The primordial He budget of the Earth set by percolative core formation in planetesimals
Affiliations | Corresponding Author | Cite as | Funding information- Share this article
Article views:2,479Cumulative count of HTML views and PDF downloads.
- Download Citation
- Rights & Permissions
top
Abstract

Figures and Tables
![]() Table 1 Helium and Ne isotopic concentrations. The noble gas concentrations are in 10-10 cm3 STP/g (1 cm3 STP corresponds to 2.6868 × 1019 atoms). The 4He concentration in the olivine of the two-day experiment was not measured. The uncertainties are 2 sd and include only ion beam measurement uncertainties and sample mass uncertainties. | ![]() Figure 1 Helium and Ne isotopic compositions in the run products. Materials contain mixtures of atmospheric and cosmogenic noble gases. (a) Olivine-[FeS] pairs have within analytical uncertainties the same 3He/4He ratios except for the experiment conducted at 1200 °C for 2 hours. (b) All olivine-[FeS] pairs have different Ne isotopic compositions. Error bars are 2 sd. | ![]() Figure 2 Metal-silicate (liquid-solid) partition coefficients for He. The dashed line shows the average partition coefficient DHe value of 11.8 (n = 11). The shaded area defines the two standard error of the mean (2 se) of 1.8. Data for the two-hour experiment are excluded and the 4He partition coefficient for the two-day experiment is missing. Uncertainties include only gas concentration uncertainties. Error bars are 2 sd. | ![]() Figure 3 Helium concentration in the Earth’s early core and mantle as a function of the fraction of precursors’ cores equilibration with a terrestrial magma ocean. Mass balance calculations assume 1/3 metal and 2/3 silicate by mass and a He concentration in the bulk Earth that equals 1 (see Supplementary Information). Two scenarios are evaluated whereby metal and silicate (liquid-liquid) equilibrate in a shallow magma ocean (DHe = 0.001) and in a deep magma ocean (DHe = 0.01) (Bouhifd et al., 2013). Datasets for the Earth’s early mantle overlap on the diagram. The inset shows the enrichment factor, i.e. the He concentration in the Earth’s early core at any fraction of equilibration relative to that assuming full (liquid-liquid) equilibrium. The shaded areas represent the range of equilibration from 0 to 36 %, for which geochemical models do not have satisfactory matches between siderophile element abundances and isotopic constraints (Rudge et al., 2010). |
Table 1 | Figure 1 | Figure 2 | Figure 3 |
top
Introduction
The isotope composition of He in Earth’s interior provides key information on the processes of planetary accretion and differentiation. The two isotopes of He, 3He and 4He, are both primordial in origin, but 4He is also produced during Earth’s history by the radioactive decay of the lithophile elements U and Th. Basalts from different geological settings (mid-ocean ridges (Graham, 2002
Graham, D.W. (2002) Noble gas isotope geochemistry of mid-ocean ridge and ocean island basalts: Characterization of mantle source reservoirs. Reviews in Mineralogy and Geochemistry 47, 247-317.
) and a number of ocean islands and continental flood basalt provinces (Kurz et al., 1982Kurz, M.D., Jenkins, W.J., Hart, S.R. (1982) Helium isotopic systematics of oceanic islands and mantle heterogeneity. Nature 297, 43-47.
; Hilton et al., 1999Hilton, D.R., Grönvold, K., Macpherson, C.G., Castillo, P.R. (1999) Extreme 3He/4He ratios in northwest Iceland: constraining the common component in mantle plumes. Earth and Planetary Science Letters 173, 53-60.
; Stuart et al., 2003Stuart, F.M., Lass-Evans, S., Godfrey Fitton, J., Ellam, R.M. (2003) High 3He/4He ratios in picritic basalts from Baffin Island and the role of a mixed reservoir in mantle plumes. Nature 424, 57-59.
; Starkey et al., 2009Starkey, N.A., Stuart, F.M., Ellam, R.M., Fitton, J.G., Basu, S., Larsen, L.M. (2009) Helium isotopes in early Iceland plume picrites: Constraints on the composition of high 3He/4He mantle. Earth and Planetary Science Letters 277, 91-100.
)) have 3He/4He ratios higher than that in air indicating the presence of an undegassed reservoir in the solid Earth rich in 3He. The highest 3He/4He ratios are observed in basalts from ocean islands and continental flood basalt provinces implying a deep mantle origin. The Earth’s core is an attractive location for such a reservoir (Porcelli and Halliday, 2001Porcelli, D., Halliday, A.N. (2001) The core as a possible source of mantle helium. Earth and Planetary Science Letters 192, 45-56.
; Herzberg et al., 2013Herzberg, C., Asimow, P.D., Ionov, D.A., Vidito, C., Jackson, M.G., Geist, D. (2013) Nickel and helium evidence for melt above the core–mantle boundary. Nature 493, 393-397.
). Recent experimental work (Bouhifd et al., 2013Bouhifd, M.A., Jephcoat, A.P., Heber, V.S., Kelley, S.P. (2013) Helium in Earth’s early core. Nature Geoscience 6, 982-986.
) determined He partitioning between liquid silicate and metal, at conditions anticipated in a terrestrial magma ocean. The derived partition coefficients are much smaller than unity, indicating that He prefers to reside in the silicate mantle, rather than the core. Nevertheless, based on estimated concentrations of primordial He, the Earth’s early core could still have incorporated sufficient He to supply the lower mantle to the present day (Bouhifd et al., 2013Bouhifd, M.A., Jephcoat, A.P., Heber, V.S., Kelley, S.P. (2013) Helium in Earth’s early core. Nature Geoscience 6, 982-986.
).The Earth is thought to have largely formed through the accretion of numerous planetesimals and planetary embryos that were already differentiated into a metallic core and silicate mantle (Chambers, 2004
Chambers, J.E. (2004) Planetary accretion in the inner Solar System. Earth and Planetary Science Letters 223, 241-252.
). Due to the significantly lower melting temperature of S-bearing Fe-rich alloys than silicates, it is inevitable that core formation on these planetesimals and small planetary embryos will initially involve the percolation of liquid metal through solid silicate (Yoshino et al., 2003Yoshino, T., Walter, M.J., Katsura, T. (2003) Core formation in planetesimals triggered by permeable flow. Nature 422, 154-157.
; Terasaki et al., 2008Terasaki, H., Frost, D.J., Rubie, D.C., Langenhorst, F. (2008) Percolative core formation in planetesimals. Earth and Planetary Science Letters 273, 132-137.
; Ghanbarzadeh et al., 2017Ghanbarzadeh, S., Hesse, M.A., Prodanović, M. (2017) Percolative core formation in planetesimals enabled by hysteresis in metal connectivity. Proceedings of the National Academy of Sciences 114, 13406-13411.
) prior to the formation of a magma ocean (Greenwood et al., 2005Greenwood, R.C., Franchi, I.A., Jambon, A., Buchanan, P.C. (2005) Widespread magma oceans on asteroidal bodies in the early Solar System. Nature 435, 916-918.
; Elkins-Tanton, 2012Elkins-Tanton, L.T. (2012) Magma oceans in the inner solar system. Annual Review of Earth and Planetary Sciences 40, 113-139.
) and the loss of volatile elements (Porcelli et al., 2001Porcelli, D., Woolum, D., Cassen, P. (2001) Deep Earth rare gases: initial inventories, capture from the solar nebula, and losses during Moon formation. Earth and Planetary Science Letters 193, 237-251.
; Schönbächler et al., 2010Schönbächler, M., Carlson, R.W., Horan, M.F., Mock, T.D., Hauri, E.H. (2010) Heterogeneous accretion and the moderately volatile element budget of Earth. Science 328, 884-887.
; Norris and Wood, 2017Norris, C.A., Wood, B.J. (2017) Earth’s volatile contents established by melting and vaporization. Nature 549, 507-510.
; Pringle and Moynier, 2017Pringle, E.A., Moynier, F. (2017) Rubidium isotopic composition of the Earth, meteorites, and the Moon: Evidence for the origin of volatile loss during planetary accretion. Earth and Planetary Science Letters 473, 62-70.
). Experimental studies (Yoshino et al., 2003Yoshino, T., Walter, M.J., Katsura, T. (2003) Core formation in planetesimals triggered by permeable flow. Nature 422, 154-157.
; Terasaki et al., 2008Terasaki, H., Frost, D.J., Rubie, D.C., Langenhorst, F. (2008) Percolative core formation in planetesimals. Earth and Planetary Science Letters 273, 132-137.
) and numerical simulations (Ghanbarzadeh et al., 2017Ghanbarzadeh, S., Hesse, M.A., Prodanović, M. (2017) Percolative core formation in planetesimals enabled by hysteresis in metal connectivity. Proceedings of the National Academy of Sciences 114, 13406-13411.
) have shown that during this period liquid metal can form an interconnected network and can percolate and segregate by porous flow towards the planetary bodies’ centre. Consequently, He will efficiently partition between liquid metal and solid silicate before isolation of these two phases. Many planetesimals and planetary embryos likely possessed cores that were liquid for several tens of Myr (Chabot and Haack, 2006Chabot, N., Haack, H. (2006) Evolution of asteroidal cores. In: Lauretta, D., McSween Jr., H. (Eds.) Meteorites and the Early Solar System II. University of Arizona Press, Tucson, 747-771.
; Nimmo, 2009Nimmo, F. (2009) Energetics of asteroid dynamos and the role of compositional convection. Geophysical Research Letters 36, L10201.
; Elkins-Tanton et al., 2011Elkins-Tanton, L.T., Weiss, B.P., Zuber, M.T. (2011) Chondrites as samples of differentiated planetesimals. Earth and Planetary Science Letters 305, 1-10.
; Hunt et al., 2018)Hunt, A.C., Cook, D.L., Lichtenberg, T., Reger, P.M., Ek, M., Golabek, G.J., Schönbächler, M. (2018) Late metal–silicate separation on the IAB parent asteroid: Constraints from combined W and Pt isotopes and thermal modelling. Earth and Planetary Science Letters 482, 490-500.
). During impacts the liquid cores of the impactors will disperse or sink rapidly through a magma ocean into the Earth’s proto core. Models suggest that during descent, erosion and emulsification result in mixing and equilibration with Earth’s liquid silicate mantle (Dahl and Stevenson, 2010Dahl, T.W., Stevenson, D.J. (2010) Turbulent mixing of metal and silicate during planet accretion — And interpretation of the Hf–W chronometer. Earth and Planetary Science Letters 295, 177-186.
). However, these models also indicate that large cores (>10 km in diameter) will not emulsify entirely, and perhaps only 1-20 % of Earth’s core would equilibrate with the silicate mantle during accretion. Likewise, Hf-W and U-Pb isotope systems (Rudge et al., 2010Rudge, J.F., Kleine, T., Bourdon, B. (2010) Broad bounds on Earth’s accretion and core formation constrained by geochemical models. Nature Geoscience 3, 439-443.
) and recent N-body accretion simulations (Rubie et al., 2015Rubie, D.C., Jacobson, S.A., Morbidelli, A., O’Brien, D.P., Young, E.D., de Vries, J., Nimmo, F., Palme, H., Frost, D.J. (2015) Accretion and differentiation of the terrestrial planets with implications for the compositions of early-formed Solar System bodies and accretion of water. Icarus 248, 89-108.
) allow for partial equilibration. Consequently, the He budget of the Earth’s core may have been at least partially set in the cores of differentiated planetesimals and planetary embryos that subsequently accreted to form the Earth, rather than later in a terrestrial magma ocean. Here we present new experimental constraints on He partitioning between solid silicate and liquid Fe-rich metal at the conditions expected to prevail during core formation in planetesimals.top
Methods
We performed seven high pressure, high temperature experiments at 1 GPa using a conventional solid-media piston-cylinder apparatus. This pressure corresponds to that at the centre of a planetesimal with a radius of about 100 km. Starting materials were 1:5 mass mixtures of submillimetre-size olivine fragments from the Admire pallasite (stony-iron meteorite) and powdered iron sulphide with a composition of Fe0.705S0.295 (mass fraction; referred to hereafter as [FeS]) corresponding to the eutectic composition at 1 GPa (Fei et al., 1997
Fei, Y., Bertka, C.M., Finger, L.W. (1997) High-pressure iron-sulfur compound, Fe3S2, and melting relations in the Fe-FeS system. Science 275, 1621-1623.
). Although the chemical composition of our metal is likely different from that of most core-forming liquids, no important influence is expected on the partitioning of the noble gases, which are inert. These materials provide two isotopically distinct noble gas sources: cosmogenic noble gases from the meteoritic olivine (3He/4He = 0.1483 ± 0.0020, 20Ne/22Ne = 0.8557 ± 0.0022, and 21Ne/22Ne = 1.0419 ± 0.0018; 2 sd, Table 1) and atmospheric noble gases (essentially no 3He, and 20Ne/22Ne and 21Ne/22Ne of about 9.8 and 0.03, respectively) from the air trapped within the sample capsules. The starting materials were loaded into graphite inner capsules and subsequently sealed in welded Pt outer capsules. Five experiments were conducted at 1200 °C and two at 1450 °C. Both temperatures are above the eutectic of [FeS] and below the melting point of olivine so that [FeS] was molten and olivine remained solid. Experimental run durations ranged from 2 hours to 6 days. After quenching and recovering the samples, olivine and [FeS] aliquots of a few hundred µg were hand-picked using dental tools under a binocular and the noble gases were extracted in vacuum by melting with an infrared laser. Helium and Ne isotopic concentrations were then measured using a magnetic sector field mass spectrometer equipped with a unique high-sensitivity compressor source (Baur, 1999Baur, H. (1999) A noble gas mass spectrometer compressor source with two orders of magnitude improvement in sensitivity. EOS, Transactions of the American Geophysical Union 46, F1118.
) following the procedure given by Riebe et al. (2017)Riebe, M.E.I., Huber, L., Metzler, K., Busemann, H., Luginbuehl, S.M., Meier, M.M.M., Maden, C., Wieler, R. (2017) Cosmogenic He and Ne in chondrules from clastic matrix and a lithic clast of Murchison: No pre-irradiation by the early sun. Geochimica et Cosmochimica Acta 213, 618-634.
. Metal-silicate (i.e. liquid-solid) partition coefficients (Di) were calculated as the ratio of the concentration of a given noble gas isotope i (in cm3 STP/g) in [FeS] and in olivine.top
Results
The observed He and Ne isotopic concentrations in the run products were up to four orders of magnitude lower than in the starting materials (Table 1), indicating that He and Ne were lost by diffusion through the capsule walls. However, the two isotopically distinct noble gas sources in the starting materials provided a proxy for the extent of equilibration between olivine and [FeS]. At equilibrium and assuming no isotopic fractionation, the He and Ne isotopic compositions are expected to be the same in the two phases. As shown in Figure 1a, for each run the 3He/4He ratios in olivine and [FeS] were identical within analytical uncertainties, except for the experiment with the shortest run duration of 2 hours, indicating insufficient time for equilibration. The situation is different for Ne, where isotopic ratios never attain common values (Fig. 1b). While some degree of isotopic equilibration in [FeS] can be inferred from the linear mixing trend observed between the two initial sources, the Ne isotopic composition of olivine does not substantially deviate from the starting cosmogenic composition, probably due to the much lower diffusion coefficient of Ne compared to He (Trull et al., 1991
Trull, T.W., Kurz, M.D., Jenkins, W.J. (1991) Diffusion of cosmogenic 3He in olivine and quartz: implications for surface exposure dating. Earth and Planetary Science Letters 103, 241-256.
; Gourbet et al., 2012Gourbet, L., Shuster, D.L., Balco, G., Cassata, W.S., Renne, P.R., Rood, D. (2012) Neon diffusion kinetics in olivine, pyroxene and feldspar: Retentivity of cosmogenic and nucleogenic neon. Geochimica et Cosmochimica Acta 86, 21-36.
). Nevertheless, our new He data imply that, despite loss of noble gases from the capsules, true equilibrium concentrations between olivine and [FeS] were measured (Fig. 2). Excluding the results from the insufficiently equilibrated experiment (performed for only 2 hours) yielded an average partition coefficient of He between liquid metal and solid silicate (DHe) of 11.8 ± 1.8 (2 se) for the conditions in our experiments. There was no resolvable difference between the partitioning of 3He and 4He (which implies no isotopic fractionation) or between the partitioning values obtained at the different run durations or temperatures. Any major non-systematic cross-contamination of the olivine-[FeS] pairs is unlikely as the derived partition coefficients for the equilibrated experiments are concordant.Table 1 Helium and Ne isotopic concentrations. The noble gas concentrations are in 10-10 cm3 STP/g (1 cm3 STP corresponds to 2.6868 × 1019 atoms). The 4He concentration in the olivine of the two-day experiment was not measured. The uncertainties are 2 sd and include only ion beam measurement uncertainties and sample mass uncertainties.
Sample | Duration | 3He | 4He | 22Ne | 20Ne/22Ne | 21Ne/22Ne | ||||||||||
Olivine start. mat. | 10072 | ± | 133 | 67914 | ± | 120 | 4242.2 | ± | 6.2 | 0.8557 | ± | 0.0022 | 1.0419 | ± | 0.0018 | |
1200 °C | ||||||||||||||||
Olivine | 2 hours | 7.44 | ± | 0.14 | 81 | ± | 12 | 2026.7 | ± | 4.2 | 0.8390 | ± | 0.0023 | 0.9712 | ± | 0.0021 |
[FeS] | 2.607 | ± | 0.041 | 55.7 | ± | 8.5 | 87.70 | ± | 0.26 | 2.262 | ± | 0.085 | 0.8413 | ± | 0.0038 | |
Olivine | 1 day | 0.412 | ± | 0.022 | 7.3 | ± | 3.1 | 889.8 | ± | 1.4 | 0.8534 | ± | 0.0016 | 0.9763 | ± | 0.0017 |
[FeS] | 4.361 | ± | 0.097 | 73.8 | ± | 8.7 | 31.68 | ± | 0.16 | 1.716 | ± | 0.018 | 0.8909 | ± | 0.0059 | |
Olivine | 2 days | 0.284 | ± | 0.017 | - | 1034.8 | ± | 2.4 | 0.8543 | ± | 0.0030 | 0.9756 | ± | 0.0026 | ||
[FeS] | 4.25 | ± | 0.14 | 100 | ± | 27 | 17.59 | ± | 0.57 | 8.43 | ± | 0.34 | 0.1677 | ± | 0.0093 | |
Olivine | 4 days | 0.776 | ± | 0.031 | 12 | ± | 10 | 1407.7 | ± | 2.0 | 0.8735 | ± | 0.0035 | 0.9775 | ± | 0.0017 |
[FeS] | 6.033 | ± | 0.095 | 180.8 | ± | 4.9 | 13.87 | ± | 0.24 | 9.19 | ± | 0.18 | 0.1067 | ± | 0.0039 | |
Olivine | 6 days | 0.465 | ± | 0.013 | 7.1 | ± | 3.2 | 1086.8 | ± | 3.3 | 0.8460 | ± | 0.0027 | 0.9781 | ± | 0.0045 |
[FeS] | 7.18 | ± | 0.15 | 88.4 | ± | 5.3 | 3.12 | ± | 0.23 | 5.95 | ± | 0.49 | 0.325 | ± | 0.042 | |
1450 °C | ||||||||||||||||
Olivine | 2 hours | 1.25 | ± | 0.32 | 19 | ± | 12 | 785.5 | ± | 2.7 | 0.9629 | ± | 0.0063 | 1.0245 | ± | 0.0042 |
[FeS] | 18.37 | ± | 0.55 | 215 | ± | 11 | 6.45 | ± | 0.52 | 4.11 | ± | 0.41 | 0.680 | ± | 0.057 | |
Olivine | 1 day | 0.82 | ± | 0.11 | 17.4 | ± | 9.5 | 58.03 | ± | 0.45 | 0.928 | ± | 0.042 | 1.0116 | ± | 0.0088 |
[FeS] | 8.78 | ± | 0.26 | 116.2 | ± | 9.4 | 6.76 | ± | 0.38 | 7.02 | ± | 0.57 | 0.413 | ± | 0.026 |

Figure 1 Helium and Ne isotopic compositions in the run products. Materials contain mixtures of atmospheric and cosmogenic noble gases. (a) Olivine-[FeS] pairs have within analytical uncertainties the same 3He/4He ratios except for the experiment conducted at 1200 °C for 2 hours. (b) All olivine-[FeS] pairs have different Ne isotopic compositions. Error bars are 2 sd.

Figure 2 Metal-silicate (liquid-solid) partition coefficients for He. The dashed line shows the average partition coefficient DHe value of 11.8 (n = 11). The shaded area defines the two standard error of the mean (2 se) of 1.8. Data for the two-hour experiment are excluded and the 4He partition coefficient for the two-day experiment is missing. Uncertainties include only gas concentration uncertainties. Error bars are 2 sd.
top
Discussion
Our experimental results (Fig. 2, Table 1) show that He behaves as a moderately siderophile element under conditions similar to percolative core formation in planetesimals, and that He is enriched in core-forming liquids. These data can now be used to explore the consequences for the Earth’s primordial He budget assuming that some fraction of the precursor cores contributed to the Earth’s core without complete equilibration in a terrestrial magma ocean for the reasons outlined previously (Dahl and Stevenson, 2010
Dahl, T.W., Stevenson, D.J. (2010) Turbulent mixing of metal and silicate during planet accretion — And interpretation of the Hf–W chronometer. Earth and Planetary Science Letters 295, 177-186.
; Rudge et al., 2010Rudge, J.F., Kleine, T., Bourdon, B. (2010) Broad bounds on Earth’s accretion and core formation constrained by geochemical models. Nature Geoscience 3, 439-443.
; Rubie et al., 2015Rubie, D.C., Jacobson, S.A., Morbidelli, A., O’Brien, D.P., Young, E.D., de Vries, J., Nimmo, F., Palme, H., Frost, D.J. (2015) Accretion and differentiation of the terrestrial planets with implications for the compositions of early-formed Solar System bodies and accretion of water. Icarus 248, 89-108.
). Mass balance calculations (Fig. 3) (see Supplementary Information) can be used to illustrate the He distribution between the Earth’s early core and mantle as a function of the fraction of the precursor cores equilibrating with a magma ocean. At full equilibration, the He concentration in the Earth’s core is entirely set by liquid-liquid partitioning (Bouhifd et al., 2013Bouhifd, M.A., Jephcoat, A.P., Heber, V.S., Kelley, S.P. (2013) Helium in Earth’s early core. Nature Geoscience 6, 982-986.
) (where DHe ranges from 10-2 to 10-3, depending on the average depth of the magma ocean due to pressure dependence of DHe). Due to the lithophile behaviour of He under such conditions, the absolute He concentrations in the Earth’s core are low (Bouhifd et al., 2013Bouhifd, M.A., Jephcoat, A.P., Heber, V.S., Kelley, S.P. (2013) Helium in Earth’s early core. Nature Geoscience 6, 982-986.
). In contrast, based on the conditions studied here, core-forming liquids from planetesimals and planetary embryos are enriched in He by up to four orders of magnitude relative to Fe-rich metal in equilibrium with a magma ocean. Therefore, even small contributions from those enriched liquids to the Earth’s core will have a significant impact on the total He balance. For example, if 90 % of precursor cores equilibrate with the mantle, our model predicts that the He concentration in Earth’s core is 18 to 168 times higher than in the case of full metal-silicate (liquid-liquid) equilibrium, depending on the assumed depth of the magma ocean (Bouhifd et al., 2013Bouhifd, M.A., Jephcoat, A.P., Heber, V.S., Kelley, S.P. (2013) Helium in Earth’s early core. Nature Geoscience 6, 982-986.
). This “enrichment factor” rises to about 110 to 1100 when using the lowest limit of equilibration of 36 % constrained by geochemical systems (Rudge et al., 2010Rudge, J.F., Kleine, T., Bourdon, B. (2010) Broad bounds on Earth’s accretion and core formation constrained by geochemical models. Nature Geoscience 3, 439-443.
). However, even at 99 % equilibration of precursor cores, the enrichment factor is still around 10.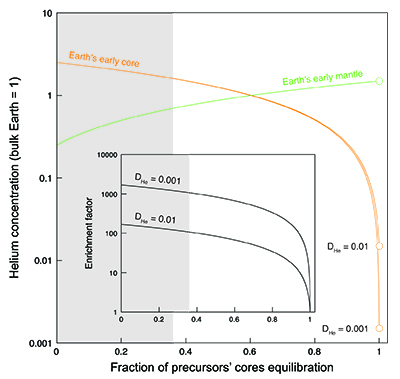
Figure 3 Helium concentration in the Earth’s early core and mantle as a function of the fraction of precursors’ cores equilibration with a terrestrial magma ocean. Mass balance calculations assume 1/3 metal and 2/3 silicate by mass and a He concentration in the bulk Earth that equals 1 (see Supplementary Information). Two scenarios are evaluated whereby metal and silicate (liquid-liquid) equilibrate in a shallow magma ocean (DHe = 0.001) and in a deep magma ocean (DHe = 0.01) (Bouhifd et al., 2013
Bouhifd, M.A., Jephcoat, A.P., Heber, V.S., Kelley, S.P. (2013) Helium in Earth’s early core. Nature Geoscience 6, 982-986.
). Datasets for the Earth’s early mantle overlap on the diagram. The inset shows the enrichment factor, i.e. the He concentration in the Earth’s early core at any fraction of equilibration relative to that assuming full (liquid-liquid) equilibrium. The shaded areas represent the range of equilibration from 0 to 36 %, for which geochemical models do not have satisfactory matches between siderophile element abundances and isotopic constraints (Rudge et al., 2010Rudge, J.F., Kleine, T., Bourdon, B. (2010) Broad bounds on Earth’s accretion and core formation constrained by geochemical models. Nature Geoscience 3, 439-443.
).The new metal-silicate (liquid-solid) partition coefficient for He (Fig. 2) obtained here shows that the Earth’s core may have incorporated significantly more primordial He (= 3He-rich material) than previously considered possible (Bouhifd et al., 2013
Bouhifd, M.A., Jephcoat, A.P., Heber, V.S., Kelley, S.P. (2013) Helium in Earth’s early core. Nature Geoscience 6, 982-986.
) due to a fraction of planetesimal cores that fails to equilibrate with magma ocean liquids. The degree of equilibration and the depth of the magma ocean, for which precise constraints are missing, control the He balance; our new estimates for the initial primordial He concentration in the Earth’s core vary by three orders of magnitude (Fig. 3). The lowest limit of equilibration of 36 % (Rudge et al., 2010Rudge, J.F., Kleine, T., Bourdon, B. (2010) Broad bounds on Earth’s accretion and core formation constrained by geochemical models. Nature Geoscience 3, 439-443.
) should be regarded with caution because more recent accretion models for Earth based on N-body simulations (Rubie et al., 2015Rubie, D.C., Jacobson, S.A., Morbidelli, A., O’Brien, D.P., Young, E.D., de Vries, J., Nimmo, F., Palme, H., Frost, D.J. (2015) Accretion and differentiation of the terrestrial planets with implications for the compositions of early-formed Solar System bodies and accretion of water. Icarus 248, 89-108.
) are only compatible with substantially higher degrees of equilibration.Interestingly, all noble gases behave similarly when partitioning between crystal and melt (Heber et al., 2007
Heber, V.S., Brooker, R.A., Kelley, S.P., Wood, B.J. (2007) Crystal–melt partitioning of noble gases (helium, neon, argon, krypton, and xenon) for olivine and clinopyroxene. Geochimica et Cosmochimica Acta 71, 1041-1061.
) or between metal and silicate (liquid-liquid) (Matsuda et al., 1993Matsuda, J., Sudo, M., Ozima, M., Ito, K., Ohtaka, O., Ito, E. (1993) Noble gas partitioning between metal and silicate under high pressures. Science 259, 788-790.
). Assuming this holds true between metal and silicate (liquid-solid) during percolative core formation, we infer that all noble gases are enriched in core-forming liquids in planetesimals and that the Earth’s core incorporated not only primordial He but also Ne, Ar, Kr, and Xe, although elemental fractionation likely altered their relative initial abundances. In this scenario, the Earth’s core could be the source of other primordial noble gases found in the lower mantle, such as solar-like Ne (Farley and Poreda, 1993Farley, K.A., Poreda, R.J. (1993) Mantle neon and atmospheric contamination. Earth and Planetary Science Letters 114, 325-339.
). Because there is no evidence of direct assimilation of core-derived materials into the silicate Earth (Scherstén et al., 2004Scherstén, A., Elliott, T., Hawkesworth, C., Norman, M. (2004) Tungsten isotope evidence that mantle plumes contain no contribution from the Earth’s core. Nature 427, 234-237.
; Mundl et al., 2017Mundl, A., Touboul, M., Jackson, M.G., Day, J.M.D., Kurz, M.D., Lekic, V., Helz, R.T., Walker, R.J. (2017) Tungsten-182 heterogeneity in modern ocean island basalts. Science 356, 66-69.
), diffusion must be the mechanism by which the noble gases efficiently migrate from the Earth’s outer core to the Earth’s lower mantle. Core crystallisation (Hirose et al., 2017Hirose, K., Morard, G., Sinmyo, R., Umemoto, K., Hernlund, J., Helffrich, G., Labrosse, S. (2017) Crystallization of silicon dioxide and compositional evolution of the Earth’s core. Nature 543, 99-102.
) can be a driving force. The noble gases, which most likely prefer to reside in the liquid, are enriched in the liquid outer core, causing a permanent disequilibrium at the core-mantle boundary. We conclude, based on our mass balance considerations, that the high 3He/4He ratios in basalts (Kurz et al., 1982Kurz, M.D., Jenkins, W.J., Hart, S.R. (1982) Helium isotopic systematics of oceanic islands and mantle heterogeneity. Nature 297, 43-47.
; Hilton et al., 1999Hilton, D.R., Grönvold, K., Macpherson, C.G., Castillo, P.R. (1999) Extreme 3He/4He ratios in northwest Iceland: constraining the common component in mantle plumes. Earth and Planetary Science Letters 173, 53-60.
; Graham, 2002Graham, D.W. (2002) Noble gas isotope geochemistry of mid-ocean ridge and ocean island basalts: Characterization of mantle source reservoirs. Reviews in Mineralogy and Geochemistry 47, 247-317.
; Stuart et al., 2003Stuart, F.M., Lass-Evans, S., Godfrey Fitton, J., Ellam, R.M. (2003) High 3He/4He ratios in picritic basalts from Baffin Island and the role of a mixed reservoir in mantle plumes. Nature 424, 57-59.
; Starkey et al., 2009Starkey, N.A., Stuart, F.M., Ellam, R.M., Fitton, J.G., Basu, S., Larsen, L.M. (2009) Helium isotopes in early Iceland plume picrites: Constraints on the composition of high 3He/4He mantle. Earth and Planetary Science Letters 277, 91-100.
) may well represent primarily the last vestiges of metal-silicate disequilibrium in a terrestrial magma ocean during Earth’s formation.top
Author Contributions
ASGR and HB conceived the idea. ASGR conducted the experiments and analyses with assistance from CL, CM and HB. ASGR, KWB and CL wrote the manuscript. All authors contributed to interpreting the results and commenting the manuscript.
top
Acknowledgements
We thank R. Wieler for enlightening discussions. Funding: this work was carried out in the framework of the National Center for Competence in Research “PlanetS” supported by the Swiss National Science Foundation (SNSF).
Editor: Simon Redfern
top
References
Baur, H. (1999) A noble gas mass spectrometer compressor source with two orders of magnitude improvement in sensitivity. EOS, Transactions of the American Geophysical Union 46, F1118.

Helium and Ne isotopic concentrations were then measured using a magnetic sector field mass spectrometer equipped with a unique high-sensitivity compressor source (Baur, 1999) following the procedure given by Riebe et al. (2017).
View in article
Bouhifd, M.A., Jephcoat, A.P., Heber, V.S., Kelley, S.P. (2013) Helium in Earth’s early core. Nature Geoscience 6, 982-986.

Recent experimental work (Bouhifd et al., 2013) determined He partitioning between liquid silicate and metal, at conditions anticipated in a terrestrial magma ocean.
View in article
Nevertheless, based on estimated concentrations of primordial He, the Earth’s early core could still have incorporated sufficient He to supply the lower mantle to the present day (Bouhifd et al., 2013).
View in article
At full equilibration, the He concentration in the Earth’s core is entirely set by liquid-liquid partitioning (Bouhifd et al., 2013) (where DHe ranges from 10-2 to 10-3, depending on the average depth of the magma ocean due to pressure dependence of DHe)
View in article
Due to the lithophile behaviour of He under such conditions, the absolute He concentrations in the Earth’s core are low (Bouhifd et al., 2013).
View in article
For example, if 90 % of precursor cores equilibrate with the mantle, our model predicts that the He concentration in Earth’s core is 18 to 168 times higher than in the case of full metal-silicate (liquid-liquid) equilibrium, depending on the assumed depth of the magma ocean (Bouhifd et al., 2013).
View in article
Figure 3 [...] Two scenarios are evaluated whereby metal and silicate (liquid-liquid) equilibrate in a shallow magma ocean (DHe = 0.001) and in a deep magma ocean (DHe = 0.01) (Bouhifd et al., 2013).
View in article
The new metal-silicate (liquid-solid) partition coefficient for He (Fig. 2) obtained here shows that the Earth’s core may have incorporated significantly more primordial He (= 3He-rich material) than previously considered possible (Bouhifd et al., 2013) due to a fraction of planetesimal cores that fails to equilibrate with magma ocean liquids.
View in article
Chabot, N., Haack, H. (2006) Evolution of asteroidal cores. In: Lauretta, D., McSween Jr., H. (Eds.) Meteorites and the Early Solar System II. University of Arizona Press, Tucson, 747-771.

Many planetesimals and planetary embryos likely possessed cores that were liquid for several tens of Myr (Chabot and Haack, 2006; Nimmo, 2009; Elkins-Tanton et al., 2011; Hunt et al., 2018).
View in article
Chambers, J.E. (2004) Planetary accretion in the inner Solar System. Earth and Planetary Science Letters 223, 241-252.

The Earth is thought to have largely formed through the accretion of numerous planetesimals and planetary embryos that were already differentiated into a metallic core and silicate mantle (Chambers, 2004).
View in article
Dahl, T.W., Stevenson, D.J. (2010) Turbulent mixing of metal and silicate during planet accretion — And interpretation of the Hf–W chronometer. Earth and Planetary Science Letters 295, 177-186.

Models suggest that during descent, erosion and emulsification result in mixing and equilibration with Earth’s liquid silicate mantle (Dahl and Stevenson, 2010).
View in article
These data can now be used to explore the consequences for the Earth’s primordial He budget assuming that some fraction of the precursor cores contributed to the Earth’s core without complete equilibration in a terrestrial magma ocean for the reasons outlined previously (Dahl and Stevenson, 2010; Rudge et al., 2010; Rubie et al., 2015).
View in article
Elkins-Tanton, L.T. (2012) Magma oceans in the inner solar system. Annual Review of Earth and Planetary Sciences 40, 113-139.

Due to the significantly lower melting temperature of S-bearing Fe-rich alloys than silicates, it is inevitable that core formation on these planetesimals and small planetary embryos will initially involve the percolation of liquid metal through solid silicate (Yoshino et al., 2003; Terasaki et al., 2008; Ghanbarzadeh et al., 2017) prior to the formation of a magma ocean (Greenwood et al., 2005; Elkins-Tanton, 2012) and the loss of volatile elements (Porcelli et al., 2001; Schönbächler et al., 2010; Norris and Wood, 2017; Pringle and Moynier, 2017).
View in article
Elkins-Tanton, L.T., Weiss, B.P., Zuber, M.T. (2011) Chondrites as samples of differentiated planetesimals. Earth and Planetary Science Letters 305, 1-10.

Many planetesimals and planetary embryos likely possessed cores that were liquid for several tens of Myr (Chabot and Haack, 2006; Nimmo, 2009; Elkins-Tanton et al., 2011; Hunt et al., 2018).
View in article
Farley, K.A., Poreda, R.J. (1993) Mantle neon and atmospheric contamination. Earth and Planetary Science Letters 114, 325-339.

In this scenario, the Earth’s core could be the source of other primordial noble gases found in the lower mantle, such as solar-like Ne (Farley and Poreda, 1993).
View in article
Fei, Y., Bertka, C.M., Finger, L.W. (1997) High-pressure iron-sulfur compound, Fe3S2, and melting relations in the Fe-FeS system. Science 275, 1621-1623.

Starting materials were 1:5 mass mixtures of submillimetre-size olivine fragments from the Admire pallasite (stony-iron meteorite) and powdered iron sulphide with a composition of Fe0.705S0.295 (mass fraction; referred to hereafter as [FeS]) corresponding to the eutectic composition at 1 GPa (Fei et al., 1997)
View in article
Ghanbarzadeh, S., Hesse, M.A., Prodanović, M. (2017) Percolative core formation in planetesimals enabled by hysteresis in metal connectivity. Proceedings of the National Academy of Sciences 114, 13406-13411.

Due to the significantly lower melting temperature of S-bearing Fe-rich alloys than silicates, it is inevitable that core formation on these planetesimals and small planetary embryos will initially involve the percolation of liquid metal through solid silicate (Yoshino et al., 2003; Terasaki et al., 2008; Ghanbarzadeh et al., 2017) prior to the formation of a magma ocean (Greenwood et al., 2005; Elkins-Tanton, 2012) and the loss of volatile elements (Porcelli et al., 2001; Schönbächler et al., 2010; Norris and Wood, 2017; Pringle and Moynier, 2017).
View in article
Experimental studies (Yoshino et al., 2003; Terasaki et al., 2008) and numerical simulations (Ghanbarzadeh et al., 2017) have shown that during this period liquid metal can form an interconnected network and can percolate and segregate by porous flow towards the planetary bodies’ centre.
View in article
Gourbet, L., Shuster, D.L., Balco, G., Cassata, W.S., Renne, P.R., Rood, D. (2012) Neon diffusion kinetics in olivine, pyroxene and feldspar: Retentivity of cosmogenic and nucleogenic neon. Geochimica et Cosmochimica Acta 86, 21-36.

While some degree of isotopic equilibration in [FeS] can be inferred from the linear mixing trend observed between the two initial sources, the Ne isotopic composition of olivine does not substantially deviate from the starting cosmogenic composition, probably due to the much lower diffusion coefficient of Ne compared to He (Trull et al., 1991; Gourbet et al., 2012).
View in article
Graham, D.W. (2002) Noble gas isotope geochemistry of mid-ocean ridge and ocean island basalts: Characterization of mantle source reservoirs. Reviews in Mineralogy and Geochemistry 47, 247-317.

Basalts from different geological settings (mid-ocean ridges (Graham, 2002) and a number of ocean islands and continental flood basalt provinces (Kurz et al., 1982; Hilton et al., 1999; Stuart et al., 2003; Starkey et al., 2009)) have 3He/4He ratios higher than that in air indicating the presence of an undegassed reservoir in the solid Earth rich in 3He.
View in article
We conclude, based on our mass balance considerations, that the high 3He/4He ratios in basalts (Kurz et al., 1982; Hilton et al., 1999; Graham, 2002; Stuart et al., 2003; Starkey et al., 2009) may well represent primarily the last vestiges of metal-silicate disequilibrium in a terrestrial magma ocean during Earth’s formation.
View in article
Greenwood, R.C., Franchi, I.A., Jambon, A., Buchanan, P.C. (2005) Widespread magma oceans on asteroidal bodies in the early Solar System. Nature 435, 916-918.

Due to the significantly lower melting temperature of S-bearing Fe-rich alloys than silicates, it is inevitable that core formation on these planetesimals and small planetary embryos will initially involve the percolation of liquid metal through solid silicate (Yoshino et al., 2003; Terasaki et al., 2008; Ghanbarzadeh et al., 2017) prior to the formation of a magma ocean (Greenwood et al., 2005; Elkins-Tanton, 2012) and the loss of volatile elements (Porcelli et al., 2001; Schönbächler et al., 2010; Norris and Wood, 2017; Pringle and Moynier, 2017).
View in article
Heber, V.S., Brooker, R.A., Kelley, S.P., Wood, B.J. (2007) Crystal–melt partitioning of noble gases (helium, neon, argon, krypton, and xenon) for olivine and clinopyroxene. Geochimica et Cosmochimica Acta 71, 1041-1061.

Interestingly, all noble gases behave similarly when partitioning between crystal and melt (Heber et al., 2007) or between metal and silicate (liquid-liquid) (Matsuda et al., 1993).
View in article
Herzberg, C., Asimow, P.D., Ionov, D.A., Vidito, C., Jackson, M.G., Geist, D. (2013) Nickel and helium evidence for melt above the core–mantle boundary. Nature 493, 393-397.

The Earth’s core is an attractive location for such a reservoir (Porcelli and Halliday, 2001; Herzberg et al., 2013).
View in article
Hilton, D.R., Grönvold, K., Macpherson, C.G., Castillo, P.R. (1999) Extreme 3He/4He ratios in northwest Iceland: constraining the common component in mantle plumes. Earth and Planetary Science Letters 173, 53-60.

Basalts from different geological settings (mid-ocean ridges (Graham, 2002) and a number of ocean islands and continental flood basalt provinces (Kurz et al., 1982; Hilton et al., 1999; Stuart et al., 2003; Starkey et al., 2009)) have 3He/4He ratios higher than that in air indicating the presence of an undegassed reservoir in the solid Earth rich in 3He.
View in article
We conclude, based on our mass balance considerations, that the high 3He/4He ratios in basalts (Kurz et al., 1982; Hilton et al., 1999; Graham, 2002; Stuart et al., 2003; Starkey et al., 2009) may well represent primarily the last vestiges of metal-silicate disequilibrium in a terrestrial magma ocean during Earth’s formation.
View in article
Hirose, K., Morard, G., Sinmyo, R., Umemoto, K., Hernlund, J., Helffrich, G., Labrosse, S. (2017) Crystallization of silicon dioxide and compositional evolution of the Earth’s core. Nature 543, 99-102.

Core crystallisation (Hirose et al., 2017) can be a driving force.
View in article
Hunt, A.C., Cook, D.L., Lichtenberg, T., Reger, P.M., Ek, M., Golabek, G.J., Schönbächler, M. (2018) Late metal–silicate separation on the IAB parent asteroid: Constraints from combined W and Pt isotopes and thermal modelling. Earth and Planetary Science Letters 482, 490-500.

Many planetesimals and planetary embryos likely possessed cores that were liquid for several tens of Myr (Chabot and Haack, 2006; Nimmo, 2009; Elkins-Tanton et al., 2011; Hunt et al., 2018).
View in article
Kurz, M.D., Jenkins, W.J., Hart, S.R. (1982) Helium isotopic systematics of oceanic islands and mantle heterogeneity. Nature 297, 43-47.

Basalts from different geological settings (mid-ocean ridges (Graham, 2002) and a number of ocean islands and continental flood basalt provinces (Kurz et al., 1982; Hilton et al., 1999; Stuart et al., 2003; Starkey et al., 2009)) have 3He/4He ratios higher than that in air indicating the presence of an undegassed reservoir in the solid Earth rich in 3He.
View in article
We conclude, based on our mass balance considerations, that the high 3He/4He ratios in basalts (Kurz et al., 1982; Hilton et al., 1999; Graham, 2002; Stuart et al., 2003; Starkey et al., 2009) may well represent primarily the last vestiges of metal-silicate disequilibrium in a terrestrial magma ocean during Earth’s formation.
View in article
Matsuda, J., Sudo, M., Ozima, M., Ito, K., Ohtaka, O., Ito, E. (1993) Noble gas partitioning between metal and silicate under high pressures. Science 259, 788-790.

Interestingly, all noble gases behave similarly when partitioning between crystal and melt (Heber et al., 2007) or between metal and silicate (liquid-liquid) (Matsuda et al., 1993).
View in article
Mundl, A., Touboul, M., Jackson, M.G., Day, J.M.D., Kurz, M.D., Lekic, V., Helz, R.T., Walker, R.J. (2017) Tungsten-182 heterogeneity in modern ocean island basalts. Science 356, 66-69.

Because there is no evidence of direct assimilation of core-derived materials into the silicate Earth (Scherstén et al., 2004; Mundl et al., 2017), diffusion must be the mechanism by which the noble gases efficiently migrate from the Earth’s outer core to the Earth’s lower mantle.
View in article
Nimmo, F. (2009) Energetics of asteroid dynamos and the role of compositional convection. Geophysical Research Letters 36, L10201.

Many planetesimals and planetary embryos likely possessed cores that were liquid for several tens of Myr (Chabot and Haack, 2006; Nimmo, 2009; Elkins-Tanton et al., 2011; Hunt et al., 2018).
View in article
Norris, C.A., Wood, B.J. (2017) Earth’s volatile contents established by melting and vaporization. Nature 549, 507-510.

Due to the significantly lower melting temperature of S-bearing Fe-rich alloys than silicates, it is inevitable that core formation on these planetesimals and small planetary embryos will initially involve the percolation of liquid metal through solid silicate (Yoshino et al., 2003; Terasaki et al., 2008; Ghanbarzadeh et al., 2017) prior to the formation of a magma ocean (Greenwood et al., 2005; Elkins-Tanton, 2012) and the loss of volatile elements (Porcelli et al., 2001; Schönbächler et al., 2010; Norris and Wood, 2017; Pringle and Moynier, 2017).
View in article
Porcelli, D., Halliday, A.N. (2001) The core as a possible source of mantle helium. Earth and Planetary Science Letters 192, 45-56.

The Earth’s core is an attractive location for such a reservoir (Porcelli and Halliday, 2001; Herzberg et al., 2013).
View in article
Porcelli, D., Woolum, D., Cassen, P. (2001) Deep Earth rare gases: initial inventories, capture from the solar nebula, and losses during Moon formation. Earth and Planetary Science Letters 193, 237-251.

Due to the significantly lower melting temperature of S-bearing Fe-rich alloys than silicates, it is inevitable that core formation on these planetesimals and small planetary embryos will initially involve the percolation of liquid metal through solid silicate (Yoshino et al., 2003; Terasaki et al., 2008; Ghanbarzadeh et al., 2017) prior to the formation of a magma ocean (Greenwood et al., 2005; Elkins-Tanton, 2012) and the loss of volatile elements (Porcelli et al., 2001; Schönbächler et al., 2010; Norris and Wood, 2017; Pringle and Moynier, 2017).
View in article
Pringle, E.A., Moynier, F. (2017) Rubidium isotopic composition of the Earth, meteorites, and the Moon: Evidence for the origin of volatile loss during planetary accretion. Earth and Planetary Science Letters 473, 62-70.

Due to the significantly lower melting temperature of S-bearing Fe-rich alloys than silicates, it is inevitable that core formation on these planetesimals and small planetary embryos will initially involve the percolation of liquid metal through solid silicate (Yoshino et al., 2003; Terasaki et al., 2008; Ghanbarzadeh et al., 2017) prior to the formation of a magma ocean (Greenwood et al., 2005; Elkins-Tanton, 2012) and the loss of volatile elements (Porcelli et al., 2001; Schönbächler et al., 2010; Norris and Wood, 2017; Pringle and Moynier, 2017).
View in article
Riebe, M.E.I., Huber, L., Metzler, K., Busemann, H., Luginbuehl, S.M., Meier, M.M.M., Maden, C., Wieler, R. (2017) Cosmogenic He and Ne in chondrules from clastic matrix and a lithic clast of Murchison: No pre-irradiation by the early sun. Geochimica et Cosmochimica Acta 213, 618-634.

Helium and Ne isotopic concentrations were then measured using a magnetic sector field mass spectrometer equipped with a unique high-sensitivity compressor source (Baur, 1999) following the procedure given by Riebe et al. (2017).
View in article
Rubie, D.C., Jacobson, S.A., Morbidelli, A., O’Brien, D.P., Young, E.D., de Vries, J., Nimmo, F., Palme, H., Frost, D.J. (2015) Accretion and differentiation of the terrestrial planets with implications for the compositions of early-formed Solar System bodies and accretion of water. Icarus 248, 89-108.

Likewise, Hf-W and U-Pb isotope systems (Rudge et al., 2010) and recent N-body accretion simulations (Rubie et al., 2015) allow for partial equilibration.
View in article
These data can now be used to explore the consequences for the Earth’s primordial He budget assuming that some fraction of the precursor cores contributed to the Earth’s core without complete equilibration in a terrestrial magma ocean for the reasons outlined previously (Dahl and Stevenson, 2010; Rudge et al., 2010; Rubie et al., 2015).
View in article
The lowest limit of equilibration of 36 % (Rudge et al., 2010) should be regarded with caution because more recent accretion models for Earth based on N-body simulations (Rubie et al., 2015) are only compatible with substantially higher degrees of equilibration.
View in article
Rudge, J.F., Kleine, T., Bourdon, B. (2010) Broad bounds on Earth’s accretion and core formation constrained by geochemical models. Nature Geoscience 3, 439-443.

Likewise, Hf-W and U-Pb isotope systems (Rudge et al., 2010) and recent N-body accretion simulations (Rubie et al., 2015) allow for partial equilibration.
View in article
These data can now be used to explore the consequences for the Earth’s primordial He budget assuming that some fraction of the precursor cores contributed to the Earth’s core without complete equilibration in a terrestrial magma ocean for the reasons outlined previously (Dahl and Stevenson, 2010; Rudge et al., 2010; Rubie et al., 2015).
View in article
This “enrichment factor” rises to about 110 to 1100 when using the lowest limit of equilibration of 36 % constrained by geochemical systems (Rudge et al., 2010).
View in article
Figure 3 [...] The shaded areas represent the range of equilibration from 0 to 36 %, for which geochemical models do not have satisfactory matches between siderophile element abundances and isotopic constraints (Rudge et al., 2010).
View in article
The lowest limit of equilibration of 36 % (Rudge et al., 2010) should be regarded with caution because more recent accretion models for Earth based on N-body simulations (Rubie et al., 2015) are only compatible with substantially higher degrees of equilibration.
View in article
Scherstén, A., Elliott, T., Hawkesworth, C., Norman, M. (2004) Tungsten isotope evidence that mantle plumes contain no contribution from the Earth’s core. Nature 427, 234-237.

Because there is no evidence of direct assimilation of core-derived materials into the silicate Earth (Scherstén et al., 2004; Mundl et al., 2017), diffusion must be the mechanism by which the noble gases efficiently migrate from the Earth’s outer core to the Earth’s lower mantle.
View in article
Schönbächler, M., Carlson, R.W., Horan, M.F., Mock, T.D., Hauri, E.H. (2010) Heterogeneous accretion and the moderately volatile element budget of Earth. Science 328, 884-887.

Due to the significantly lower melting temperature of S-bearing Fe-rich alloys than silicates, it is inevitable that core formation on these planetesimals and small planetary embryos will initially involve the percolation of liquid metal through solid silicate (Yoshino et al., 2003; Terasaki et al., 2008; Ghanbarzadeh et al., 2017) prior to the formation of a magma ocean (Greenwood et al., 2005; Elkins-Tanton, 2012) and the loss of volatile elements (Porcelli et al., 2001; Schönbächler et al., 2010; Norris and Wood, 2017; Pringle and Moynier, 2017).
View in article
Starkey, N.A., Stuart, F.M., Ellam, R.M., Fitton, J.G., Basu, S., Larsen, L.M. (2009) Helium isotopes in early Iceland plume picrites: Constraints on the composition of high 3He/4He mantle. Earth and Planetary Science Letters 277, 91-100.

Basalts from different geological settings (mid-ocean ridges (Graham, 2002) and a number of ocean islands and continental flood basalt provinces (Kurz et al., 1982; Hilton et al., 1999; Stuart et al., 2003; Starkey et al., 2009)) have 3He/4He ratios higher than that in air indicating the presence of an undegassed reservoir in the solid Earth rich in 3He.
View in article
We conclude, based on our mass balance considerations, that the high 3He/4He ratios in basalts (Kurz et al., 1982; Hilton et al., 1999; Graham, 2002; Stuart et al., 2003; Starkey et al., 2009) may well represent primarily the last vestiges of metal-silicate disequilibrium in a terrestrial magma ocean during Earth’s formation.
View in article
Stuart, F.M., Lass-Evans, S., Godfrey Fitton, J., Ellam, R.M. (2003) High 3He/4He ratios in picritic basalts from Baffin Island and the role of a mixed reservoir in mantle plumes. Nature 424, 57-59.

Basalts from different geological settings (mid-ocean ridges (Graham, 2002) and a number of ocean islands and continental flood basalt provinces (Kurz et al., 1982; Hilton et al., 1999; Stuart et al., 2003; Starkey et al., 2009)) have 3He/4He ratios higher than that in air indicating the presence of an undegassed reservoir in the solid Earth rich in 3He.
View in article
We conclude, based on our mass balance considerations, that the high 3He/4He ratios in basalts (Kurz et al., 1982; Hilton et al., 1999; Graham, 2002; Stuart et al., 2003; Starkey et al., 2009) may well represent primarily the last vestiges of metal-silicate disequilibrium in a terrestrial magma ocean during Earth’s formation.
View in article
Terasaki, H., Frost, D.J., Rubie, D.C., Langenhorst, F. (2008) Percolative core formation in planetesimals. Earth and Planetary Science Letters 273, 132-137.

Due to the significantly lower melting temperature of S-bearing Fe-rich alloys than silicates, it is inevitable that core formation on these planetesimals and small planetary embryos will initially involve the percolation of liquid metal through solid silicate (Yoshino et al., 2003; Terasaki et al., 2008; Ghanbarzadeh et al., 2017) prior to the formation of a magma ocean (Greenwood et al., 2005; Elkins-Tanton, 2012) and the loss of volatile elements (Porcelli et al., 2001; Schönbächler et al., 2010; Norris and Wood, 2017; Pringle and Moynier, 2017).
View in article
Experimental studies (Yoshino et al., 2003; Terasaki et al., 2008) and numerical simulations (Ghanbarzadeh et al., 2017) have shown that during this period liquid metal can form an interconnected network and can percolate and segregate by porous flow towards the planetary bodies’ centre.
View in article
Trull, T.W., Kurz, M.D., Jenkins, W.J. (1991) Diffusion of cosmogenic 3He in olivine and quartz: implications for surface exposure dating. Earth and Planetary Science Letters 103, 241-256.

While some degree of isotopic equilibration in [FeS] can be inferred from the linear mixing trend observed between the two initial sources, the Ne isotopic composition of olivine does not substantially deviate from the starting cosmogenic composition, probably due to the much lower diffusion coefficient of Ne compared to He (Trull et al., 1991; Gourbet et al., 2012).
View in article
Yoshino, T., Walter, M.J., Katsura, T. (2003) Core formation in planetesimals triggered by permeable flow. Nature 422, 154-157.

Due to the significantly lower melting temperature of S-bearing Fe-rich alloys than silicates, it is inevitable that core formation on these planetesimals and small planetary embryos will initially involve the percolation of liquid metal through solid silicate (Yoshino et al., 2003; Terasaki et al., 2008; Ghanbarzadeh et al., 2017) prior to the formation of a magma ocean (Greenwood et al., 2005; Elkins-Tanton, 2012) and the loss of volatile elements (Porcelli et al., 2001; Schönbächler et al., 2010; Norris and Wood, 2017; Pringle and Moynier, 2017).
View in article
Experimental studies (Yoshino et al., 2003; Terasaki et al., 2008) and numerical simulations (Ghanbarzadeh et al., 2017) have shown that during this period liquid metal can form an interconnected network and can percolate and segregate by porous flow towards the planetary bodies’ centre.
View in article
top
Supplementary Information
The Supplementary Information includes:
- Noble Gas Mass Spectrometry
- High-pressure Experiments
- Mass Balance Calculations
- Supplementary Information References
Download the Supplementary Information (PDF).
Figures and Tables
Table 1 Helium and Ne isotopic concentrations. The noble gas concentrations are in 10-10 cm3 STP/g (1 cm3 STP corresponds to 2.6868 × 1019 atoms). The 4He concentration in the olivine of the two-day experiment was not measured. The uncertainties are 2 sd and include only ion beam measurement uncertainties and sample mass uncertainties.
Sample | Duration | 3He | 4He | 22Ne | 20Ne/22Ne | 21Ne/22Ne | ||||||||||
Olivine start. mat. | 10072 | ± | 133 | 67914 | ± | 120 | 4242.2 | ± | 6.2 | 0.8557 | ± | 0.0022 | 1.0419 | ± | 0.0018 | |
1200 °C | ||||||||||||||||
Olivine | 2 hours | 7.44 | ± | 0.14 | 81 | ± | 12 | 2026.7 | ± | 4.2 | 0.8390 | ± | 0.0023 | 0.9712 | ± | 0.0021 |
[FeS] | 2.607 | ± | 0.041 | 55.7 | ± | 8.5 | 87.70 | ± | 0.26 | 2.262 | ± | 0.085 | 0.8413 | ± | 0.0038 | |
Olivine | 1 day | 0.412 | ± | 0.022 | 7.3 | ± | 3.1 | 889.8 | ± | 1.4 | 0.8534 | ± | 0.0016 | 0.9763 | ± | 0.0017 |
[FeS] | 4.361 | ± | 0.097 | 73.8 | ± | 8.7 | 31.68 | ± | 0.16 | 1.716 | ± | 0.018 | 0.8909 | ± | 0.0059 | |
Olivine | 2 days | 0.284 | ± | 0.017 | - | 1034.8 | ± | 2.4 | 0.8543 | ± | 0.0030 | 0.9756 | ± | 0.0026 | ||
[FeS] | 4.25 | ± | 0.14 | 100 | ± | 27 | 17.59 | ± | 0.57 | 8.43 | ± | 0.34 | 0.1677 | ± | 0.0093 | |
Olivine | 4 days | 0.776 | ± | 0.031 | 12 | ± | 10 | 1407.7 | ± | 2.0 | 0.8735 | ± | 0.0035 | 0.9775 | ± | 0.0017 |
[FeS] | 6.033 | ± | 0.095 | 180.8 | ± | 4.9 | 13.87 | ± | 0.24 | 9.19 | ± | 0.18 | 0.1067 | ± | 0.0039 | |
Olivine | 6 days | 0.465 | ± | 0.013 | 7.1 | ± | 3.2 | 1086.8 | ± | 3.3 | 0.8460 | ± | 0.0027 | 0.9781 | ± | 0.0045 |
[FeS] | 7.18 | ± | 0.15 | 88.4 | ± | 5.3 | 3.12 | ± | 0.23 | 5.95 | ± | 0.49 | 0.325 | ± | 0.042 | |
1450 °C | ||||||||||||||||
Olivine | 2 hours | 1.25 | ± | 0.32 | 19 | ± | 12 | 785.5 | ± | 2.7 | 0.9629 | ± | 0.0063 | 1.0245 | ± | 0.0042 |
[FeS] | 18.37 | ± | 0.55 | 215 | ± | 11 | 6.45 | ± | 0.52 | 4.11 | ± | 0.41 | 0.680 | ± | 0.057 | |
Olivine | 1 day | 0.82 | ± | 0.11 | 17.4 | ± | 9.5 | 58.03 | ± | 0.45 | 0.928 | ± | 0.042 | 1.0116 | ± | 0.0088 |
[FeS] | 8.78 | ± | 0.26 | 116.2 | ± | 9.4 | 6.76 | ± | 0.38 | 7.02 | ± | 0.57 | 0.413 | ± | 0.026 |

Figure 1 Helium and Ne isotopic compositions in the run products. Materials contain mixtures of atmospheric and cosmogenic noble gases. (a) Olivine-[FeS] pairs have within analytical uncertainties the same 3He/4He ratios except for the experiment conducted at 1200 °C for 2 hours. (b) All olivine-[FeS] pairs have different Ne isotopic compositions. Error bars are 2 sd.
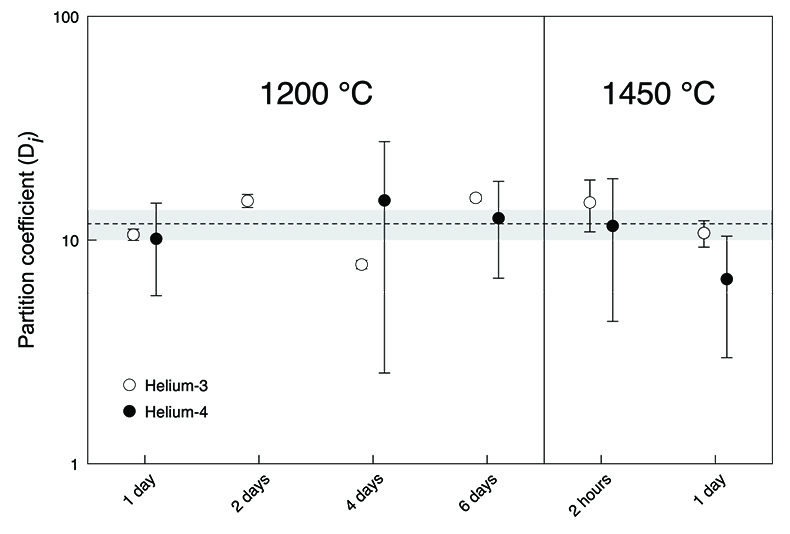
Figure 2 Metal-silicate (liquid-solid) partition coefficients for He. The dashed line shows the average partition coefficient DHe value of 11.8 (n = 11). The shaded area defines the two standard error of the mean (2 se) of 1.8. Data for the two-hour experiment are excluded and the 4He partition coefficient for the two-day experiment is missing. Uncertainties include only gas concentration uncertainties. Error bars are 2 sd.
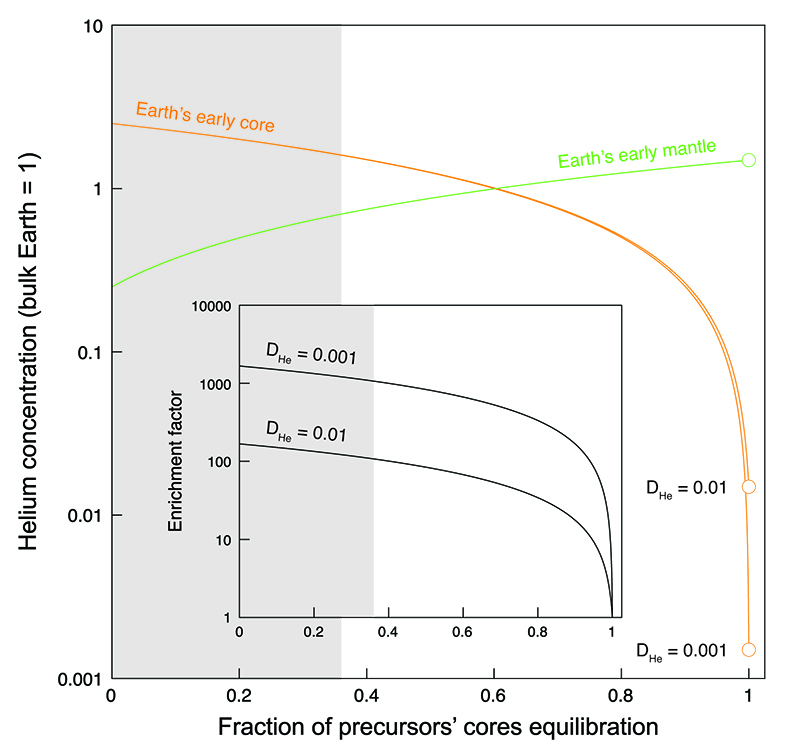
Figure 3 Helium concentration in the Earth’s early core and mantle as a function of the fraction of precursors’ cores equilibration with a terrestrial magma ocean. Mass balance calculations assume 1/3 metal and 2/3 silicate by mass and a He concentration in the bulk Earth that equals 1 (see Supplementary Information). Two scenarios are evaluated whereby metal and silicate (liquid-liquid) equilibrate in a shallow magma ocean (DHe = 0.001) and in a deep magma ocean (DHe = 0.01) (Bouhifd et al., 2013
Bouhifd, M.A., Jephcoat, A.P., Heber, V.S., Kelley, S.P. (2013) Helium in Earth’s early core. Nature Geoscience 6, 982-986.
). Datasets for the Earth’s early mantle overlap on the diagram. The inset shows the enrichment factor, i.e. the He concentration in the Earth’s early core at any fraction of equilibration relative to that assuming full (liquid-liquid) equilibrium. The shaded areas represent the range of equilibration from 0 to 36 %, for which geochemical models do not have satisfactory matches between siderophile element abundances and isotopic constraints (Rudge et al., 2010Rudge, J.F., Kleine, T., Bourdon, B. (2010) Broad bounds on Earth’s accretion and core formation constrained by geochemical models. Nature Geoscience 3, 439-443.
).