cu during high temperature processes, and to compare it with two other MVE, we investigated melted glasses (trinitite) formed during nuclear detonation at the Trinity Test site, New Mexico, USA. During this event, local conditions exceeded 8430 K, leading to vapourisation of the arkosic desert sand substrate (Supplementary Information). Temperature estimates for the explosion are above those anticipated for typical basaltic eruption temperatures. The conditions of formation were likely to be reducing since the absolute logfO2 (bar) defined by the FMQ buffer is -6.3 at 1673 K, but is -1.8 at 2273 K. In contrast, air has logfO2 = -0.68 at both temperatures, resulting in reducing conditions relative to solid-solid buffers.
top
Samples and Methods
Trinitite samples collected with known distances from the detonation and that have previously been examined for Zn (Day et al., 2017
Day, J.M.D., Moynier, F., Meshik, A.P., Pradivtseva, O.V., Petit, D.R. (2017) Evaporative fractionation of zinc during the first nuclear detonation. Science Advances 3, p.e1602668.
) and K isotopes (Chen et al., 2019Chen, H., Meshik, A.P., Pravdivtseva, O.V., Day, J.M.D., Wang, K. (2019) Potassium isotope fractionation during the high-temperature evaporation determined from the Trinity nuclear test. Chemical Geology 522, 84-92.
) were studied. These samples include trinitite taken within 10 m of ground zero (IF), at 100 m (IG) and at 200 ± 50 m (T3). Sample T3 had the least amount of trinitite glass, and we use this sample to establish background Cu abundance and 65Cu/63Cu at the Trinity test site, as it has a similar composition to ‘unmelted’ sand (Bellucci et al., 2014Bellucci, J.J., Simonetti, A., Koeman, E.C., Wallace, C., Burns, P.C. (2014) A detailed investigation of post-nuclear detonation trinitite glass at high spatial resolution: delineating anthropogenic vs. natural components. Chemical Geology 365, 69-86.
). The 10 m sample, IF, formed at 1663 K and cooled within 17 minutes of emplacement, whereas the 100 m sample, IG, formed at 1593 K, cooled within five minutes of emplacement (Meshik et al., 2008Meshik, A., Pravdivtseva, O., Hohenberg, C. (2008) Fission xenon in trinities from the first nuclear test. APS April Meeting and HEDP/HEDLA Meeting, St. Louis, MO, 11 to 15 April 2008.
). Peripheral sample T3 formed at lower temperatures and higher rates of cooling.To assess Cu elemental or isotopic heterogeneity, a systematic procedure of crushing and leaching/etching was performed on trinitites (Supplementary Information). The final Cu cuts after chemistry, representing 99 ± 1 % of Cu in the sample, were analysed for isotopic composition using a Thermo Fischer Neptune Plus Multi-Collector Inductively-Coupled-Plasma Mass-Spectrometer (MC-ICP-MS) at the Institut de Physique du Globe de Paris following procedures in Sossi et al. (2015)
Sossi, P.A., Halverson, G.P., Nebel, O., Eggins, S.M. (2015) Combined separation of Cu, Fe and Zn from rock matrices and improved analytical protocols for stable isotope determination. Geostandards and Geoanalytical Research 39, 129-149.
, and detailed in the Supplementary Information. To correct for instrumental mass bias, isotope measurements were doped with Ni to correct for matrix effects and calculated using the standard sample bracketing protocol relative to the NIST SRM976 standard, whereby variations in Cu ratios are defined using the delta notation (δ65Cu).top
Copper Isotope and Abundance Data for Trinitite
The T3 trinitite, furthest from the centre of the nuclear detonation, has a typical BSE δ65Cu value and higher Cu content than the IF and IG samples. When plotted as Cu content versus δ65Cu (Figure 1), the IF and IG residues, etchates and leachates form negatively correlated trends, only disturbed by the IG HCl leach. Hydrochloric acid is effective at leaching sulfide or metal, and we posit that isotopically heavy metal particulate from close to the detonating ‘Gadget’ was incorporated into IF, explaining the IF HCl Cu composition as well as anomalously high Pb in this sample (Day et al., 2017
Day, J.M.D., Moynier, F., Meshik, A.P., Pradivtseva, O.V., Petit, D.R. (2017) Evaporative fractionation of zinc during the first nuclear detonation. Science Advances 3, p.e1602668.
). Exclusion of this sample leads to correlations for the residue, bulk, HF-HNO3 etch and H2O leach (y = -0.057x + 2.39; R2 = 0.95) matching that of the IF sample (y = -0.070x + 0.698; R2 = 0.99). In both cases, the isotopically lightest values occur in H2O, and the heaviest values occur in the residue.Lithium and Fe isotopes during leaching can be fractionated by >2 ‰ as a consequence of either diffusion driven isotopic fractionation (Li) or proton promoted dissolution (Fe) that are influenced by sample composition (Verney-Carron et al., 2011
Verney-Carron, A., Vigier, N., Millot, R. (2011) Experimental determination of the role of diffusion on Li isotope fractionation during basaltic glass weathering. Geochimica et Cosmochimica Acta 75, 3452-3468.
; Revels et al., 2015Revels, B.N., Zhang, R., Adkins, J.F., John, S.G. (2015) Fractionation of iron isotopes during leaching of natural particles by acidic and circumneutral leaches and development of an optimal leach for marine particulate iron isotopes. Geochimica et Cosmochimica Acta 166, 92-104.
). Increasing reagent strength and prolonged time of exposure (>2 hours) also leads to increasing isotopic fractionation. The Cu isotope data for trinitites cannot be explained by laboratory induced fractionation effects. For example, no laboratory induced isotopic fractionation of Cu was observed in lunar sample 66095 using the same methods (Day et al., 2019Day, J.M.D., Sossi, P.A., Shearer, C.K., Moynier, F. (2019) Volatile distributions in and on the Moon revealed by Cu and Fe isotopes in the ‘Rusty Rock’ 66095. Geochimica et Cosmochimica Acta 266, 131-143.
). Instead, the most extreme Cu isotope fractionation occurs in the mildest leaches and not in the more aggressive etches. The Cu, Zn and K data for trinitite therefore reflect sampling of distinct sub-reservoirs in samples.
Figure 1 Copper content versus δ65Cu in trinitite. Bulk silicate Earth (BSE) estimate from Savage et al. (2015)
Savage, P.S., Moynier, F., Chen, H., Shofner, G., Siebert, J., Badro, J., Puchtel, I.S. (2015) Copper isotope evidence for large-scale sulphide fractionation during Earth’s differentiation. Geochemical Perspectives Letters 1, 53-64.
.Negative trends in Cu content versus δ65Cu space (Figure 1) indicate that Cu is isotopically heavy in the silicate fraction of trinitite and is isotopically heaviest closest to the nuclear detonation centre. Conversely, H2O leaches appear to have sampled isotopically light Cu, perhaps preserved as soluble Cu salts, on the outer glass surfaces.
top
Isotopic Fractionation of Cu, Zn and K
There is a correlation of decreasing Cu content with proximity to the ‘Gadget’ (Fig. 2). A similar less well-constrained relationship has previously been observed for Zn and Pb (Day et al., 2017
Day, J.M.D., Moynier, F., Meshik, A.P., Pradivtseva, O.V., Petit, D.R. (2017) Evaporative fractionation of zinc during the first nuclear detonation. Science Advances 3, p.e1602668.
), but is not so evident in trinitite K contents (Chen et al., 2019Chen, H., Meshik, A.P., Pravdivtseva, O.V., Day, J.M.D., Wang, K. (2019) Potassium isotope fractionation during the high-temperature evaporation determined from the Trinity nuclear test. Chemical Geology 522, 84-92.
). The relationship between distance from the nuclear detonation and δ65Cu is less well-defined, and not as clear as δ66Zn, but is better defined than δ41K, where significant K isotope fractionation from the BSE value only occurs for IF trinitite. Therefore, the IF and IG trinitite samples are demonstrably isotopically heavier than either BSE or the T3 sample for Cu, as well as for Zn, and in the case of IF, for K (Table S-1) (Fig. 3). There is no evidence that there is sufficient isotopic or elemental heterogeneity in Earth materials, or the arkosic sand at the Trinity test site, for this variation to be natural. The only process explaining this relationship is high temperature evaporative fractionation.Evaporation of volatile elements at high temperatures is thought to be a function of element condensation temperatures under nebular conditions, or bond-bond energies (Albarède et al., 2015
Albarède, F., Albalat, E., Lee, C.T.A. (2015) An intrinsic volatility scale relevant to the Earth and Moon and the status of water in the Moon. Meteoritics and Planetary Science 50, 568-577.
). However, for vapourisation or condensation processes that take place under distinct thermodynamic conditions, these temperatures bear limited relevance. This is because the condensed phase is a silicate liquid melting and vapourising at 1 bar total pressure, compared to condensation of solid metal and silicate minerals at low pressures in the H2 atmosphere of the solar nebula (Norris and Wood, 2017Norris, C.A., Wood, B.J. (2017) Earth’s volatile contents established by melting and vaporization. Nature 549, 507-510.
; Sossi et al., 2019Sossi, P.A., Klemme, S., O'Neill, H.S.C., Berndt, J., Moynier, F. (2019) Evaporation of moderately volatile elements from silicate melts: experiments and theory. Geochimica et Cosmochimica Acta 260, 204-231.
). Trinitites allow empirical assessment of relative volatilities of MVE with mass differences of two. From relationships between δ65Cu and δ66Zn and δ65Cu and δ41K in Figure 3 it is possible to calculate the relative sensitivity of these isotope systems during high temperature evaporative fractionation. We obtain:


Figure 2 (a) δ65Cu and (b) Cu abundance in trinitite with distance from the ‘Gadget’ compared with Zn, Pb and K contents from the same samples (Day et al., 2017
Day, J.M.D., Moynier, F., Meshik, A.P., Pradivtseva, O.V., Petit, D.R. (2017) Evaporative fractionation of zinc during the first nuclear detonation. Science Advances 3, p.e1602668.
; Chen et al., 2019Chen, H., Meshik, A.P., Pravdivtseva, O.V., Day, J.M.D., Wang, K. (2019) Potassium isotope fractionation during the high-temperature evaporation determined from the Trinity nuclear test. Chemical Geology 522, 84-92.
). BSE is from Savage et al. (2015)Savage, P.S., Moynier, F., Chen, H., Shofner, G., Siebert, J., Badro, J., Puchtel, I.S. (2015) Copper isotope evidence for large-scale sulphide fractionation during Earth’s differentiation. Geochemical Perspectives Letters 1, 53-64.
.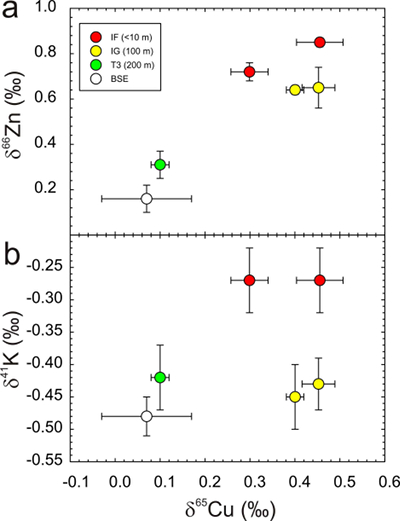
Figure 3 Copper versus (a) Zn and (b) K isotopes in trinitite. Zinc and K data for trinitites from Day et al. (2017)
Day, J.M.D., Moynier, F., Meshik, A.P., Pradivtseva, O.V., Petit, D.R. (2017) Evaporative fractionation of zinc during the first nuclear detonation. Science Advances 3, p.e1602668.
and Chen et al. (2019)Chen, H., Meshik, A.P., Pravdivtseva, O.V., Day, J.M.D., Wang, K. (2019) Potassium isotope fractionation during the high-temperature evaporation determined from the Trinity nuclear test. Chemical Geology 522, 84-92.
. BSE Cu, Zn and K values are from Moynier et al. (2017)Moynier, F., Vance, D., Fujii, T., Savage, P. (2017) The isotope geochemistry of zinc and copper. Reviews in Mineralogy and Geochemistry 82, 543-600.
and Wang and Jacobsen (2016)Wang, K., Jacobsen, S.B. (2016) Potassium isotopic evidence for a high-energy giant impact origin of the Moon. Nature 538, 487-490.
.Relative element volatilities during evaporation from trinitite can be compared with theoretical and experimental considerations of MVE evaporation from basaltic silicate melt. Both Cu and K are expected to exist as monatomic oxides in silicate melts (i.e. KO0.5 and CuO0.5) while their gas species, under nominally anhydrous conditions and high temperatures, are K0 (g) and Cu0 (g) (Lamoreaux and Hildenbrand, 1984
Lamoreaux, R.H., Hildenbrand, D.L. (1984) High temperature vaporization behavior of oxides. I. Alkali metal binary oxides. Journal of Physical and Chemical Reference Data 13, 151-173.
; Lamoreaux et al. 1987Lamoreaux, R.H., Hildenbrand, D.L., Brewer, L. (1987) High-temperature vaporization behavior of oxides II. Oxides of Be, Mg, Ca, Sr, Ba, B, Al, Ga, In, Tl, Si, Ge, Sn, Pb, Zn, Cd, and Hg. Journal of Physical and Chemical Reference Data 16, 419-443.
). Therefore, partial pressures during evaporation should increase proportionally to (fO2)-0.25. Although Zn is also present in the gas as Zn0, its stable melt oxide species is ZnO(l), conferring a dependence of (fO2)-0.5 on p(Zn) (Sossi et al., 2019Sossi, P.A., Klemme, S., O'Neill, H.S.C., Berndt, J., Moynier, F. (2019) Evaporation of moderately volatile elements from silicate melts: experiments and theory. Geochimica et Cosmochimica Acta 260, 204-231.
). Therefore, increasingly reducing conditions favour evaporation of Zn over Cu and K. Calculations of equilibrium partial pressures of gaseous elements over silicate melts requires knowledge of the activity coefficients of these elements in the condensed phase, assuming an ideal gas phase. The Cu activity coefficient in basaltic melts has been determined to be ≈10-20 at 1300 °C, extending down to 3.5 at 1650 °C (Holzheid and Lodders, 2001Holzheid, A, Lodders, K. (2001) Solubility of copper in silicate melts as function of oxygen and sulfur fugacities, temperature, and silicate composition. Geochimica et Cosmochimica Acta 65, 1933–1951.
; Wood and Wade, 2013Wood, B.J., Wade, J. (2013) Activities and volatilities of trace components in silicate melts: a novel use of metal–silicate partitioning data. Contributions to Mineralogy and Petrology 166, 911-921.
), whereas that of Zn is slightly less than unity (Sossi et al., 2019Sossi, P.A., Klemme, S., O'Neill, H.S.C., Berndt, J., Moynier, F. (2019) Evaporation of moderately volatile elements from silicate melts: experiments and theory. Geochimica et Cosmochimica Acta 260, 204-231.
). By contrast, activity coefficients of alkali oxides in silicate melts are much less than unity (see discussion in Sossi and Fegley, 2018Sossi, P.A., Fegley, B. (2018) Thermodynamics of element volatility and its application to planetary processes. Reviews in Mineralogy and Geochemistry 84, 393-459.
). This key property stabilises them in the silicate melt, resulting in decreased volatilities compared to the nebular condensation temperature.For KO0.5, appropriate activity coefficients are ≈2 × 10-4 (Hastie and Bonnell, 1985
Hastie, J.W., Bonnell, D.W. (1985) A predictive phase equilibrium model for multicomponent oxide mixtures part II. Oxides of Na–K–Ca–Mg–Al–Si. High Temperature Science 19, 275–306.
; Sossi et al., 2019Sossi, P.A., Klemme, S., O'Neill, H.S.C., Berndt, J., Moynier, F. (2019) Evaporation of moderately volatile elements from silicate melts: experiments and theory. Geochimica et Cosmochimica Acta 260, 204-231.
). Insofar as these values are applicable to trinitite melts, the equilibrium partial pressures of these species can be calculated in air (log fO2 = -0.68). Relative to their mole fraction in the condensed phase, their partial pressures at 1600 K and 1 bar total pressure are 1.06 × 10-5 bar for K, 1.58 × 10-4 bar for Zn and 8.84 × 10-5 bar for Cu, resulting in an expected order of volatility Zn > Cu >> K, in agreement with observations. If the amount of an element (E) vapourised is proportional to its partial pressure (p) divided by its mole fraction (X), then: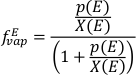



Ni, P., Zhang, Y. (2017) Cu diffusion in a basaltic melt. American Mineralogist 101, 1474-1482.
), this will promote loss of Cu relative to K and Zn, enhancing isotopic fractionation (Moynier et al. 2010Moynier, F., Koeberl, C., Beck, P., Jourdan, F., Telouk, P. (2010) Isotopic fractionation of Cu in tektites. Geochimica et Cosmochimica Acta 74, 799-807.
).top
Implications for the Formation of Tektites and the Moon
Volatile behaviour in trinitite glasses enables assessment of how high temperature evaporation processes may have acted on other planetary materials. In the first instance, we consider tektites. Tektites are glassy materials of various shapes and sizes that are considered to have formed from meteoroid-induced atmospheric air blasts that melted soils and upper crustal materials in certain regions during discrete events. Tektites show extreme δ66Zn and δ65Cu variations, being enriched in the heavier isotopes due to evaporative fractionation (Moynier et al., 2009
Moynier, F., Beck, P., Jourdan, F., Yin, Q.-Z., Reimold, U., Koeberl, C. (2009) Isotopic fractionation of zinc in tektites. Earth and Planetary Science Letters 277, 482-489.
, 2010Moynier, F., Koeberl, C., Beck, P., Jourdan, F., Telouk, P. (2010) Isotopic fractionation of Cu in tektites. Geochimica et Cosmochimica Acta 74, 799-807.
). Potassium isotopes are less extremely fractionated than for Cu or Zn isotopes, spanning the range of δ41K observed in trinitites (e.g., Jiang et al., 2019Jiang, Y., Chen, H., Fegley, B. Jr., Lodders, K., Hsu, W., Jacobsen, S.B., Wang, K. (2019) Implications of K, Cu and Zn isotopes for the formation of tektites. Geochimica et Cosmochimica Acta 259, 170-187.
). The results from trinitite are consistent with fractionation of Cu and Zn isotopes occurring in the tektites that is as great or greater than for the IF (10 m) trinitite sample. Limited K isotope fractionation is consistent with its low activity coefficient in silicate melts compared to Cu and Zn, hindering evaporation (e.g., Hastie and Bonnell, 1985Hastie, J.W., Bonnell, D.W. (1985) A predictive phase equilibrium model for multicomponent oxide mixtures part II. Oxides of Na–K–Ca–Mg–Al–Si. High Temperature Science 19, 275–306.
). The variation in δ66Zn in tektites is large, whereas the variation in δ65Cu is well described by fractionation factors (α) of between 0.999 and 0.9998, similar to those required to explain Zn, Cu and K isotope fractionation at the Trinity nuclear test site (Fig. 4).The other example we consider is MVE depletion in lunar samples. Shown in Figure 4 are Zn isotope data for mare basalts, and Cu and K isotope data for a variety of lunar samples, including mare basalts and some soils. The lunar samples are systematically enriched in the heavier isotopes of Zn, Cu and K relative to BSE. These isotope systems can all be explained by fractionation factors (α) of between 0.999 and 0.9998 for 66Zn/64Zn, 65Cu/63Cu and 41K/39K, corresponding to the empirical fractionation factors derived from trinitite, and far smaller than for evaporation into a vacuum. Lunar samples span a range in isotopic composition and contents of Zn, Cu and K, suggesting inhomogeneity in the Moon and MVE fractionation at high temperatures during magmatic evolution. Such variability is therefore inconsistent with MVE evaporative fractionation solely in a solid-vapour disk forming the Earth and Moon (Paniello et al., 2012
Paniello, R.C., Day, J.M.D., Moynier, F. (2012) Zinc isotopic evidence for the origin of the Moon. Nature 490, 376-379.
; Wang and Jacobsen, 2016Wang, K., Jacobsen, S.B. (2016) Potassium isotopic evidence for a high-energy giant impact origin of the Moon. Nature 538, 487-490.
), and also requires volatile loss during later lunar evolution, such as during a magma ocean phase (e.g., Day and Moynier, 2014Day, J.M.D., Moynier, F. (2014) Evaporative fractionation of volatile stable isotopes and their bearing on the origin of the Moon. Philosophical Transactions of the Royal Society of London A 372, 20130259.
; Boyce et al., 2015Boyce, J.W., Treiman, A.H., Guan, Y., Ma, C., Eiler, J.M., Gross J., Greenwood, J.P., Stolper, E.M. (2015) The chlorine isotope fngerprint of the lunar magma ocean. Science Advances 1, e1500380.
; Day et al., 2020Day, J.M.D., van Kooten, E.M.M.E., Hofmann, B.A., Moynier, F. (2020) Mare basalt meteorites, magnesian-suite rocks and KREEP reveal loss of zinc during and after lunar formation. Earth and Planetary Science Letters 351, 115998.
) where partial pressures of degassing species may lead to accumulation at the surface, reducing the degree of isotopic fractionation to near-equilibrium values.
Figure 4 Rayleigh distillation curves versus (a) Cu, (b) Zn and (c) K contents and isotopic compositions for trinitite glass, tektites and lunar samples. Curves are shown for different alpha fractionation (α) factors (1, 0.9998, 0.999, 0.998) established from trinitite, with 70 µg g-1 Cu, 30 µg g-1 Zn and 40000 µg g-1 K for estimated BSE isotopic compositions. Data are from this study, Day et al. (2017)
Day, J.M.D., Moynier, F., Meshik, A.P., Pradivtseva, O.V., Petit, D.R. (2017) Evaporative fractionation of zinc during the first nuclear detonation. Science Advances 3, p.e1602668.
, and Chen et al. (2019)Chen, H., Meshik, A.P., Pravdivtseva, O.V., Day, J.M.D., Wang, K. (2019) Potassium isotope fractionation during the high-temperature evaporation determined from the Trinity nuclear test. Chemical Geology 522, 84-92.
for trinitite. Tektite data are from Moynier et al. (2009Moynier, F., Beck, P., Jourdan, F., Yin, Q.-Z., Reimold, U., Koeberl, C. (2009) Isotopic fractionation of zinc in tektites. Earth and Planetary Science Letters 277, 482-489.
, 2010Moynier, F., Koeberl, C., Beck, P., Jourdan, F., Telouk, P. (2010) Isotopic fractionation of Cu in tektites. Geochimica et Cosmochimica Acta 74, 799-807.
), and Jiang et al. (2019)Jiang, Y., Chen, H., Fegley, B. Jr., Lodders, K., Hsu, W., Jacobsen, S.B., Wang, K. (2019) Implications of K, Cu and Zn isotopes for the formation of tektites. Geochimica et Cosmochimica Acta 259, 170-187.
, and lunar basalts data are from Paniello et al. (2012)Paniello, R.C., Day, J.M.D., Moynier, F. (2012) Zinc isotopic evidence for the origin of the Moon. Nature 490, 376-379.
, Kato et al. (2015)Kato, C., Moynier, F., Valdes, M.C., Dhaliwal, J.K., Day, J.M.D. (2015) Extensive volatile loss during formation and differentiation of the Moon. Nature Communications 6, 7617, doi: 10.1038/ncomms8617.
, and Wang and Jacobsen (2016)Wang, K., Jacobsen, S.B. (2016) Potassium isotopic evidence for a high-energy giant impact origin of the Moon. Nature 538, 487-490.
and references therein.top
Acknowledgements
We thank C.-T. Lee and an anonymous reviewer for their thoughtful comments. This work was supported by the NASA Emerging Worlds program (NNX15AL74G; 80NSSC19K0932) and an IPGP Visiting Professor position to JD. We thank the ERC under the European Community’s H2020 framework program/ERC grant agreement # 637503 (Pristine) and for the UnivEarthS Labex program (no. ANR-10-LABX-0023 and ANR-11-IDEX-0005-02). Parts of this work were supported by IPGP multidisciplinary program PARI, and by Region île-de-France SESAME Grant (no. 12015908). The authors declare no conflicts of interest. All data referred to in this article can be found in the tables or cited references.
Editor: Cin-Ty Lee
top
Author Contributions
JD designed the research and wrote the article; JD, PS and FM collected the data; AM, OP and DP provided samples. All authors provided comments and suggestions on the article.
top
References
Albarède, F., Albalat, E., Lee, C.T.A. (2015) An intrinsic volatility scale relevant to the Earth and Moon and the status of water in the Moon. Meteoritics and Planetary Science 50, 568-577.

The MVE have 50 % nebular condensation temperatures (TC50) at solar nebula conditions (10-4 bar) of between 1134 K and 665 K (Lodders, 2003), and have low element-element bond energies (Albarède et al., 2015).
View in article
Evaporation of volatile elements at high temperatures is thought to be a function of element condensation temperatures under nebular conditions, or bond-bond energies (Albarède et al., 2015).
View in article
Bellucci, J.J., Simonetti, A., Koeman, E.C., Wallace, C., Burns, P.C. (2014) A detailed investigation of post-nuclear detonation trinitite glass at high spatial resolution: delineating anthropogenic vs. natural components. Chemical Geology 365, 69-86.

Sample T3 had the least amount of trinitite glass, and we use this sample to establish background Cu abundance and 65Cu/63Cu at the Trinity test site, as it has a similar composition to ‘unmelted’ sand (Bellucci et al., 2014).
View in article
Boyce, J.W., Treiman, A.H., Guan, Y., Ma, C., Eiler, J.M., Gross J., Greenwood, J.P., Stolper, E.M. (2015) The chlorine isotope fngerprint of the lunar magma ocean. Science Advances 1, e1500380.

Stable isotope MVE compositions have been utilised to examine fractionation effects induced by evaporation or condensation, and to assess stable isotope differences between Earth and the Moon (e.g., Paniello et al., 2012; Day and Moynier, 2014; Boyce et al., 2015; Wang and Jacobsen, 2016; Dhaliwal et al., 2018).
View in article
Such variability is therefore inconsistent with MVE evaporative fractionation solely in a solid-vapour disk forming the Earth and Moon (Paniello et al., 2012; Wang and Jacobsen, 2016), and also requires volatile loss during later lunar evolution, such as during a magma ocean phase (e.g., Day and Moynier, 2014; Boyce et al., 2015; Day et al., 2020) where partial pressures of degassing species may lead to accumulation at the surface, reducing the degree of isotopic fractionation to near-equilibrium values.
View in article
Chen, H., Meshik, A.P., Pravdivtseva, O.V., Day, J.M.D., Wang, K. (2019) Potassium isotope fractionation during the high-temperature evaporation determined from the Trinity nuclear test. Chemical Geology 522, 84-92.

Trinitite samples collected with known distances from the detonation and that have previously been examined for Zn (Day et al., 2017) and K isotopes (Chen et al., 2019) were studied.
View in article
A similar less well-constrained relationship has previously been observed for Zn and Pb (Day et al., 2017), but is not so evident in trinitite K contents (Chen et al., 2019).
View in article
Figure 2 (a) δ65Cu and (b) Cu abundance in trinitite with distance from the ‘Gadget’ compared with Zn, Pb and K contents from the same samples (Day et al., 2017; Chen et al., 2019)
View in article
Figure 3 [...] Zinc and K data for trinitites from Day et al. (2017) and Chen et al. (2019).
View in article
Figure 4 [...] Data are from this study, Day et al. (2017), and Chen et al. (2019) for trinitite.
View in article
Day, J.M.D., Moynier, F. (2014) Evaporative fractionation of volatile stable isotopes and their bearing on the origin of the Moon. Philosophical Transactions of the Royal Society of London A 372, 20130259.

Stable isotope MVE compositions have been utilised to examine fractionation effects induced by evaporation or condensation, and to assess stable isotope differences between Earth and the Moon (e.g., Paniello et al., 2012; Day and Moynier, 2014; Boyce et al., 2015; Wang and Jacobsen, 2016; Dhaliwal et al., 2018).
View in article
Such variability is therefore inconsistent with MVE evaporative fractionation solely in a solid-vapour disk forming the Earth and Moon (Paniello et al., 2012; Wang and Jacobsen, 2016), and also requires volatile loss during later lunar evolution, such as during a magma ocean phase (e.g., Day and Moynier, 2014; Boyce et al., 2015; Day et al., 2020) where partial pressures of degassing species may lead to accumulation at the surface, reducing the degree of isotopic fractionation to near-equilibrium values.
View in article
Day, J.M.D., Moynier, F., Meshik, A.P., Pradivtseva, O.V., Petit, D.R. (2017) Evaporative fractionation of zinc during the first nuclear detonation. Science Advances 3, p.e1602668.

Trinitite samples collected with known distances from the detonation and that have previously been examined for Zn (Day et al., 2017) and K isotopes (Chen et al., 2019) were studied.
View in article
Hydrochloric acid is effective at leaching sulfide or metal, and we posit that isotopically heavy metal particulate from close to the detonating ‘Gadget’ was incorporated into IF, explaining the IF HCl Cu composition as well as anomalously high Pb in this sample (Day et al., 2017).
View in article
A similar less well-constrained relationship has previously been observed for Zn and Pb (Day et al., 2017), but is not so evident in trinitite K contents (Chen et al., 2019).
View in article
Figure 2 (a) δ65Cu and (b) Cu abundance in trinitite with distance from the ‘Gadget’ compared with Zn, Pb and K contents from the same samples (Day et al., 2017; Chen et al., 2019)
View in article
Figure 3 [...] Zinc and K data for trinitites from Day et al. (2017) and Chen et al. (2019).
View in article
Figure 4 [...] Data are from this study, Day et al. (2017), and Chen et al. (2019) for trinitite.
View in article
Day, J.M.D., Sossi, P.A., Shearer, C.K., Moynier, F. (2019) Volatile distributions in and on the Moon revealed by Cu and Fe isotopes in the ‘Rusty Rock’ 66095. Geochimica et Cosmochimica Acta 266, 131-143.

For example, no laboratory induced isotopic fractionation of Cu was observed in lunar sample 66095 using the same methods (Day et al., 2019).
View in article
Day, J.M.D., van Kooten, E.M.M.E., Hofmann, B.A., Moynier, F. (2020) Mare basalt meteorites, magnesian-suite rocks and KREEP reveal loss of zinc during and after lunar formation. Earth and Planetary Science Letters 351, 115998.

Such variability is therefore inconsistent with MVE evaporative fractionation solely in a solid-vapour disk forming the Earth and Moon (Paniello et al., 2012; Wang and Jacobsen, 2016), and also requires volatile loss during later lunar evolution, such as during a magma ocean phase (e.g., Day and Moynier, 2014; Boyce et al., 2015; Day et al., 2020) where partial pressures of degassing species may lead to accumulation at the surface, reducing the degree of isotopic fractionation to near-equilibrium values.
View in article
Dhaliwal, J.K., Day, J.M.D., Moynier, F. (2018) Volatile element loss during planetary magma ocean phases. Icarus 300, 249-260.

Stable isotope MVE compositions have been utilised to examine fractionation effects induced by evaporation or condensation, and to assess stable isotope differences between Earth and the Moon (e.g., Paniello et al., 2012; Day and Moynier, 2014; Boyce et al., 2015; Wang and Jacobsen, 2016; Dhaliwal et al., 2018).
View in article
Hastie, J.W., Bonnell, D.W. (1985) A predictive phase equilibrium model for multicomponent oxide mixtures part II. Oxides of Na–K–Ca–Mg–Al–Si. High Temperature Science 19, 275–306.

For KO0.5, appropriate activity coefficients are ≈2 × 10-4 (Hastie and Bonnell, 1985; Sossi et al., 2019).
View in article
Limited K isotope fractionation is consistent with its low activity coefficient in silicate melts compared to Cu and Zn, hindering evaporation (e.g., Hastie and Bonnell, 1985).
View in article
Holzheid, A, Lodders, K. (2001) Solubility of copper in silicate melts as function of oxygen and sulfur fugacities, temperature, and silicate composition. Geochimica et Cosmochimica Acta 65, 1933–1951.

The Cu activity coefficient in basaltic melts has been determined to be ≈10-20 at 1300 °C, extending down to 3.5 at 1650 °C (Holzheid and Lodders, 2001; Wood and Wade, 2013), whereas that of Zn is slightly less than unity (Sossi et al., 2019).
View in article
Jiang, Y., Chen, H., Fegley, B. Jr., Lodders, K., Hsu, W., Jacobsen, S.B., Wang, K. (2019) Implications of K, Cu and Zn isotopes for the formation of tektites. Geochimica et Cosmochimica Acta 259, 170-187.

Potassium isotopes are less extremely fractionated than for Cu or Zn isotopes, spanning the range of δ41K observed in trinitites (e.g., Jiang et al., 2019).
View in article
Figure 4 [...] Tektite data are from Moynier et al. (2009, 2010), and Jiang et al. (2019), and lunar basalts data are from Paniello et al. (2012), Kato et al. (2015), and Wang and Jacobsen (2016) and references therein.
View in article
Kato, C., Moynier, F., Valdes, M.C., Dhaliwal, J.K., Day, J.M.D. (2015) Extensive volatile loss during formation and differentiation of the Moon. Nature Communications 6, 7617, doi: 10.1038/ncomms8617.

Figure 4 [...] Tektite data are from Moynier et al. (2009, 2010), and Jiang et al. (2019), and lunar basalts data are from Paniello et al. (2012), Kato et al. (2015), and Wang and Jacobsen (2016) and references therein.
View in article
Lamoreaux, R.H., Hildenbrand, D.L. (1984) High temperature vaporization behavior of oxides. I. Alkali metal binary oxides. Journal of Physical and Chemical Reference Data 13, 151-173.

Relative element volatilities during evaporation from trinitite can be compared with theoretical and experimental considerations of MVE evaporation from basaltic silicate melt. Both Cu and K are expected to exist as monatomic oxides in silicate melts (i.e. KO0.5 and CuO0.5) while their gas species, under nominally anhydrous conditions and high temperatures, are K0 (g) and Cu0 (g) (Lamoreaux and Hildenbrand, 1984; Lamoreaux et al. 1987).
View in article
Lamoreaux, R.H., Hildenbrand, D.L., Brewer, L. (1987) High-temperature vaporization behavior of oxides II. Oxides of Be, Mg, Ca, Sr, Ba, B, Al, Ga, In, Tl, Si, Ge, Sn, Pb, Zn, Cd, and Hg. Journal of Physical and Chemical Reference Data 16, 419-443.

Relative element volatilities during evaporation from trinitite can be compared with theoretical and experimental considerations of MVE evaporation from basaltic silicate melt. Both Cu and K are expected to exist as monatomic oxides in silicate melts (i.e. KO0.5 and CuO0.5) while their gas species, under nominally anhydrous conditions and high temperatures, are K0 (g) and Cu0 (g) (Lamoreaux and Hildenbrand, 1984; Lamoreaux et al. 1987).
View in article
Lodders, K. (2003) Solar system abundances and condensation temperatures of the elements. The Astrophysical Journal 591, 1220.

The MVE have 50 % nebular condensation temperatures (TC50) at solar nebula conditions (10-4 bar) of between 1134 K and 665 K (Lodders, 2003), and have low element-element bond energies (Albarède et al., 2015).
View in article
Meshik, A., Pravdivtseva, O., Hohenberg, C. (2008) Fission xenon in trinities from the first nuclear test. APS April Meeting and HEDP/HEDLA Meeting, St. Louis, MO, 11 to 15 April 2008.

The 10 m sample, IF, formed at 1663 K and cooled within 17 minutes of emplacement, whereas the 100 m sample, IG, formed at 1593 K, cooled within five minutes of emplacement (Meshik et al., 2008).
View in article
Moynier, F., Beck, P., Jourdan, F., Yin, Q.-Z., Reimold, U., Koeberl, C. (2009) Isotopic fractionation of zinc in tektites. Earth and Planetary Science Letters 277, 482-489.

Tektites show extreme δ66Zn and δ65Cu variations, being enriched in the heavier isotopes due to evaporative fractionation (Moynier et al., 2009, 2010).
View in article
Figure 4 [...] Tektite data are from Moynier et al. (2009, 2010), and Jiang et al. (2019), and lunar basalts data are from Paniello et al. (2012), Kato et al. (2015), and Wang and Jacobsen (2016) and references therein.
View in article
Moynier, F., Koeberl, C., Beck, P., Jourdan, F., Telouk, P. (2010) Isotopic fractionation of Cu in tektites. Geochimica et Cosmochimica Acta 74, 799-807.

As Cu is the fastest diffusing element of the trio (Zn being the slowest; Ni and Zhang, 2017), this will promote loss of Cu relative to K and Zn, enhancing isotopic fractionation (Moynier et al. 2010).
View in article
Tektites show extreme δ66Zn and δ65Cu variations, being enriched in the heavier isotopes due to evaporative fractionation (Moynier et al., 2009, 2010).
View in article
Figure 4 [...] Tektite data are from Moynier et al. (2009, 2010), and Jiang et al. (2019), and lunar basalts data are from Paniello et al. (2012), Kato et al. (2015), and Wang and Jacobsen (2016) and references therein.
View in article
Moynier, F., Vance, D., Fujii, T., Savage, P. (2017) The isotope geochemistry of zinc and copper. Reviews in Mineralogy and Geochemistry 82, 543-600.

Copper (TC50 = 1037 K) is a useful tracer of planetary volatile histories, being both moderately volatile and chalcophile during planetary processes (e.g., Moynier et al., 2017).
View in article
Copper isotopic fractionations (expressed as δ65Cu = ([65Cu/63Cu)sample/[65Cu/63Cu]SRM976 - 1) × 1000) of planetary materials do not match predicted kinetic isotopic fractionation factors, but are instead consistent with subdued fractionation factors approaching unity (Moynier et al., 2017).
View in article
Figure 3 [...] BSE Cu, Zn and K values are from Moynier et al. (2017) and Wang and Jacobsen (2016).
View in article
Ni, P., Zhang, Y. (2017) Cu diffusion in a basaltic melt. American Mineralogist 101, 1474-1482.

As Cu is the fastest diffusing element of the trio (Zn being the slowest; Ni and Zhang, 2017), this will promote loss of Cu relative to K and Zn, enhancing isotopic fractionation (Moynier et al. 2010).
View in article
Norris, C.A., Wood, B.J. (2017) Earth’s volatile contents established by melting and vaporization. Nature 549, 507-510.

This is because the condensed phase is a silicate liquid melting and vapourising at 1 bar total pressure, compared to condensation of solid metal and silicate minerals at low pressures in the H2 atmosphere of the solar nebula (Norris and Wood, 2017; Sossi et al., 2019).
View in article
O’Brien, D.P., Walsh, K.J., Morbidelli, A., Raymond, S.N., Mandell, A.M. (2014) Water delivery and giant impacts in the ‘Grand Tack’ scenario. Icarus 239, 74-84.

This is particularly troubling since debate surrounds the extent of volatile loss experienced by planets and when this occurred (Paniello et al., 2012; O’Brien et al., 2014; Wang and Jacobsen, 2016).
View in article
Paniello, R.C., Day, J.M.D., Moynier, F. (2012) Zinc isotopic evidence for the origin of the Moon. Nature 490, 376-379.

Stable isotope MVE compositions have been utilised to examine fractionation effects induced by evaporation or condensation, and to assess stable isotope differences between Earth and the Moon (e.g., Paniello et al., 2012; Day and Moynier, 2014; Boyce et al., 2015; Wang and Jacobsen, 2016; Dhaliwal et al., 2018).
View in article
This is particularly troubling since debate surrounds the extent of volatile loss experienced by planets and when this occurred (Paniello et al., 2012; O’Brien et al., 2014; Wang and Jacobsen, 2016).
View in article
Such variability is therefore inconsistent with MVE evaporative fractionation solely in a solid-vapour disk forming the Earth and Moon (Paniello et al., 2012; Wang and Jacobsen, 2016), and also requires volatile loss during later lunar evolution, such as during a magma ocean phase (e.g., Day and Moynier, 2014; Boyce et al., 2015; Day et al., 2020) where partial pressures of degassing species may lead to accumulation at the surface, reducing the degree of isotopic fractionation to near-equilibrium values.
View in article
Figure 4 [...] Tektite data are from Moynier et al. (2009, 2010), and Jiang et al. (2019), and lunar basalts data are from Paniello et al. (2012), Kato et al. (2015), and Wang and Jacobsen (2016) and references therein.
View in article
Revels, B.N., Zhang, R., Adkins, J.F., John, S.G. (2015) Fractionation of iron isotopes during leaching of natural particles by acidic and circumneutral leaches and development of an optimal leach for marine particulate iron isotopes. Geochimica et Cosmochimica Acta 166, 92-104.

Lithium and Fe isotopes during leaching can be fractionated by >2 ‰ as a consequence of either diffusion driven isotopic fractionation (Li) or proton promoted dissolution (Fe) that are influenced by sample composition (Verney-Carron et al., 2011; Revels et al., 2015).
View in article
Savage, P.S., Moynier, F., Chen, H., Shofner, G., Siebert, J., Badro, J., Puchtel, I.S. (2015) Copper isotope evidence for large-scale sulphide fractionation during Earth’s differentiation. Geochemical Perspectives Letters 1, 53-64.

Figure 1 [...] Bulk silicate Earth (BSE) estimate from Savage et al. (2015).
View in article
Figure 2 [...] BSE is from Savage et al. (2015).
View in article
Sossi, P.A., Fegley, B. (2018) Thermodynamics of element volatility and its application to planetary processes. Reviews in Mineralogy and Geochemistry 84, 393-459.

By contrast, activity coefficients of alkali oxides in silicate melts are much less than unity (see discussion in Sossi and Fegley, 2018).
View in article
Sossi, P.A., Halverson, G.P., Nebel, O., Eggins, S.M. (2015) Combined separation of Cu, Fe and Zn from rock matrices and improved analytical protocols for stable isotope determination. Geostandards and Geoanalytical Research 39, 129-149.

The final Cu cuts after chemistry, representing 99 ± 1 % of Cu in the sample, were analysed for isotopic composition using a Thermo Fischer Neptune Plus Multi-Collector Inductively-Coupled-Plasma Mass-Spectrometer (MC-ICP-MS) at the Institut de Physique du Globe de Paris following procedures in Sossi et al. (2015), and detailed in the Supplementary Information.
View in article
Sossi, P.A., Klemme, S., O'Neill, H.S.C., Berndt, J., Moynier, F. (2019) Evaporation of moderately volatile elements from silicate melts: experiments and theory. Geochimica et Cosmochimica Acta 260, 204-231.

This is because the condensed phase is a silicate liquid melting and vapourising at 1 bar total pressure, compared to condensation of solid metal and silicate minerals at low pressures in the H2 atmosphere of the solar nebula (Norris and Wood, 2017; Sossi et al., 2019).
View in article
Although Zn is also present in the gas as Zn0, its stable melt oxide species is ZnO(l), conferring a dependence of (fO2)-0.5 on p(Zn) (Sossi et al., 2019).
View in article
The Cu activity coefficient in basaltic melts has been determined to be ≈10-20 at 1300 °C, extending down to 3.5 at 1650 °C (Holzheid and Lodders, 2001; Wood and Wade, 2013), whereas that of Zn is slightly less than unity (Sossi et al., 2019).
View in article
For KO0.5, appropriate activity coefficients are ≈2 × 10-4 (Hastie and Bonnell, 1985; Sossi et al., 2019).
View in article
Verney-Carron, A., Vigier, N., Millot, R. (2011) Experimental determination of the role of diffusion on Li isotope fractionation during basaltic glass weathering. Geochimica et Cosmochimica Acta 75, 3452-3468.

Lithium and Fe isotopes during leaching can be fractionated by >2 ‰ as a consequence of either diffusion driven isotopic fractionation (Li) or proton promoted dissolution (Fe) that are influenced by sample composition (Verney-Carron et al., 2011; Revels et al., 2015).
View in article
Wang, K., Jacobsen, S.B. (2016) Potassium isotopic evidence for a high-energy giant impact origin of the Moon. Nature 538, 487-490.

Stable isotope MVE compositions have been utilised to examine fractionation effects induced by evaporation or condensation, and to assess stable isotope differences between Earth and the Moon (e.g., Paniello et al., 2012; Day and Moynier, 2014; Boyce et al., 2015; Wang and Jacobsen, 2016; Dhaliwal et al., 2018).
View in article
This is particularly troubling since debate surrounds the extent of volatile loss experienced by planets and when this occurred (Paniello et al., 2012; O’Brien et al., 2014; Wang and Jacobsen, 2016).
View in article
Figure 3 [...] BSE Cu, Zn and K values are from Moynier et al. (2017) and Wang and Jacobsen (2016).
View in article
Such variability is therefore inconsistent with MVE evaporative fractionation solely in a solid-vapour disk forming the Earth and Moon (Paniello et al., 2012; Wang and Jacobsen, 2016), and also requires volatile loss during later lunar evolution, such as during a magma ocean phase (e.g., Day and Moynier, 2014; Boyce et al., 2015; Day et al., 2020) where partial pressures of degassing species may lead to accumulation at the surface, reducing the degree of isotopic fractionation to near-equilibrium values.
View in article
Figure 4 [...] Tektite data are from Moynier et al. (2009, 2010), and Jiang et al. (2019), and lunar basalts data are from Paniello et al. (2012), Kato et al. (2015), and Wang and Jacobsen (2016) and references therein.
View in article
Wood, B.J., Wade, J. (2013) Activities and volatilities of trace components in silicate melts: a novel use of metal–silicate partitioning data. Contributions to Mineralogy and Petrology 166, 911-921.

The Cu activity coefficient in basaltic melts has been determined to be ≈10-20 at 1300 °C, extending down to 3.5 at 1650 °C (Holzheid and Lodders, 2001; Wood and Wade, 2013), whereas that of Zn is slightly less than unity (Sossi et al., 2019).
View in article
top
Supplementary Information
The Supplementary Information includes:
- The Trinity Test Site
- Figure S-1
- Detailed Methods
- Detailed Results
- Table S-1
- Supplementary Information References
Download the Supplementary Information (PDF).
Figures and Tables

Figure 1 Copper content versus δ65Cu in trinitite. Bulk silicate Earth (BSE) estimate from Savage et al. (2015)
Savage, P.S., Moynier, F., Chen, H., Shofner, G., Siebert, J., Badro, J., Puchtel, I.S. (2015) Copper isotope evidence for large-scale sulphide fractionation during Earth’s differentiation. Geochemical Perspectives Letters 1, 53-64.
.
Figure 2 (a) δ65Cu and (b) Cu abundance in trinitite with distance from the ‘Gadget’ compared with Zn, Pb and K contents from the same samples (Day et al., 2017
Day, J.M.D., Moynier, F., Meshik, A.P., Pradivtseva, O.V., Petit, D.R. (2017) Evaporative fractionation of zinc during the first nuclear detonation. Science Advances 3, p.e1602668.
; Chen et al., 2019Chen, H., Meshik, A.P., Pravdivtseva, O.V., Day, J.M.D., Wang, K. (2019) Potassium isotope fractionation during the high-temperature evaporation determined from the Trinity nuclear test. Chemical Geology 522, 84-92.
). BSE is from Savage et al. (2015)Savage, P.S., Moynier, F., Chen, H., Shofner, G., Siebert, J., Badro, J., Puchtel, I.S. (2015) Copper isotope evidence for large-scale sulphide fractionation during Earth’s differentiation. Geochemical Perspectives Letters 1, 53-64.
.
Figure 3 Copper versus (a) Zn and (b) K isotopes in trinitite. Zinc and K data for trinitites from Day et al. (2017)
Day, J.M.D., Moynier, F., Meshik, A.P., Pradivtseva, O.V., Petit, D.R. (2017) Evaporative fractionation of zinc during the first nuclear detonation. Science Advances 3, p.e1602668.
and Chen et al. (2019)Chen, H., Meshik, A.P., Pravdivtseva, O.V., Day, J.M.D., Wang, K. (2019) Potassium isotope fractionation during the high-temperature evaporation determined from the Trinity nuclear test. Chemical Geology 522, 84-92.
. BSE Cu, Zn and K values are from Moynier et al. (2017)Moynier, F., Vance, D., Fujii, T., Savage, P. (2017) The isotope geochemistry of zinc and copper. Reviews in Mineralogy and Geochemistry 82, 543-600.
and Wang and Jacobsen (2016)Wang, K., Jacobsen, S.B. (2016) Potassium isotopic evidence for a high-energy giant impact origin of the Moon. Nature 538, 487-490.
.
Figure 4 Rayleigh distillation curves versus (a) Cu, (b) Zn and (c) K contents and isotopic compositions for trinitite glass, tektites and lunar samples. Curves are shown for different alpha fractionation (α) factors (1, 0.9998, 0.999, 0.998) established from trinitite, with 70 µg g-1 Cu, 30 µg g-1 Zn and 40000 µg g-1 K for estimated BSE isotopic compositions. Data are from this study, Day et al. (2017)
Day, J.M.D., Moynier, F., Meshik, A.P., Pradivtseva, O.V., Petit, D.R. (2017) Evaporative fractionation of zinc during the first nuclear detonation. Science Advances 3, p.e1602668.
, and Chen et al. (2019)Chen, H., Meshik, A.P., Pravdivtseva, O.V., Day, J.M.D., Wang, K. (2019) Potassium isotope fractionation during the high-temperature evaporation determined from the Trinity nuclear test. Chemical Geology 522, 84-92.
for trinitite. Tektite data are from Moynier et al. (2009Moynier, F., Beck, P., Jourdan, F., Yin, Q.-Z., Reimold, U., Koeberl, C. (2009) Isotopic fractionation of zinc in tektites. Earth and Planetary Science Letters 277, 482-489.
, 2010Moynier, F., Koeberl, C., Beck, P., Jourdan, F., Telouk, P. (2010) Isotopic fractionation of Cu in tektites. Geochimica et Cosmochimica Acta 74, 799-807.
), and Jiang et al. (2019)Jiang, Y., Chen, H., Fegley, B. Jr., Lodders, K., Hsu, W., Jacobsen, S.B., Wang, K. (2019) Implications of K, Cu and Zn isotopes for the formation of tektites. Geochimica et Cosmochimica Acta 259, 170-187.
, and lunar basalts data are from Paniello et al. (2012)Paniello, R.C., Day, J.M.D., Moynier, F. (2012) Zinc isotopic evidence for the origin of the Moon. Nature 490, 376-379.
, Kato et al. (2015)Kato, C., Moynier, F., Valdes, M.C., Dhaliwal, J.K., Day, J.M.D. (2015) Extensive volatile loss during formation and differentiation of the Moon. Nature Communications 6, 7617, doi: 10.1038/ncomms8617.
, and Wang and Jacobsen (2016)Wang, K., Jacobsen, S.B. (2016) Potassium isotopic evidence for a high-energy giant impact origin of the Moon. Nature 538, 487-490.
and references therein.