Oxidised micrometeorites as evidence for low atmospheric pressure on the early Earth
Affiliations | Corresponding Author | Cite as | Funding information- Share this article
Article views:2,933Cumulative count of HTML views and PDF downloads.
- Download Citation
- Rights & Permissions
top
Abstract

Tomkins, A.G., Bowlt, L., Genge M., Wilson, S.A., Brand, H.E.A., Wykes, J.L. (2016) Ancient micrometeorites suggestive of an oxygen-rich Archaean upper atmosphere. Nature 533, 235–238.
). We here explore the possibility that the mixing ratio of oxygen in Earth’s upper atmosphere, that probed by micrometeorites, may instead be sensitive to the surface atmospheric pressure. We find that the concentrations of oxygen in the upper atmosphere required for micrometeorite oxidation are achieved for a 0.3 bar atmosphere. In this case, significant water vapour reaches high up in the atmosphere and is photodissociated, leading to the formation of molecular oxygen. The presence of oxidised iron in micrometeorites at 2.7 Ga may therefore be further evidence that the atmospheric pressure at the surface of the early Earth was substantially lower than it is today.Figures and Tables
![]() Table 1 Mixing ratios for two model atmospheres. | ![]() Figure 1 Temperature profile for the Earth’s atmosphere with surface pressure of 0.3 bar composed of 33 % N2, 33 % CO2 and 33 % CO (dashed), and 33 % N2, 66 % CO2 (solid). | ![]() Figure 2 Two low pressure model atmospheres of the 3.5 Ga − 2.7 Ga Earth. One model includes a surface mixing ratio of CO of 0.33 (dashed), and the other a surface mixing ratio of 10-6 (solid). The surface pressure is 0.3 bar for both models. Mixing ratios are shown as a function of atmospheric height, h (km; top two figures) and pressure (bar; bottom two figures). | ![]() Figure 3 The minimum O2/CO ratio suggested by the observed iron oxidation in 2.7 Ga micrometeorites (Tomkins et al., 2016), as a function of the melting temperature T [K]. Shaded regions show where there is sufficient O2 (light blue), sufficient temperature (light orange), and the overlap between these two regions (dark orange). The dotted line is the O2/CO ratio in the upper atmosphere for an anoxic atmosphere with a 1 bar surface pressure, and the dashed line is the O2/CO ratio in the upper atmosphere for an anoxic atmosphere with a 0.3 bar surface pressure. |
Table 1 | Figure 1 | Figure 2 | Figure 3 |
top
Introduction
Reconstructing the history of atmospheric oxygen on Earth has been a longstanding, yet elusive, goal of the geosciences. Constraints from several observations now show that a major transition in atmospheric oxygen occurred in the past, the so called ‘Great Oxidation Event’ (GOE). The current paradigm places the GOE at 2.33 Ga (Luo et al., 2016
Luo, G., Ono, S., Beukes, N.J., Wang, D.T., Xie, S., Summons, R.E. (2016) Rapid oxygenation of Earth’s atmosphere 2.33 billion years ago. Science Advances 2, e1600134.
), although recent evidence challenges this (Philippot et al., 2018Philippot, P., Ávila, J.N., Killingsworth, B.A., Tessalina, S., Baton, F., Caquineau, T., Muller, E., Pecoits, E., Cartigny, P., Lalonde, S.V., Ireland, T.R, Thomazo, C., van Kranendonk, M.J., Busigny, V. (2018) Globally asynchronous sulphur isotope signals require re-definition of the Great Oxidation Event. Nature Communications 9, 2245.
). One of the most stringent constraints on the past abundance of atmospheric oxygen comes from the mass independent fractionation of sulphur isotopes (Farquhar et al., 2000Farquhar, J., Bao, H., Thiemens, M. (2000) Atmospheric influence of earth’s earliest sulfur cycle. Science 289, 756–758.
), which limits pre-GOE atmospheric oxygen to <10-5 times present levels. However, all the geochemical, isotopic, and sedimentary proxies commonly used to reconstruct past atmospheric oxygen are incorporated into the geological record by processes occurring in the lower atmosphere.In contrast, Tomkins et al. (2016)
Tomkins, A.G., Bowlt, L., Genge M., Wilson, S.A., Brand, H.E.A., Wykes, J.L. (2016) Ancient micrometeorites suggestive of an oxygen-rich Archaean upper atmosphere. Nature 533, 235–238.
identified that micrometeorites may sample the upper atmosphere, by reacting with the atmosphere during transient heating on entry. Sixty micrometeorites were recovered from the Pilbara region of Australia by Tomkins et al. (2016)Tomkins, A.G., Bowlt, L., Genge M., Wilson, S.A., Brand, H.E.A., Wykes, J.L. (2016) Ancient micrometeorites suggestive of an oxygen-rich Archaean upper atmosphere. Nature 533, 235–238.
, with their age estimated at 2.7 Ga. Tomkins et al. (2016)Tomkins, A.G., Bowlt, L., Genge M., Wilson, S.A., Brand, H.E.A., Wykes, J.L. (2016) Ancient micrometeorites suggestive of an oxygen-rich Archaean upper atmosphere. Nature 533, 235–238.
examined the FeNi metal in the spherules, and determined that they were oxidised while molten, with much of the iron now contained in magnetite (Fe3O4) and wüstite (FeO). The authors argue that this level of oxidation requires modern earth levels of molecular oxygen in the upper atmosphere, 75–90 km above the surface of the Earth. This would be a remarkable result if verified, given the abundant evidence for anoxic conditions at Earth’s surface during this period. The authors explain this observation by appealing to a methane-rich atmosphere, in which an organic haze could have produced a temperature inversion within the mesopause (see Tomkins et al., 2016Tomkins, A.G., Bowlt, L., Genge M., Wilson, S.A., Brand, H.E.A., Wykes, J.L. (2016) Ancient micrometeorites suggestive of an oxygen-rich Archaean upper atmosphere. Nature 533, 235–238.
and citations therein).We here suggest an alternative explanation: that low atmospheric pressure in the Archean led to higher concentrations of molecular oxygen in Earth’s upper atmosphere. Recent evidence from the measured radii of fossilised rain droplets, from the size of volcanic vesicles, and from nitrogen trapped in quartz, suggests that the atmospheric pressure at the surface of the Earth at 2.7 Ga was less than 0.5 bar (e.g., Som et al., 2016
Som, S.M., Buick, R., Hagadorn, J.W., Blake, T.S., Perreault, J.M., Harnmeijer, J.P., Catling, D.C. (2016) Earth’s air pressure 2.7 billion years ago constrained to less than half of modern levels. Nature Geoscience 9, 448–451.
). We find that this low pressure can explain the oxidation of the micrometeoritic iron, with the Pilbara micrometeorites thus providing support for a low pressure atmosphere at 2.7 Ga.top
Methods
We model the global chemistry of low pressure atmospheres for the Earth prior to 2.7 Ga. Our atmospheres are dominated by N2, CO2, CO, and H2O. If the atmosphere of the Earth at this time had pressure <0.8 bar, then the partial pressure of N2 must have been lower than it is today. CO as well as CO2 were plausibly major constituents of volcanic outgassing on the early Earth, with the flux of CO increasing as pressures decreases, to the point that CO becomes the dominant species outgassed when p ≲ 0.1 bar (Gaillard and Scaillet, 2014
Gaillard, F., Scaillet, B. (2014) A theoretical framework for volcanic degassing chemistry in a comparative planetology perspective and implications for planetary atmospheres. Earth and Planetary Science Letters 403, 307–316.
). The ratio of degassed CO/CO2 is dependent on both pressure and the redox state of the crust and mantle. H2O is assumed to be present at vapour pressure.We seek to describe a plausible long term global quasi-steady state atmospheric condition appropriate for the Earth’s atmosphere at 2.7 Ga. To ensure that we have identified a realistic quasi-steady state, we need to account for the global redox balance of the atmosphere and of the atmosphere-ocean system. We follow the approach of Harman et al. (2015)
Harman, C.E., Schwieterman, E.W., Schottelkotte, J.C., Kasting, J.F. (2015) Abiotic O2 Levels on Planets around F, G, K, and M Stars: Possible False Positives for Life? The Astrophysical Journal 812, 137.
, with CO2, N2, and H2O as neutral species. The global redox balance is:Eq. 1
where Φout(Red) is the outgassing flux of reduced species, Φdep(Ox) the deposition flux of oxidised species (either via dry deposition or rainout), Φdep(Red) the deposition flux of reduced species, and Φesc(H2) the escape of H2 (all with units of cm-2 s-1). For now, we will stipulate that the outgassing flux of methane is negligible, and so:
Eq. 2
Also, we use the standard form for escape (adapted from Kasting, 2013
Kasting, J.F. (2013) What caused the rise of atmospheric O2? Chemical Geology 362, 13–25.
).Eq. 3
where vdep(X) [cm s-1] is the deposition velocity of species X, and ƒ0(X) is the surface mixing ratio of species X.
Applying these equations to Equation 1, noting that we are interested in atmospheres with surface pressure of 0.3 bar, we note that there are two independent components, dependent on CO and H2, so we separate these into two independent equations and concentrate on the mixing ratio of CO:
Eq. 4
As with Harman et al. (2015)
Harman, C.E., Schwieterman, E.W., Schottelkotte, J.C., Kasting, J.F. (2015) Abiotic O2 Levels on Planets around F, G, K, and M Stars: Possible False Positives for Life? The Astrophysical Journal 812, 137.
, we will adjust H2 and vdep(H2) to balance out the hydrogen escape. The deposition velocity of CO during the Archean is driven by acetogens converting CO efficiently into acetate, with an equivalent deposition velocity of vdep(CO) = 1.2 × 10-4 cm s-1 (Harman et al., 2015Harman, C.E., Schwieterman, E.W., Schottelkotte, J.C., Kasting, J.F. (2015) Abiotic O2 Levels on Planets around F, G, K, and M Stars: Possible False Positives for Life? The Astrophysical Journal 812, 137.
). The CO deposition is compensated for by CO volcanic outgassing.We consider two cases:
- Volcanically dominated, with volcanic CO ≫ volcanic CO2 (0.3 bar with 33 % N2, 33 % CO, and 33 % CO2).
- Biotically dominated, with negligible CO (0.3 bar with 33 % N2 and 66 % CO2)
Case 1 is appropriate for a >3.5 Ga atmosphere. During this period three effects would have combined to lead plausibly to an atmosphere with equal parts CO and CO2 if the atmospheric surface pressure was low: i) A more reducing crust and plausibly more reducing upper mantle (Yang et al., 2014
Yang, X., Gaillard, F., Scaillet, B. (2014) A relatively reduced Hadean continental crust and implications for the early atmosphere and crustal rheology. Earth and Planetary Science Letters 393, 210.
; Nicklas et al., 2018Nicklas, R.W., Puchtel, I.S., Ash, R.D. (2018) Redox state of the Archean mantle: Evidence from V partitioning in 3.5-2.4 Ga komatiites. Geochimica et Cosmochimica Acta 222, 447-466.
); ii) A lower outgassing pressure favouring the outgassing of CO over CO2 (Gaillard et al., 2011Gaillard, F., Scaillet, B., Arndt, N. (2011) Atmospheric oxygenation caused by a change in volcanic degassing pressure. Nature 478, 229–232.
); and iii) the absence of acetogens to consume the CO. Case 1 is less plausible at 2.7 Ga, because acetogens will now form a major CO sink, and CO outgassing fluxes from the now more oxidised mantle will be lower (Nicklas et al., 2018Nicklas, R.W., Puchtel, I.S., Ash, R.D. (2018) Redox state of the Archean mantle: Evidence from V partitioning in 3.5-2.4 Ga komatiites. Geochimica et Cosmochimica Acta 222, 447-466.
). Case 2 would arise from more moderate outgassing rates of CO and CO2 of Φout(CO) ≈ 3 × 109 cm-2 s-1, suggesting a surface steady state mixing ratio of CO of 3 × 10-6. The initial conditions at the surface used in our model are shown for the two cases in Table 1.To calculate the atmospheric temperature and pressure, we use a 1D climate model developed for high-CO2/high-CH4 terrestrial atmospheres (Pavlov et al., 2000
Pavlov, A.A., Kasting, J.F., Brown, L.L., Rages, K.A., Freedman, R. (2000) Greenhouse warming by CH4 in the atmosphere of early Earth. Journal of Geophysical Research: Planets 105 (E5), 11981-11990.
; Kharecha et al., 2005Kharecha, P., Kasting, J.F., Siefert J. (2005) A coupled atmosphere–ecosystem model of the early Archean earth. Geobiology 3, 53–73.
). For case 1 we model a 0.3 bar atmosphere with 66 % N2 and 33 % CO2. For case 2 we model a 0.3 bar atmosphere with 33 % N2 and 66 % CO2. For case 1, CO is not a greenhouse gas and influences the climate primarily by pressure broadening, incorporated here via the increased N2 concentration. The similar temperature – pressure profiles in cases 1 and 2, despite much larger CO2 concentrations in case 2, indicate that taking N2 to represent the pressure broadening effects of CO is justified (Fig. 1). We use a solar evolution model for the incoming stellar radiation at 3.9 Ga for case 1 and 2.7 Ga for case 2 (Claire et al., 2012Claire, M.W., Sheets, J., Cohen, M., Ribas, I., Meadows, V.S., Catling, D.C. (2012) The Evolution of Solar Flux from 0.1 nm to 160 μm: Quantitative Estimates for Planetary Studies. Astrophysical Journal Letters 757, 95.
). In both cases, the profile is taken to be isothermal above 10-6 bar.Table 1 Mixing ratios for two model atmospheres.
Species | Case 1 | Case 2 |
N2 | 0.33 | 0.33 |
CO2 | 0.33 | 0.66 |
CO | 0.33 | 3 x 10-6 |
H2 | 10-3 | 10-3 |
H2O | Vapour pressure | Vapour pressure |
For the atmospheric chemistry, we use the ARGO photochemistry model (Rimmer and Helling, 2016
Rimmer, P.B., Helling, C. (2016) A Chemical Kinetics Network for Lightning and Life in Planetary Atmospheres. The Astrophysical Journal Supplementary Series 224, 9.
), which solves the photochemistry-transport equation:Eq. 5

where, ni [cm-3] is the number density of species i, i = 1,...,Is, Is being the total number of species. Pi [cm-3 s-1] and Li [cm-3 s-1] represent the production and loss rates calculated from the STAND2016 chemical network and

Rimmer, P.B., Helling, C. (2016) A Chemical Kinetics Network for Lightning and Life in Planetary Atmospheres. The Astrophysical Journal Supplementary Series 224, 9.
). We apply fixed boundary conditions discussed above.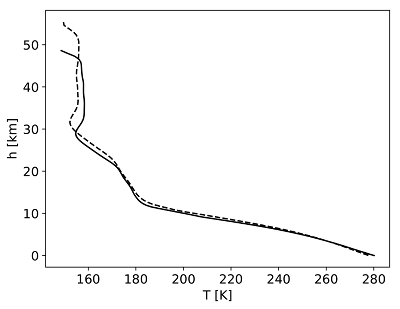
Figure 1 Temperature profile for the Earth’s atmosphere with surface pressure of 0.3 bar composed of 33 % N2, 33 % CO2 and 33 % CO (dashed), and 33 % N2, 66 % CO2 (solid).
top
Results
The chemical profile for a 0.3 bar atmosphere dominated by N2, CO2 and CO is shown in Figure 2. The upper atmosphere is composed of N2, O2 and CO, with O2 mixing ratios achieving modern levels (Tomkins et al., 2016
Tomkins, A.G., Bowlt, L., Genge M., Wilson, S.A., Brand, H.E.A., Wykes, J.L. (2016) Ancient micrometeorites suggestive of an oxygen-rich Archaean upper atmosphere. Nature 533, 235–238.
). It is water vapour that is responsible for the enhancement of O2 within a low pressure atmosphere. A dominant mechanism by which water vapour produces molecular oxygen is as follows:Eq. 6
Eq. 7
Eq. 8
This is similar to the mechanism that can lead to large amounts of oxygen in exoplanets without a cold trap (Wordsworth and Pierrehumbert, 2014
Wordsworth, R., Pierrehumbert, R. (2014) Abiotic Oxygen-dominated Atmospheres on Terrestrial Habitable Zone Planets. Astrophysical Journal Letters 785, L20.
). When CO is present, however, neutral iron (Fe0), acts as a catalyst for the oxidation of CO by O2, at the rates (Smirnov, 2008Smirnov, V.N. (2008) Rate constant of the gas-phase reaction between Fe atoms and CO2. Kinetics and Catalysis 49, 607–609.
and Akhmadov et al., 1988Akhmadov, U.S., Zaslonko, I.S., Smirnov, V.N. (1988) Mechanism and kinetics of interaction of Fe, Cr, Mo, and Mn atoms with molecular oxygen. (English Translation).Kinet. Katal./Kinetics and Catalysis 29, 291-297.
) respectively:Eq. 9
Eq. 10
The balance between Equations 9 and 10 determines the amount of oxygen needed relative to CO, in order to reproduce the observed micrometeoritic iron oxidation. The minimum ratio of O2/CO needed to explain the data is shown in Figure 3. Because of the large energy barrier for reaction (Eq. 9), at temperatures <1700 K, reaction (Eq. 10) is faster than (Eq. 9), and so much more O2 than CO is needed to oxidise the iron sufficiently. At higher temperatures, however, CO can be as much as 50 times as abundant as O2 and oxidation still occurs. Tomkins et al. (2016)
Tomkins, A.G., Bowlt, L., Genge M., Wilson, S.A., Brand, H.E.A., Wykes, J.L. (2016) Ancient micrometeorites suggestive of an oxygen-rich Archaean upper atmosphere. Nature 533, 235–238.
demonstrate that, given the size of the micrometeorites, they would have oxidised while at a temperature above 1600 K. The micrometeorites could, therefore, have been oxidised within a reducing, low pressure, early Earth atmosphere like that shown in Figure 2.
Figure 2 Two low pressure model atmospheres of the 3.5 Ga − 2.7 Ga Earth. One model includes a surface mixing ratio of CO of 0.33 (dashed), and the other a surface mixing ratio of 10-6 (solid). The surface pressure is 0.3 bar for both models. Mixing ratios are shown as a function of atmospheric height, h (km; top two figures) and pressure (bar; bottom two figures).

Figure 3 The minimum O2/CO ratio suggested by the observed iron oxidation in 2.7 Ga micrometeorites (Tomkins et al. (2016)
Tomkins, A.G., Bowlt, L., Genge M., Wilson, S.A., Brand, H.E.A., Wykes, J.L. (2016) Ancient micrometeorites suggestive of an oxygen-rich Archaean upper atmosphere. Nature 533, 235–238.
), as a function of the melting temperature T [K]. Shaded regions show where there is sufficient O2 (light blue), sufficient temperature (light orange), and the overlap between these two regions (dark orange). The dotted line is the O2/CO ratio in the upper atmosphere for an anoxic atmosphere with a 1 bar surface pressure, and the dashed line is the O2/CO ratio in the upper atmosphere for an anoxic atmosphere with a 0.3 bar surface pressure.top
Discussion and Summary
We have shown that the presence of magnetite and wüstite in 2.7 Ga micrometeorites is consistent with the surface pressure of the 2.7 Ga Earth being much less than today. This fits well with other evidence of low atmospheric surface pressures on the Archean Earth (e.g., Som et al., 2016
Som, S.M., Buick, R., Hagadorn, J.W., Blake, T.S., Perreault, J.M., Harnmeijer, J.P., Catling, D.C. (2016) Earth’s air pressure 2.7 billion years ago constrained to less than half of modern levels. Nature Geoscience 9, 448–451.
).Kopparapu et al. (2014)
Kopparapu, R.K., Ramirez, R.M., SchottelKotte, J., Kasting, J.F., Domagal-Goldman, S., Eymet, V. (2014) Habitable Zones around Main-sequence Stars: Dependence on Planetary Mass. Astrophysical Journal Letters 787, L29.
have investigated the effect on climate when atmospheres are at low pressures. At low pressures and without a cold trap, water vapour extends much further into the upper atmosphere. This by itself resolves the Faint Young Sun Paradox (Sagan and Mullen, 1972Sagan, C., Mullen, G. (1972) Earth and Mars: Evolution of Atmospheres and Surface Temperatures. Science 177, 52–56.
), possibly providing too much of a correction as, with water being such a strong greenhouse gas, there is the risk such atmospheres enter a runaway greenhouse state. However, low pressure atmospheres are not necessarily unstable (Kopparapu et al., 2014Kopparapu, R.K., Ramirez, R.M., SchottelKotte, J., Kasting, J.F., Domagal-Goldman, S., Eymet, V. (2014) Habitable Zones around Main-sequence Stars: Dependence on Planetary Mass. Astrophysical Journal Letters 787, L29.
), and the growing evidence for low atmospheric pressures on the 2.7 Ga Earth suggest that somehow the Earth avoided this runaway scenario.A low surface pressure on the early Earth may be explained by a reasonably fast rate of abiotic nitrogen fixation without any efficient abiotic mechanism for restoring nitrogen (Som et al., 2016
Som, S.M., Buick, R., Hagadorn, J.W., Blake, T.S., Perreault, J.M., Harnmeijer, J.P., Catling, D.C. (2016) Earth’s air pressure 2.7 billion years ago constrained to less than half of modern levels. Nature Geoscience 9, 448–451.
). Alternatively, Lammer et al. (2018)Lammer, H., Zerkle, A.L., Gebauer, S., Tosi, N., Noack, L., Scherf, M., Pilat-Lohinger, E., Güdel, M., Grenfell, J.L., Godolt, M., Nikolaou, A. (2018) Origin and evolution of the atmospheres of early Venus, Earth and Mars. The Astronomy and Astrophysics Review 26, 2.
have speculated that the Earth's nitrogen reservoir was largely held within its interior following the lunar impactor stripping the Earth of its primordial atmosphere. The N2 would take some time to build up because of slower N2 outgassing under abiotic conditions (Mikhail et al., 2014Mikhail, S., Sverjensky, D.A. (2014) Nitrogen speciation in upper mantle fluids and the origin of Earth's nitrogen-rich atmosphere. Nature Geoscience 7, 816.
), and more rapid N2 removal from fixation and escape due to heightened solar activity (Lammer et al., 2018Lammer, H., Zerkle, A.L., Gebauer, S., Tosi, N., Noack, L., Scherf, M., Pilat-Lohinger, E., Güdel, M., Grenfell, J.L., Godolt, M., Nikolaou, A. (2018) Origin and evolution of the atmospheres of early Venus, Earth and Mars. The Astronomy and Astrophysics Review 26, 2.
). Lammer et al. (2018)Lammer, H., Zerkle, A.L., Gebauer, S., Tosi, N., Noack, L., Scherf, M., Pilat-Lohinger, E., Güdel, M., Grenfell, J.L., Godolt, M., Nikolaou, A. (2018) Origin and evolution of the atmospheres of early Venus, Earth and Mars. The Astronomy and Astrophysics Review 26, 2.
consider these sources and sinks and estimate that the N2 partial pressure at 2.7 Ga was somewhere between 0.1 and 0.5 bar. The consequences of an initially low and increasing nitrogen content need to be explored, both for understanding the effects of low pressure on the evolution of climate, atmosphere, crust and upper mantle, and for identifying further tests of this low pressure hypothesis.top
Acknowledgements
PBR thanks the Simons Foundation and Kavli Foundation for funding, specifically Simons Foundation SCOL awards 599634, as well as Helmut Lammer for helpful discussions. OS thanks Trinity College Cambridge for support. SR would like to acknowledge the Simons foundation (SCOL award 339489) for their support for this research. All authors thank James F. Kasting and Andy Tomkins for several helpful comments that improved the paper.
Editor: Cin-Ty Lee
top
References
Akhmadov, U.S., Zaslonko, I.S., Smirnov, V.N. (1988) Mechanism and kinetics of interaction of Fe, Cr, Mo, and Mn atoms with molecular oxygen. (English Translation). Kinet. Katal./Kinetics and Catalysis 29, 291-297.

When CO is present, however, neutral iron (Fe0), acts as a catalyst for the oxidation of CO by O2, at the rates (Smirnov, 2008 and Akhmadov et al., 1988) respectively:
Fe + O2 → FeO + O, k9 = 2.1 x 10-10 cm-3 s-1 e-10180K/T, FeO + CO → Fe + CO2
k10 = 7.7 x 10-13 cm-3 s-1 e-490K/T
View in article
Claire, M.W., Sheets, J., Cohen, M., Ribas, I., Meadows, V.S., Catling, D.C. (2012) The Evolution of Solar Flux from 0.1 nm to 160 μm: Quantitative Estimates for Planetary Studies. Astrophysical Journal Letters 757, 95.

We use a solar evolution model for the incoming stellar radiation at 3.9 Ga for case 1 and 2.7 Ga for case 2 (Claire et al., 2012).
View in article
Farquhar, J., Bao, H., Thiemens, M. (2000) Atmospheric influence of earth’s earliest sulfur cycle. Science 289, 756–758.

One of the most stringent constraints on the past abundance of atmospheric oxygen comes from the mass independent fractionation of sulphur isotopes (Farquhar et al., 2000), which limits pre-GOE atmospheric oxygen to <10-5 times present levels.
View in article
Gaillard, F., Scaillet, B. (2014) A theoretical framework for volcanic degassing chemistry in a comparative planetology perspective and implications for planetary atmospheres. Earth and Planetary Science Letters 403, 307–316.

CO as well as CO2 were plausibly major constituents of volcanic outgassing on the early Earth, with the flux of CO increasing as pressures decreases, to the point that CO becomes the dominant species outgassed when p ≲ 0.1 bar (Gaillard and Scaillet, 2014).
View in article
Gaillard, F., Scaillet, B., Arndt, N. (2011) Atmospheric oxygenation caused by a change in volcanic degassing pressure. Nature 478, 229–232.

During this period three effects would have combined to lead plausibly to an atmosphere with equal parts CO and CO2 if the atmospheric surface pressure was low: i) A more reducing crust and plausibly more reducing upper mantle (Yang et al., 2014; Nicklas et al., 2018); ii) A lower outgassing pressure favouring the outgassing of CO over CO2 (Gaillard et al., 2011); and iii) the absence of acetogens to consume the CO.
View in article
Harman, C.E., Schwieterman, E.W., Schottelkotte, J.C., Kasting, J.F. (2015) Abiotic O2 Levels on Planets around F, G, K, and M Stars: Possible False Positives for Life? The Astrophysical Journal 812, 137.

We follow the approach of Harman et al. (2015), with CO2, N2, and H2O as neutral species.
View in article
As with Harman et al. (2015), we will adjust H2 and vdep(H2) to balance out the hydrogen escape.
View in article
The deposition velocity of CO during the Archean is driven by acetogens converting CO efficiently into acetate, with an equivalent deposition velocity of vdep(CO) = 1.2 × 10-4 cm s-1 (Harman et al., 2015).
View in article
Kharecha, P., Kasting, J.F., Siefert J. (2005) A coupled atmosphere–ecosystem model of the early Archean earth. Geobiology 3, 53–73.

To calculate the atmospheric temperature and pressure, we use a 1D climate model developed for high-CO2/high-CH4 terrestrial atmospheres (Pavlov et al., 2000; Kharecha et al., 2005).
View in article
Kasting, J.F. (2013) What caused the rise of atmospheric O2? Chemical Geology 362, 13–25.

Also, we use the standard form for escape (adapted from Kasting, 2013).
View in article
Kopparapu, R.K., Ramirez, R.M., SchottelKotte, J., Kasting, J.F., Domagal-Goldman, S., Eymet, V. (2014) Habitable Zones around Main-sequence Stars: Dependence on Planetary Mass. Astrophysical Journal Letters 787, L29.

Kopparapu et al. (2014) have investigated the effect on climate when atmospheres are at low pressures.
View in article
However, low pressure atmospheres are not necessarily unstable (Kopparapu et al., 2014), and the growing evidence for low atmospheric pressures on the 2.7 Ga Earth suggest that somehow the Earth avoided this runaway scenario.
View in article
Lammer, H., Zerkle, A.L., Gebauer, S., Tosi, N., Noack, L., Scherf, M., Pilat-Lohinger, E., Güdel, M., Grenfell, J.L., Godolt, M., Nikolaou, A. (2018) Origin and evolution of the atmospheres of early Venus, Earth and Mars. The Astronomy and Astrophysics Review 26, 2.

Alternatively, Lammer et al. (2018) have speculated that the Earth's nitrogen reservoir was largely held within its interior following the lunar impactor stripping the Earth of its primordial atmosphere.
View in article
The N2 would take some time to build up because of slower N2 outgassing under abiotic conditions (Mikhail et al., 2014), and more rapid N2 removal from fixation and escape due to heightened solar activity (Lammer et al., 2018).
View in article
Lammer et al. (2018) consider these sources and sinks and estimate that the N2 partial pressure at 2.7 Ga was somewhere between 0.1 and 0.5 bar.
View in article
Luo, G., Ono, S., Beukes, N.J., Wang, D.T., Xie, S., Summons, R.E. (2016) Rapid oxygenation of Earth’s atmosphere 2.33 billion years ago. Science Advances 2, e1600134.

The current paradigm places the GOE at 2.33 Ga (Luo et al., 2016), although recent evidence challenges this (Philippot et al., 2018).
View in article
Mikhail, S., Sverjensky, D.A. (2014) Nitrogen speciation in upper mantle fluids and the origin of Earth's nitrogen-rich atmosphere. Nature Geoscience 7, 816.

The N2 would take some time to build up because of slower N2 outgassing under abiotic conditions (Mikhail et al., 2014), and more rapid N2 removal from fixation and escape due to heightened solar activity (Lammer et al., 2018).
View in article
Nicklas, R.W., Puchtel, I.S., Ash, R.D. (2018) Redox state of the Archean mantle: Evidence from V partitioning in 3.5-2.4 Ga komatiites. Geochimica et Cosmochimica Acta 222, 447-466.

During this period three effects would have combined to lead plausibly to an atmosphere with equal parts CO and CO2 if the atmospheric surface pressure was low: i) A more reducing crust and plausibly more reducing upper mantle (Yang et al., 2014; Nicklas et al., 2018); ii) A lower outgassing pressure favouring the outgassing of CO over CO2 (Gaillard et al., 2011); and iii) the absence of acetogens to consume the CO.
View in article
Case 1 is less plausible at 2.7 Ga, because acetogens will now form a major CO sink, and CO outgassing fluxes from the now more oxidised mantle will be lower (Nicklas et al., 2018).
View in article
Pavlov, A.A., Kasting, J.F., Brown, L.L., Rages, K.A., Freedman, R. (2000) Greenhouse warming by CH4 in the atmosphere of early Earth. Journal of Geophysical Research: Planets 105 (E5), 11981-11990.

To calculate the atmospheric temperature and pressure, we use a 1D climate model developed for high-CO2/high-CH4 terrestrial atmospheres (Pavlov et al., 2000; Kharecha et al., 2005).
View in article
Philippot, P., Ávila, J.N., Killingsworth, B.A., Tessalina, S., Baton, F., Caquineau, T., Muller, E., Pecoits, E., Cartigny, P., Lalonde, S.V., Ireland, T.R, Thomazo, C., van Kranendonk, M.J., Busigny, V. (2018) Globally asynchronous sulphur isotope signals require re-definition of the Great Oxidation Event. Nature Communications 9, 2245.

The current paradigm places the GOE at 2.33 Ga (Luo et al., 2016), although recent evidence challenges this (Philippot et al., 2018).
View in article
Rimmer, P.B., Helling, C. (2016) A Chemical Kinetics Network for Lightning and Life in Planetary Atmospheres. The Astrophysical Journal Supplementary Series 224, 9.

For the atmospheric chemistry, we use the ARGO photochemistry model (Rimmer and Helling, 2016), which solves the photochemistry-transport equation:
where, ni [cm-3] is the number density of species i, i = 1,...,Is, Is being the total number of species. Pi [cm-3 s-1] and Li [cm-3 s-1] represent the production and loss rates calculated from the STAND2016 chemical network and is a vertical flux term capturing eddy and molecular diffusion (Rimmer and Helling, 2016).
View in article
Sagan, C., Mullen, G. (1972) Earth and Mars: Evolution of Atmospheres and Surface Temperatures. Science 177, 52–56.

This by itself resolves the Faint Young Sun Paradox (Sagan and Mullen, 1972), possibly providing too much of a correction as, with water being such a strong greenhouse gas, there is the risk such atmospheres enter a runaway greenhouse state.
View in article
Smirnov, V.N. (2008) Rate constant of the gas-phase reaction between Fe atoms and CO2. Kinetics and Catalysis 49, 607–609.

When CO is present, however, neutral iron (Fe0), acts as a catalyst for the oxidation of CO by O2, at the rates (Smirnov, 2008 and Akhmadov et al., 1988) respectively:
Fe + O2 → FeO + O, k9 = 2.1 x 10-10 cm-3 s-1 e-10180K/T, FeO + CO → Fe + CO2
k10 = 7.7 x 10-13 cm-3 s-1 e-490K/T
View in article
Som, S.M., Buick, R., Hagadorn, J.W., Blake, T.S., Perreault, J.M., Harnmeijer, J.P., Catling, D.C. (2016) Earth’s air pressure 2.7 billion years ago constrained to less than half of modern levels. Nature Geoscience 9, 448–451.

Recent evidence from the measured radii of fossilised rain droplets, from the size of volcanic vesicles, and from nitrogen trapped in quartz, suggests that the atmospheric pressure at the surface of the Earth at 2.7 Ga was less than 0.5 bar (e.g., Som et al., 2016).
View in article
This fits well with other evidence of low atmospheric surface pressures on the Archean Earth (e.g., Som et al., 2016).
View in article
A low surface pressure on the early Earth may be explained by a reasonably fast rate of abiotic nitrogen fixation without any efficient abiotic mechanism for restoring nitrogen (Som et al., 2016).
View in article
Tomkins, A.G., Bowlt, L., Genge M., Wilson, S.A., Brand, H.E.A., Wykes, J.L. (2016) Ancient micrometeorites suggestive of an oxygen-rich Archaean upper atmosphere. Nature 533, 235–238.

Recently, the oxidation state of iron in micrometeorites has been taken to imply the presence of modern Earth concentrations of oxygen in the upper atmosphere at 2.7 Ga, and therefore a highly chemically stratified atmosphere (Tomkins et al., 2016).
View in article
In contrast, Tomkins et al. (2016) identified that micrometeorites may sample the upper atmosphere, by reacting with the atmosphere during transient heating on entry.
View in article
Sixty micrometeorites were recovered from the Pilbara region of Australia by Tomkins et al. (2016), with their age estimated at 2.7 Ga.
View in article
Tomkins et al. (2016) examined the FeNi metal in the spherules, and determined that they were oxidised while molten, with much of the iron now contained in magnetite (Fe3O4) and wüstite (FeO).
View in article
The authors explain this observation by appealing to a methane-rich atmosphere, in which an organic haze could have produced a temperature inversion within the mesopause (see Tomkins et al., 2016 and citations therein).
View in article
The upper atmosphere is composed of N2, O2 and CO, with O2 mixing ratios achieving modern levels (Tomkins et al., 2016).
View in article
Tomkins et al. (2016) demonstrate that, given the size of the micrometeorites, they would have oxidised while at a temperature above 1600 K.
View in article
Figure 3 The minimum O2/CO ratio suggested by the observed iron oxidation in 2.7 Ga micrometeorites (Tomkins et al. (2016)), as a function of the melting temperature T [K].
View in article
Wordsworth, R., Pierrehumbert, R. (2014) Abiotic Oxygen-dominated Atmospheres on Terrestrial Habitable Zone Planets. Astrophysical Journal Letters 785, L20.

This is similar to the mechanism that can lead to large amounts of oxygen in exoplanets without a cold trap (Wordsworth and Pierrehumbert, 2014).
View in article
Yang, X., Gaillard, F., Scaillet, B. (2014) A relatively reduced Hadean continental crust and implications for the early atmosphere and crustal rheology. Earth and Planetary Science Letters 393, 210.

During this period three effects would have combined to lead plausibly to an atmosphere with equal parts CO and CO2 if the atmospheric surface pressure was low: i) A more reducing crust and plausibly more reducing upper mantle (Yang et al., 2014; Nicklas et al., 2018); ii) A lower outgassing pressure favouring the outgassing of CO over CO2 (Gaillard et al., 2011); and iii) the absence of acetogens to consume the CO.
View in article
Figures and Tables
Table 1 Mixing ratios for two model atmospheres.
Species | Case 1 | Case 2 |
N2 | 0.33 | 0.33 |
CO2 | 0.33 | 0.66 |
CO | 0.33 | 3 x 10-6 |
H2 | 10-3 | 10-3 |
H2O | Vapour pressure | Vapour pressure |

Figure 1 Temperature profile for the Earth’s atmosphere with surface pressure of 0.3 bar composed of 33 % N2, 33 % CO2 and 33 % CO (dashed), and 33 % N2, 66 % CO2 (solid).

Figure 2 Two low pressure model atmospheres of the 3.5 Ga − 2.7 Ga Earth. One model includes a surface mixing ratio of CO of 0.33 (dashed), and the other a surface mixing ratio of 10-6 (solid). The surface pressure is 0.3 bar for both models. Mixing ratios are shown as a function of atmospheric height, h (km; top two figures) and pressure (bar; bottom two figures).

Figure 3 The minimum O2/CO ratio suggested by the observed iron oxidation in 2.7 Ga micrometeorites (Tomkins et al. (2016)
Tomkins, A.G., Bowlt, L., Genge M., Wilson, S.A., Brand, H.E.A., Wykes, J.L. (2016) Ancient micrometeorites suggestive of an oxygen-rich Archaean upper atmosphere. Nature 533, 235–238.
), as a function of the melting temperature T [K]. Shaded regions show where there is sufficient O2 (light blue), sufficient temperature (light orange), and the overlap between these two regions (dark orange). The dotted line is the O2/CO ratio in the upper atmosphere for an anoxic atmosphere with a 1 bar surface pressure, and the dashed line is the O2/CO ratio in the upper atmosphere for an anoxic atmosphere with a 0.3 bar surface pressure.