Radiogenic Ca isotopes confirm post-formation K depletion of lower crust
Affiliations | Corresponding Author | Cite as | Funding information- Share this article
Article views:2,570Cumulative count of HTML views and PDF downloads.
- Download Citation
- Rights & Permissions
top
Abstract

Figures and Tables
![]() Figure 1 Garnet grossular content versus (a) whole rock peraluminosity index (A/CNK) and (b) garnet εCa values (for samples containing garnet, n = 14). 2σ uncertainties (±1 for εCa) are smaller than the symbols. Approximate mafic, granitic, and pelitic compositional zones are separated by dashed lines (SI). Darker purple band in (b) represents Bulk Silicate Earth (εCa = 0) composition, corresponding to 40Ca/44Ca = 47.156 (SI). | ![]() Figure 2 Dependence of [K/Ca]protolith on protolith age for samples from Dallwitz Nunatak (NC, n = 12) based on Equation 2. Curves are labelled by sample and delineate constant values for initial εCa at 2.5 Ga with varying protolith ages. Grey band demarcates oldest protolith age found at Dallwitz Nunatak, in the northern Napier Complex (SI). | ![]() Figure 3 Whole rock K/Ca (modern) versus K/Ca (protolith) based on εCa [Equation 2]. NC granulites (n = 10), NC migmatites (n = 2), SP (n = 4), IVZ (n = 3). Contours indicate relative K-loss (in %) assuming constant Ca. Uncertainties in protolith K/Ca are calculated using Equation 2, using our 2 sd on εCa (±1); arrow terminations represent samples within error of BSE. Protolith-metamorphic ages: 3.5-2.5 Ga (NC); 3.0-2.5 Ga (SP); and 0.6-0.3 Ga (IVZ) (SI). Uncertainties for modern K/Ca are <~5 %. | ![]() Figure 4 εCa–based [K/Ca]mdrn/[K/Ca]protolith [Equation 2] versus measured [K2O]mdrn (excluding amphibolites and eclogites). Predictions from our melting model [Equations S-2 through S-4], for various starting compositions (same as in Fig. S-5) varying degree of partial melting (F) (coloured lines). NC granulites (n = 10), NC migmatites (n = 2), SP (n = 4), IVZ (IV-16-24, n = 1). Vertical error bars calculated using Equation 2, combining with those from XRF (<~5 % for K2O/CaO); horizontal error bars are smaller than the symbols. Arrow shows effect of trapped melt on residual solids. Samples plotting above 100 % are consistent with model predictions for high K/Ca protoliths, but petrographic evidence (see SM) suggests that these potential enrichments result from (externally derived) trapped melt. |
Figure 1 | Figure 2 | Figure 3 | Figure 4 |
top
Introduction
Samples from lower continental crust (LCC) can be brought to the surface in two major ways, either (i) as coherent high grade terranes, through tectonic processes, or (ii) as individual xenoliths, through deep-seated volcanism. Based on analysis of these samples, it has been established that much of the LCC was heated to granulite facies conditions (>~800 °C) and lost significant amounts of its original heat producing element budget (Rudnick and Gao, 2014
Rudnick, R.L., Gao, S. (2014) 4.1 – Composition of the Continental Crust. In: Holland, H., Turekian, K. (Eds.) Treatise on Geochemistry. Second Edition, Elsevier, Oxford, 1–51.
), yet it is uncertain whether this is a sufficient explanation for modern heat flow data without invoking greater fractions of mafic crust at depth (Hacker et al., 2015Hacker, B.R., Kelemen, P.B., Behn, M.D. (2015) Continental Lower Crust. Annual Review of Earth and Planetary Sciences 43, 167–205.
).Metamorphic heating results in the breakdown of primary hydrous mineral phases to anhydrous mineral assemblages. Typical reactions involve the transformation of amphibole and mica into feldspar, pyroxene, sillimanite/kyanite, and (at higher pressures) garnet. These transformations are typically accompanied by the generation of granitic liquids (rich in incompatible elements and containing dissolved H2O) that are generally lost from the system, but found preserved as microscopic melt inclusions (e.g., Bartoli et al., 2016
Bartoli, O., Acosta-Vigil, A., Ferrero, S., Cesare, B. (2016) Granitoid magmas preserved as melt inclusions in high-grade metamorphic rocks. American Mineralogist 101, 1543–1559.
; Stepanov et al., 2016Stepanov, A.S., Hermann, J., Rubatto, D., Korsakov, A.V., Danyushevsky, L.V. (2016) Melting history of an ultrahigh-pressure paragneiss revealed by multiphase solid inclusions in garnet, Kokchetav massif, Kazakhstan. Journal of Petrology 57, 1531–1554.
; Ferrero et al., 2018Ferrero, S., Godard, G., Palmieri, R., Wunder, B., Cesare, B. (2018) Partial melting of ultramafic granulites from Dronning Maud Land, Antartica: constraints from melt inclusions and thermodynamic modeling. American Mineralogist 103, 610–622.
). There is also evidence that fluid loss in the absence of melt generation could contribute to the geochemical changes, as suggested by the common observation of high Th/U in granulite facies rocks (Rudnick and Gao, 2014Rudnick, R.L., Gao, S. (2014) 4.1 – Composition of the Continental Crust. In: Holland, H., Turekian, K. (Eds.) Treatise on Geochemistry. Second Edition, Elsevier, Oxford, 1–51.
). Generation and loss of melt, however, is viewed in the more recent literature as being the primary mechanism affecting granulite and ultrahigh temperature (UHT) rocks (e.g., White and Powell, 2002White, R.W., Powell, R. (2002) Melt loss and the preservation of granulite facies mineral assemblages. Journal of Metamorphic Geology 20, 621–632.
; Guernina and Sawyer, 2003Guernina, S., Sawyer, E.W. (2003) Large-scale melt-depletion in granulite terranes: An example from the Archean Ashuanipi subprovince of Quebec. Journal of Metamorphic Geology 21, 181–201.
; Kelsey and Hand, 2015Kelsey, D.E., Hand, M. (2015) On ultrahigh temperature crustal metamorphism: Phase equilibria, trace element thermometry, bulk composition, heat sources, timescales and tectonic settings. Geoscience Frontiers 6, 311–356.
). The lower U, Th, (and K) concentrations and higher density of LCC (Rudnick and Gao, 2014Rudnick, R.L., Gao, S. (2014) 4.1 – Composition of the Continental Crust. In: Holland, H., Turekian, K. (Eds.) Treatise on Geochemistry. Second Edition, Elsevier, Oxford, 1–51.
; Hacker et al., 2015Hacker, B.R., Kelemen, P.B., Behn, M.D. (2015) Continental Lower Crust. Annual Review of Earth and Planetary Sciences 43, 167–205.
) could therefore be attributed to the effects of melt loss during high temperature (HT) metamorphism accompanied by anatexis.Previous work suggesting that U is lost from LCC is based on Pb isotopes and by comparison with Th (Rudnick and Gao, 2014
Rudnick, R.L., Gao, S. (2014) 4.1 – Composition of the Continental Crust. In: Holland, H., Turekian, K. (Eds.) Treatise on Geochemistry. Second Edition, Elsevier, Oxford, 1–51.
), which can also be lost during partial melting at extreme conditions (Ewing et al., 2014Ewing, T.A., Rubatto, D., Hermann, J. (2014) Hafnium isotopes and Zr/Hf of rutile and zircon from lower crustal metapelites (Ivrea-Verbano Zone, Italy): Implications for chemical differentiation of the crust. Earth and Planetary Science Letters 389, 106–118.
; Stepanov et al., 2014Stepanov, A.S., Hermann, J., Korsakov, A.V., Rubatto, D. (2014) Geochemistry of ultrahigh-pressure anatexis: Fractionation of elements in the Kokchetav gneisses during melting at diamond-facies conditions. Contributions to Mineralogy and Petrology 167, 1–25.
). However, ancient K-loss during melting of LCC is hard to constrain because there are few means to establish K content of the protoliths (Rudnick et al., 1985Rudnick, R.L., McLennan, S.M., Taylor, S.R. (1985) Large ion lithophile elements in rocks from high-pressure granulite facies terrains. Geochimica et Cosmochimica Acta 49, 1645–1655.
). Previous studies show that Rb is preferentially lost during granulite facies metamorphism relative to Sr and K (DePaolo et al., 1982DePaolo, D.J., Manton, W.I., Grew, E.S., Halpern, M. (1982) Sm–Nd, Rb–Sr and U–Th–Pb systematics of granulite facies rocks from Fyfe Hills, Enderby Land, Antarctica. Nature 298, 614–618.
; Rudnick et al., 1985Rudnick, R.L., McLennan, S.M., Taylor, S.R. (1985) Large ion lithophile elements in rocks from high-pressure granulite facies terrains. Geochimica et Cosmochimica Acta 49, 1645–1655.
), and that there can also be K loss relative to Ca (Ewing et al., 2014Ewing, T.A., Rubatto, D., Hermann, J. (2014) Hafnium isotopes and Zr/Hf of rutile and zircon from lower crustal metapelites (Ivrea-Verbano Zone, Italy): Implications for chemical differentiation of the crust. Earth and Planetary Science Letters 389, 106–118.
; Stepanov et al., 2014Stepanov, A.S., Hermann, J., Korsakov, A.V., Rubatto, D. (2014) Geochemistry of ultrahigh-pressure anatexis: Fractionation of elements in the Kokchetav gneisses during melting at diamond-facies conditions. Contributions to Mineralogy and Petrology 167, 1–25.
). However, this argument has considerable uncertainty for K when the protoliths are unavailable for analysis, and the magnitude of K loss from LCC remains poorly constrained. To address this problem, we use measurements of radiogenic 40Ca in lower crustal granulite facies rocks and minerals. The K-Ca system is well-suited for this purpose because 40K decays to 40Ca (and 40Ar, t1/2 ~1.25 Gyr), the two elements are separated from each other during partial melting, and the daughter product is generally more compatible than the parent (Marshall and DePaolo, 1989Marshall, B.D., DePaolo, D.J. (1989) Calcium isotopes in igneous rocks and the origin of granite. Geochimica et Cosmochimica Acta 53, 917–922.
).top
Samples and Analytical Procedures
We report radiogenic 40Ca variations (εCa) in granoblastic to porphyroblastic mafic, granitic, and pelitic whole rocks and mineral separates from four localities: the Napier Complex, Antarctica (NC); the Slave Province, Canada (SP); the Ivrea-Verbano Zone, Italy (IVZ); and the Lhasa Block, Tibet (Sumdo eclogite, SE). The rocks span a wide range of chemical compositions and metamorphic pressure and temperature conditions, including granulite/UHT (n = 17), amphibolite (n = 3), and eclogite facies (n = 1), and range in age from Archean to Mesozoic (Supplementary Information, SI, and Tables S-1 through S-4). The SE is not likely to have lost significant amounts of K and is included only for reference (Fig. 1). Ca isotopic compositions were measured by TIMS at the University of California, Berkeley, and are reported in epsilon notation relative to Bulk Silicate Earth (BSE, SI, Table S-5), according to Equation 1.
Eq. 1

top
εCa in Low-K Metamorphic Rocks and Minerals
Garnet is an ideal mineral for εCa analyses because it effectively excludes K, is easy to separate, and commonly contains substantial amounts of Ca. Given a clean mineral separation, any excess radiogenic Ca in garnet must be inherited from 40K that decayed to 40Ca in the protolith, prior to garnet formation. We find that clean garnet separates have εCa ranging from 0 to +42 (Table S-5). Higher εCa values are found in garnets with lower grossular content [molar Ca/(Ca+Fe+Mg+Mn) < ~5 %], from rocks with generally higher whole rock peraluminosity [defined as molar Al2O3/(CaO+Na2O+K2O) and denoted A/CNK in Fig. 1a]. In mafic granulites, both garnet and low-K plagioclase separates (which were sampled in the absence of garnet), have a narrow εCa range from 0 to +3 (Fig. 1b). Whole rock and high-K feldspar separates were also measured (Fig. S-1) in order to obtain rough K-Ca isochrons (Fig. S-2), which are in general agreement with more precise dating methods for the various regions (SI).

Figure 1 Garnet grossular content versus (a) whole rock peraluminosity index (A/CNK) and (b) garnet εCa values (for samples containing garnet, n = 14). 2σ uncertainties (±1 for εCa) are smaller than the symbols. Approximate mafic, granitic, and pelitic compositional zones are separated by dashed lines (SI). Darker purple band in (b) represents Bulk Silicate Earth (εCa = 0) composition, corresponding to 40Ca/44Ca = 47.156 (SI).
top
Protolith K/Ca Estimates
Large depletions in K associated with metamorphism can be detected with our data on garnet mineral separates. Garnet and whole rock measurements for 2040C (NC), for example, have indistinguishable (yet highly elevated) εCa values (+12.5, see Table S-5) due to a nearly complete loss of K from the rock during metamorphism, resulting in a near zero K/Ca similar to that of garnet. For rocks where K-loss is less extreme, protolith K/Ca values are evaluated from εCa (garnet or low-K plagioclase/whole rocks, SI) and the time interval between protolith formation and granulite facies metamorphism.
Using the metamorphic and protolith ages for each locality (‘two-stage-model’, SI), we are able to estimate the protolith K/Ca values using Equation 2, adapted from Marshall and DePaolo (1989)
Marshall, B.D., DePaolo, D.J. (1989) Calcium isotopes in igneous rocks and the origin of granite. Geochimica et Cosmochimica Acta 53, 917–922.
.Eq. 2

Where εCa(t2) and εCa(t1) are εCa values at metamorphic age (t2) and protolith age (t1), respectively; λK is the total decay constant of 40K (assumed 0.554 Gyr-1), and QCa (~1.0804) is a factor incorporating the branching ratio of 40K decay and the abundances of 40K, 44Ca, and 40Ca relative to BSE.
In Figure 2, we show the effect of protolith age uncertainty on [K/Ca]protolith for our NC samples, based on Equation 2. This equation provides a minimum constraint on [K/Ca]protolith values because of three assumptions implicit in our use of the equation: (i) the oldest protolith age estimates for the various localities, (ii) single stage evolution from BSE [εCa(t1) = 0] to garnet εCa values [εCa(t2)], and (iii) that garnets form out of the bulk protolith Ca pool at the time of metamorphism. Younger protolith ages, multiple stages for [K/Ca]protolith (e.g., increases driven by weathering/metasomatic processes), and/or partial loss of radiogenic Ca from K-bearing minerals prior to garnet formation (through earlier melting events), would all require higher [K/Ca]protolith to reach the same initial εCa values at the time of metamorphism.

Figure 2 Dependence of [K/Ca]protolith on protolith age for samples from Dallwitz Nunatak (NC, n = 12) based on Equation 2. Curves are labelled by sample and delineate constant values for initial εCa at 2.5 Ga with varying protolith ages. Grey band demarcates oldest protolith age found at Dallwitz Nunatak, in the northern Napier Complex (SI).
top
Potassium Loss during High-T Metamorphism
Including data from the other localities, we find as expected that mafic samples have lower [K/Ca]protolith (<1), and pelitic samples have higher [K/Ca]protolith ranging from 1 to ~13. Comparing these estimates with [K/Ca]modern in the whole rocks (Fig. 3), we are able to assess K-mobility during granulite metamorphism (see Fig. S-3 for data labels).

Figure 3 Whole rock K/Ca (modern) versus K/Ca (protolith) based on εCa [Equation 2]. NC granulites (n = 10), NC migmatites (n = 2), SP (n = 4), IVZ (n = 3). Contours indicate relative K-loss (in %) assuming constant Ca. Uncertainties in protolith K/Ca are calculated using Equation 2, using our 2 sd on εCa (±1); arrow terminations represent samples within error of BSE. Protolith-metamorphic ages: 3.5-2.5 Ga (NC); 3.0-2.5 Ga (SP); and 0.6-0.3 Ga (IVZ) (SI). Uncertainties for modern K/Ca are <~5 %.
Our results indicate that most granulites have measured K/Ca that is substantially lower than that calculated for the protolith, indicating significant K-loss during metamorphism. The samples fall into 3 rough groups: one group has no K-loss, or a slight K enrichment, and the other groups cluster at 67 % and 97 %; one NC sample suggests greater than 99.9 % K-loss (Fig. 3). Samples with younger ages and lower εCa have larger uncertainties. Two pelitic granulites and migmatites from the NC have an apparent increase in K/Ca relative to their protolith compositions, which can potentially be explained by the presence of (externally derived) captured melt. Our data suggest that granulite facies samples with less than ~2 wt. % modern whole rock K2O have generally lost K, and samples with >2 wt. % have generally gained K (relative to Ca, Fig. S-4). Although we are unaware of K-loss estimates for the NC and SP, our data agree with previous estimates for the IVZ, where granulite facies samples have about 2/3 less K than their lower temperature (amphibolite facies) counterparts (Ewing et al., 2014
Ewing, T.A., Rubatto, D., Hermann, J. (2014) Hafnium isotopes and Zr/Hf of rutile and zircon from lower crustal metapelites (Ivrea-Verbano Zone, Italy): Implications for chemical differentiation of the crust. Earth and Planetary Science Letters 389, 106–118.
). Our results are also in agreement with previous work on the Napier complex that found Pb and Sr isotope evidence for significant losses of Rb relative to Sr and U relative to Th (e.g., DePaolo et al., 1982DePaolo, D.J., Manton, W.I., Grew, E.S., Halpern, M. (1982) Sm–Nd, Rb–Sr and U–Th–Pb systematics of granulite facies rocks from Fyfe Hills, Enderby Land, Antarctica. Nature 298, 614–618.
; Kelsey and Hand, 2015Kelsey, D.E., Hand, M. (2015) On ultrahigh temperature crustal metamorphism: Phase equilibria, trace element thermometry, bulk composition, heat sources, timescales and tectonic settings. Geoscience Frontiers 6, 311–356.
).top
Melt-loss Modelling
Although there is still some debate as to whether or not granulites need externally derived fluids in order to initiate melting (Aranovich et al., 2016
Aranovich, L.Y., Makhluf, A.R., Manning, C.E., Newton, R.C., Touret, J.L.R. (2016) Fluids, Melting, Granulites and Granites: A Controversy - Reply to the Commentary of J.D. Clemens, I.S. Buick and G. Stevens. Precambrian Research 278, 400–404.
; Clemens et al., 2016Clemens, J.D., Buick, I.S., Stevens, G. (2016) Fluids, melting, granulites and granites: A commentary. Precambrian Research 278, 394–399.
), the conclusion from melting experiments (Gao et al., 2016Gao, P., Zheng, Y.F., Zhao, Z.F. (2016) Experimental melts from crustal rocks: A lithochemical constraint on granite petrogenesis. Lithos 266–267, 133–157.
) and from trapped melt inclusions in peritectic minerals (Bartoli et al., 2016Bartoli, O., Acosta-Vigil, A., Ferrero, S., Cesare, B. (2016) Granitoid magmas preserved as melt inclusions in high-grade metamorphic rocks. American Mineralogist 101, 1543–1559.
; Stepanov et al., 2016Stepanov, A.S., Hermann, J., Rubatto, D., Korsakov, A.V., Danyushevsky, L.V. (2016) Melting history of an ultrahigh-pressure paragneiss revealed by multiphase solid inclusions in garnet, Kokchetav massif, Kazakhstan. Journal of Petrology 57, 1531–1554.
; Ferrero et al., 2018Ferrero, S., Godard, G., Palmieri, R., Wunder, B., Cesare, B. (2018) Partial melting of ultramafic granulites from Dronning Maud Land, Antartica: constraints from melt inclusions and thermodynamic modeling. American Mineralogist 103, 610–622.
) is that granulites are often associated with loss of granitic melt. Given that we can quantify relative K/Ca decreases, we are also able to place constraints on the amount of melt loss required to form our samples by modelling the partitioning of K and Ca between melt and residual minerals.To estimate K/Ca fractionation during partial melting, we use a modified non-modal batch melting model, where the bulk distribution coefficient for K (DK) is a function of the mass fraction of remaining residual K-feldspar (the most significant K-bearing mineral at granulite facies conditions), and the bulk distribution coefficient for Ca (DCa) is a function of the mass fraction of residual plagioclase + clinopyroxene (SI, Fig. S-5).
top
Model Results
Comparing our model estimates for [K/Ca]solid/[K/Ca]o versus [K2O]solid (at various values of F) to our εCa-based [K/Ca]mdrn/[K/Ca]protolith estimates and [K2O]mdrn analyses for granulite facies samples, and assuming that melt is completely lost from the system, we find that most of the samples are consistent with 20-30 % melting, with the most extreme sample suggesting melt fractions of ~50 % (Fig. 4). Although the distribution coefficients used in our model are currently rough estimates, varying K and Ca distribution coefficients over a range of likely values (Fig. S-6) does not significantly change the results, which depend most significantly on [K/Ca]protolith.
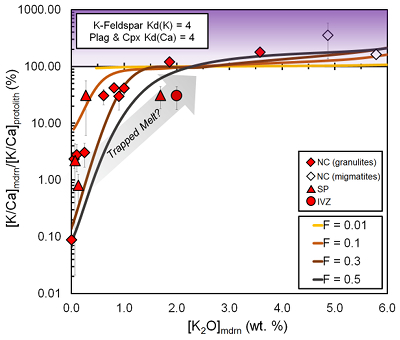
Figure 4 εCa–based [K/Ca]mdrn/[K/Ca]protolith [Equation 2] versus measured [K2O]mdrn (excluding amphibolites and eclogites). Predictions from our melting model [Equations S-2 through S-4], for various starting compositions (same as in Fig. S-5) varying degree of partial melting (F) (coloured lines). NC granulites (n = 10), NC migmatites (n = 2), SP (n = 4), IVZ (IV-16-24, n = 1). Vertical error bars calculated using Equation 2, combining with those from XRF (<~5 % for K2O/CaO); horizontal error bars are smaller than the symbols. Arrow shows effect of trapped melt on residual solids. Samples plotting above 100 % are consistent with model predictions for high K/Ca protoliths, but petrographic evidence (see SM) suggests that these potential enrichments result from (externally derived) trapped melt.
The model results generally agree with other approaches for estimating melt production during granulite facies metamorphism, including pseudosection analyses (White and Powell, 2002
White, R.W., Powell, R. (2002) Melt loss and the preservation of granulite facies mineral assemblages. Journal of Metamorphic Geology 20, 621–632.
; Redler et al., 2012Redler, C., Johnson, T.E., White, R.W., Kunz, B.E. (2012) Phase equilibrium constraints on a deep crustal metamorphic field gradient: Metapelitic rocks from the Ivrea Zone (NW Italy). Journal of Metamorphic Geology 30, 235–254.
; Yakymchuk and Brown, 2014Yakymchuk, C., Brown, M. (2014) Consequences of open-system melting in tectonics. Journal of the Geological Society 171, 21–40.
; Green et al., 2016Green, E.C.R., White, R.W., Diener, J.F.A., Powell, R., Holland, T.J.B., Palin, R.M. (2016) Activity–composition relations for the calculation of partial melting equilibria in metabasic rocks. Journal of Metamorphic Geology 34, 845–869.
; Palin et al., 2016Palin, R.M., White, R.W., Green, E.C.R. (2016) Partial melting of metabasic rocks and the generation of tonalitic–trondhjemitic–granodioritic (TTG) crust in the Archaean: Constraints from phase equilibrium modelling. Precambrian Research 287, 73–90.
) and other methods (Guernina and Sawyer, 2003Guernina, S., Sawyer, E.W. (2003) Large-scale melt-depletion in granulite terranes: An example from the Archean Ashuanipi subprovince of Quebec. Journal of Metamorphic Geology 21, 181–201.
; Bartoli, 2017Bartoli, O. (2017) Phase equilibria modelling of residual migmatites and granulites: An evaluation of the melt-reintegration approach. Journal of Metamorphic Geology 35, 919–942.
). These estimates typically range from ~20-50 % total melt depending on protolith compositions and P-T-time conditions.top
Discussion and Conclusions
Although our assumption of complete melt segregation may be an overestimate, significant melt loss is a common feature associated with granulite facies terranes (e.g., Brown, 2002
Brown, M. (2002) Retrograde processes in migmatites and granulites revisited. Journal of Metamorphic Geology 20, 25–40.
; Guernina and Sawyer, 2003Guernina, S., Sawyer, E.W. (2003) Large-scale melt-depletion in granulite terranes: An example from the Archean Ashuanipi subprovince of Quebec. Journal of Metamorphic Geology 21, 181–201.
), and a minimum of 50-70 % of generated melt (assuming 40 % total melt) must be lost in order to retain a HT mineral assemblage (White and Powell, 2002White, R.W., Powell, R. (2002) Melt loss and the preservation of granulite facies mineral assemblages. Journal of Metamorphic Geology 20, 621–632.
). If melt is not fully lost from the system, our model requires greater total melt fractions in order to match [K/Ca]mdrn measured in the samples today. This suggests that our melting model (which uses minimum [K/Ca]protolith estimates and assumes total loss of melt), provides minimum estimates for total melt fractions.Based on εCa data, and assuming conservative protolith ages for each locality, we find that many LCC samples from the Napier Complex, Ivrea-Verbano Zone, and the Slave Province have undergone significant amounts of K depletion, with relative K/Ca decreases ranging from ~60 % to greater than 95 %. These results confirm that K, which is inferred to be the most important heat producing element in the Archean, is efficiently mobilised and removed from the lower crust during HT metamorphism. This observation implies that greater fractions of mafic rock in the lower crust are not necessary to explain modern heat flow data, and supports crustal evolution models where continental stabilisation is promoted through HT metamorphism and depletion of radioactive elements from the lower crust.
top
Acknowledgements
We thank N. Botto, S. Matveev, A. Locock, W. Yang, T. Teague, and S.T. Brown for their technical expertise, C. Yakymchuk and S. Ferrero for helpful discussions, and N.J. Pester for modern hydrothermal fluid samples. This research was primarily supported by a U.S. National Science Foundation (EAR100500) grant to DJD. TC acknowledges funding from a Natural Sciences and Engineering Research Council of Canada (NSERC) discovery grant, and MAA acknowledges NSERC postgraduate funding that aided in supporting this work. ESG thanks the Australian Antarctic Division for the opportunity to participate in the 1977-1978 Australian National Antarctic Research Expedition and for logistic support, and acknowledges NSF grant DPP 76-80957 to UCLA.
Editor: Helen Williams
top
Author Contributions
MAA and DJD designed research; MAA performed analyses; MAA, DJD, and TC analysed data; ESG, TC, DR and DJD provided samples collected in the field; MAA and DJD wrote the paper with input from TC, DR and ESG.
top
References
Aranovich, L.Y., Makhluf, A.R., Manning, C.E., Newton, R.C., Touret, J.L.R. (2016) Fluids, Melting, Granulites and Granites: A Controversy - Reply to the Commentary of J.D. Clemens, I.S. Buick and G. Stevens. Precambrian Research 278, 400–404.

Although there is still some debate as to whether or not granulites need externally derived fluids in order to initiate melting (Aranovich et al., 2016; Clemens et al., 2016), the conclusion from melting experiments (Gao et al., 2016) and from trapped melt inclusions in peritectic minerals (Bartoli et al., 2016; Stepanov et al., 2016; Ferrero et al., 2018) is that granulites are often associated with loss of granitic melt.
View in article
Bartoli, O. (2017) Phase equilibria modelling of residual migmatites and granulites: An evaluation of the melt-reintegration approach. Journal of Metamorphic Geology 35, 919–942.

The model results generally agree with other approaches for estimating melt production during granulite facies metamorphism, including pseudosection analyses (White and Powell, 2002; Redler et al., 2012; Yakymchuk and Brown, 2014; Green et al., 2016; Palin et al., 2016) and other methods (Guernina and Sawyer, 2003; Bartoli, 2017).
View in article
Bartoli, O., Acosta-Vigil, A., Ferrero, S., Cesare, B. (2016) Granitoid magmas preserved as melt inclusions in high-grade metamorphic rocks. American Mineralogist 101, 1543–1559.

These transformations are typically accompanied by the generation of granitic liquids (rich in incompatible elements and containing dissolved H2O) that are generally lost from the system, but found preserved as microscopic melt inclusions (e.g., Bartoli et al., 2016; Stepanov et al., 2016; Ferrero et al., 2018).
View in article
Although there is still some debate as to whether or not granulites need externally derived fluids in order to initiate melting (Aranovich et al., 2016; Clemens et al., 2016), the conclusion from melting experiments (Gao et al., 2016) and from trapped melt inclusions in peritectic minerals (Bartoli et al., 2016; Stepanov et al., 2016; Ferrero et al., 2018) is that granulites are often associated with loss of granitic melt.
View in article
Brown, M. (2002) Retrograde processes in migmatites and granulites revisited. Journal of Metamorphic Geology 20, 25–40.

Although our assumption of complete melt segregation may be an overestimate, significant melt loss is a common feature associated with granulite facies terranes (e.g., Brown, 2002; Guernina and Sawyer, 2003), and a minimum of 50-70 % of generated melt (assuming 40 % total melt) must be lost in order to retain a HT mineral assemblage (White and Powell, 2002).
View in article
Clemens, J.D., Buick, I.S., Stevens, G. (2016) Fluids, melting, granulites and granites: A commentary. Precambrian Research 278, 394–399.

Although there is still some debate as to whether or not granulites need externally derived fluids in order to initiate melting (Aranovich et al., 2016; Clemens et al., 2016), the conclusion from melting experiments (Gao et al., 2016) and from trapped melt inclusions in peritectic minerals (Bartoli et al., 2016; Stepanov et al., 2016; Ferrero et al., 2018) is that granulites are often associated with loss of granitic melt.
View in article
DePaolo, D.J., Manton, W.I., Grew, E.S., Halpern, M. (1982) Sm–Nd, Rb–Sr and U–Th–Pb systematics of granulite facies rocks from Fyfe Hills, Enderby Land, Antarctica. Nature 298, 614–618.

Previous studies show that Rb is preferentially lost during granulite facies metamorphism relative to Sr and K (DePaolo et al., 1982; Rudnick et al., 1985), and that there can also be K loss relative to Ca (Ewing et al., 2014; Stepanov et al., 2014).
View in article
Our results are also in agreement with previous work on the Napier complex that found Pb and Sr isotope evidence for significant losses of Rb relative to Sr and U relative to Th (e.g., DePaolo et al., 1982; Kelsey and Hand, 2015).
View in article
Ewing, T.A., Rubatto, D., Hermann, J. (2014) Hafnium isotopes and Zr/Hf of rutile and zircon from lower crustal metapelites (Ivrea-Verbano Zone, Italy): Implications for chemical differentiation of the crust. Earth and Planetary Science Letters 389, 106–118.

Previous work suggesting that U is lost from LCC is based on Pb isotopes and by comparison with Th (Rudnick and Gao, 2014), which can also be lost during partial melting at extreme conditions (Ewing et al., 2014; Stepanov et al., 2014).
View in article
Previous studies show that Rb is preferentially lost during granulite facies metamorphism relative to Sr and K (DePaolo et al., 1982; Rudnick et al., 1985), and that there can also be K loss relative to Ca (Ewing et al., 2014; Stepanov et al., 2014).
View in article
Although we are unaware of K-loss estimates for the NC and SP, our data agree with previous estimates for the IVZ, where granulite facies samples have about 2/3 less K than their lower temperature (amphibolite facies) counterparts (Ewing et al., 2014).
View in article
Ferrero, S., Godard, G., Palmieri, R., Wunder, B., Cesare, B. (2018) Partial melting of ultramafic granulites from Dronning Maud Land, Antartica: constraints from melt inclusions and thermodynamic modeling. American Mineralogist 103, 610–622.

These transformations are typically accompanied by the generation of granitic liquids (rich in incompatible elements and containing dissolved H2O) that are generally lost from the system, but found preserved as microscopic melt inclusions (e.g., Bartoli et al., 2016; Stepanov et al., 2016; Ferrero et al., 2018).
View in article
Although there is still some debate as to whether or not granulites need externally derived fluids in order to initiate melting (Aranovich et al., 2016; Clemens et al., 2016), the conclusion from melting experiments (Gao et al., 2016) and from trapped melt inclusions in peritectic minerals (Bartoli et al., 2016; Stepanov et al., 2016; Ferrero et al., 2018) is that granulites are often associated with loss of granitic melt.
View in article
Gao, P., Zheng, Y.F., Zhao, Z.F. (2016) Experimental melts from crustal rocks: A lithochemical constraint on granite petrogenesis. Lithos 266–267, 133–157.

Although there is still some debate as to whether or not granulites need externally derived fluids in order to initiate melting (Aranovich et al., 2016; Clemens et al., 2016), the conclusion from melting experiments (Gao et al., 2016) and from trapped melt inclusions in peritectic minerals (Bartoli et al., 2016; Stepanov et al., 2016; Ferrero et al., 2018) is that granulites are often associated with loss of granitic melt.
View in article
Green, E.C.R., White, R.W., Diener, J.F.A., Powell, R., Holland, T.J.B., Palin, R.M. (2016) Activity–composition relations for the calculation of partial melting equilibria in metabasic rocks. Journal of Metamorphic Geology 34, 845–869.

The model results generally agree with other approaches for estimating melt production during granulite facies metamorphism, including pseudosection analyses (White and Powell, 2002; Redler et al., 2012; Yakymchuk and Brown, 2014; Green et al., 2016; Palin et al., 2016) and other methods (Guernina and Sawyer, 2003; Bartoli, 2017).
View in article
Guernina, S., Sawyer, E.W. (2003) Large-scale melt-depletion in granulite terranes: An example from the Archean Ashuanipi subprovince of Quebec. Journal of Metamorphic Geology 21, 181–201.

Generation and loss of melt, however, is viewed in the more recent literature as being the primary mechanism affecting granulite and ultrahigh temperature (UHT) rocks (e.g., White and Powell, 2002; Guernina and Sawyer, 2003; Kelsey and Hand, 2015).
View in article
The model results generally agree with other approaches for estimating melt production during granulite facies metamorphism, including pseudosection analyses (White and Powell, 2002; Redler et al., 2012; Yakymchuk and Brown, 2014; Green et al., 2016; Palin et al., 2016) and other methods (Guernina and Sawyer, 2003; Bartoli, 2017).
View in article
Although our assumption of complete melt segregation may be an overestimate, significant melt loss is a common feature associated with granulite facies terranes (e.g., Brown, 2002; Guernina and Sawyer, 2003), and a minimum of 50-70 % of generated melt (assuming 40 % total melt) must be lost in order to retain a HT mineral assemblage (White and Powell, 2002).
View in article
Hacker, B.R., Kelemen, P.B., Behn, M.D. (2015) Continental Lower Crust. Annual Review of Earth and Planetary Sciences 43, 167–205.

Based on analysis of these samples, it has been established that much of the LCC was heated to granulite facies conditions (>~800 °C) and lost significant amounts of its original heat producing element budget (Rudnick and Gao, 2014), yet it is uncertain whether this is a sufficient explanation for modern heat flow data without invoking greater fractions of mafic crust at depth (Hacker et al., 2015).
View in article
The lower U, Th, (and K) concentrations and higher density of LCC (Rudnick and Gao, 2014; Hacker et al., 2015) could therefore be attributed to the effects of melt loss during high temperature (HT) metamorphism accompanied by anatexis.
View in article
Kelsey, D.E., Hand, M. (2015) On ultrahigh temperature crustal metamorphism: Phase equilibria, trace element thermometry, bulk composition, heat sources, timescales and tectonic settings. Geoscience Frontiers 6, 311–356.

Generation and loss of melt, however, is viewed in the more recent literature as being the primary mechanism affecting granulite and ultrahigh temperature (UHT) rocks (e.g., White and Powell, 2002; Guernina and Sawyer, 2003; Kelsey and Hand, 2015).
View in article
Our results are also in agreement with previous work on the Napier complex that found Pb and Sr isotope evidence for significant losses of Rb relative to Sr and U relative to Th (e.g., DePaolo et al., 1982; Kelsey and Hand, 2015).
View in article
Marshall, B.D., DePaolo, D.J. (1989) Calcium isotopes in igneous rocks and the origin of granite. Geochimica et Cosmochimica Acta 53, 917–922.

The K-Ca system is well-suited for this purpose because 40K decays to 40Ca (and 40Ar, t1/2 ~1.25 Gyr), the two elements are separated from each other during partial melting, and the daughter product is generally more compatible than the parent (Marshall and DePaolo, 1989).
View in article
Using the metamorphic and protolith ages for each locality (‘two-stage-model’, SI), we are able to estimate the protolith K/Ca values using Equation 2, adapted from Marshall and DePaolo (1989).
View in article
Palin, R.M., White, R.W., Green, E.C.R. (2016) Partial melting of metabasic rocks and the generation of tonalitic–trondhjemitic–granodioritic (TTG) crust in the Archaean: Constraints from phase equilibrium modelling. Precambrian Research 287, 73–90.

The model results generally agree with other approaches for estimating melt production during granulite facies metamorphism, including pseudosection analyses (White and Powell, 2002; Redler et al., 2012; Yakymchuk and Brown, 2014; Green et al., 2016; Palin et al., 2016) and other methods (Guernina and Sawyer, 2003; Bartoli, 2017).
View in article
Redler, C., Johnson, T.E., White, R.W., Kunz, B.E. (2012) Phase equilibrium constraints on a deep crustal metamorphic field gradient: Metapelitic rocks from the Ivrea Zone (NW Italy). Journal of Metamorphic Geology 30, 235–254.

The model results generally agree with other approaches for estimating melt production during granulite facies metamorphism, including pseudosection analyses (White and Powell, 2002; Redler et al., 2012; Yakymchuk and Brown, 2014; Green et al., 2016; Palin et al., 2016) and other methods (Guernina and Sawyer, 2003; Bartoli, 2017).
View in article
Rudnick, R.L., Gao, S. (2014) 4.1 – Composition of the Continental Crust. In: Holland, H., Turekian, K. (Eds.) Treatise on Geochemistry. Second Edition, Elsevier, Oxford, 1–51.

Based on analysis of these samples, it has been established that much of the LCC was heated to granulite facies conditions (>~800 °C) and lost significant amounts of its original heat producing element budget (Rudnick and Gao, 2014), yet it is uncertain whether this is a sufficient explanation for modern heat flow data without invoking greater fractions of mafic crust at depth (Hacker et al., 2015).
View in article
There is also evidence that fluid loss in the absence of melt generation could contribute to the geochemical changes, as suggested by the common observation of high Th/U in granulite facies rocks (Rudnick and Gao, 2014).
View in article
The lower U, Th, (and K) concentrations and higher density of LCC (Rudnick and Gao, 2014; Hacker et al., 2015) could therefore be attributed to the effects of melt loss during high temperature (HT) metamorphism accompanied by anatexis.
View in article
Previous work suggesting that U is lost from LCC is based on Pb isotopes and by comparison with Th (Rudnick and Gao, 2014), which can also be lost during partial melting at extreme conditions (Ewing et al., 2014; Stepanov et al., 2014).
View in article
Rudnick, R.L., McLennan, S.M., Taylor, S.R. (1985) Large ion lithophile elements in rocks from high-pressure granulite facies terrains. Geochimica et Cosmochimica Acta 49, 1645–1655.

However, ancient K-loss during melting of LCC is hard to constrain because there are few means to establish K content of the protoliths (Rudnick et al., 1985).
View in article
Previous studies show that Rb is preferentially lost during granulite facies metamorphism relative to Sr and K (DePaolo et al., 1982; Rudnick et al., 1985), and that there can also be K loss relative to Ca (Ewing et al., 2014; Stepanov et al., 2014).
View in article
Stepanov, A.S., Hermann, J., Korsakov, A.V., Rubatto, D. (2014) Geochemistry of ultrahigh-pressure anatexis: Fractionation of elements in the Kokchetav gneisses during melting at diamond-facies conditions. Contributions to Mineralogy and Petrology 167, 1–25.

Previous work suggesting that U is lost from LCC is based on Pb isotopes and by comparison with Th (Rudnick and Gao, 2014), which can also be lost during partial melting at extreme conditions (Ewing et al., 2014; Stepanov et al., 2014).
View in article
Previous studies show that Rb is preferentially lost during granulite facies metamorphism relative to Sr and K (DePaolo et al., 1982; Rudnick et al., 1985), and that there can also be K loss relative to Ca (Ewing et al., 2014; Stepanov et al., 2014).
View in article
Stepanov, A.S., Hermann, J., Rubatto, D., Korsakov, A.V., Danyushevsky, L.V. (2016) Melting history of an ultrahigh-pressure paragneiss revealed by multiphase solid inclusions in garnet, Kokchetav massif, Kazakhstan. Journal of Petrology 57, 1531–1554.

Although there is still some debate as to whether or not granulites need externally derived fluids in order to initiate melting (Aranovich et al., 2016; Clemens et al., 2016), the conclusion from melting experiments (Gao et al., 2016) and from trapped melt inclusions in peritectic minerals (Bartoli et al., 2016; Stepanov et al., 2016; Ferrero et al., 2018) is that granulites are often associated with loss of granitic melt.
View in article
These transformations are typically accompanied by the generation of granitic liquids (rich in incompatible elements and containing dissolved H2O) that are generally lost from the system, but found preserved as microscopic melt inclusions (e.g., Bartoli et al., 2016; Stepanov et al., 2016; Ferrero et al., 2018).
View in article
White, R.W., Powell, R. (2002) Melt loss and the preservation of granulite facies mineral assemblages. Journal of Metamorphic Geology 20, 621–632.

Generation and loss of melt, however, is viewed in the more recent literature as being the primary mechanism affecting granulite and ultrahigh temperature (UHT) rocks (e.g., White and Powell, 2002; Guernina and Sawyer, 2003; Kelsey and Hand, 2015).
View in article
The model results generally agree with other approaches for estimating melt production during granulite facies metamorphism, including pseudosection analyses (White and Powell, 2002; Redler et al., 2012; Yakymchuk and Brown, 2014; Green et al., 2016; Palin et al., 2016) and other methods (Guernina and Sawyer, 2003; Bartoli, 2017).
View in article
Although our assumption of complete melt segregation may be an overestimate, significant melt loss is a common feature associated with granulite facies terranes (e.g., Brown, 2002; Guernina and Sawyer, 2003), and a minimum of 50-70 % of generated melt (assuming 40 % total melt) must be lost in order to retain a HT mineral assemblage (White and Powell, 2002).
View in article
Yakymchuk, C., Brown, M. (2014) Consequences of open-system melting in tectonics. Journal of the Geological Society 171, 21–40.

The model results generally agree with other approaches for estimating melt production during granulite facies metamorphism, including pseudosection analyses (White and Powell, 2002; Redler et al., 2012; Yakymchuk and Brown, 2014; Green et al., 2016; Palin et al., 2016) and other methods (Guernina and Sawyer, 2003; Bartoli, 2017).
View in article
top
Supplementary Information
The Supplementary Information includes:
- Sample Descriptions and Petrography
- Methods
- Tables S-1 to S-6
- Figures S-1 to S-6
- Supplementary Information References
Download the Supplementary Information (PDF).
Figures and Tables

Figure 1 Garnet grossular content versus (a) whole rock peraluminosity index (A/CNK) and (b) garnet εCa values (for samples containing garnet, n = 14). 2σ uncertainties (±1 for εCa) are smaller than the symbols. Approximate mafic, granitic, and pelitic compositional zones are separated by dashed lines (SI). Darker purple band in (b) represents Bulk Silicate Earth (εCa = 0) composition, corresponding to 40Ca/44Ca = 47.156 (SI).
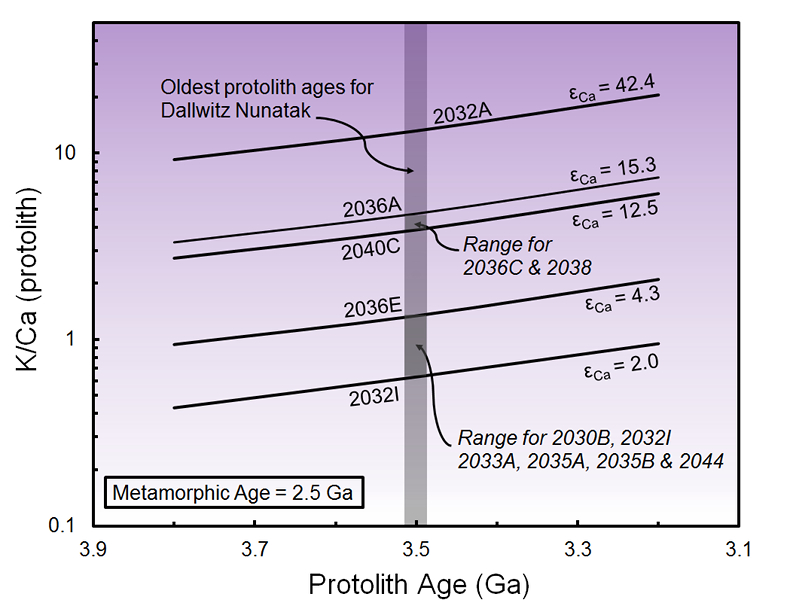
Figure 2 Dependence of [K/Ca]protolith on protolith age for samples from Dallwitz Nunatak (NC, n = 12) based on Equation 2. Curves are labelled by sample and delineate constant values for initial εCa at 2.5 Ga with varying protolith ages. Grey band demarcates oldest protolith age found at Dallwitz Nunatak, in the northern Napier Complex (SI).

Figure 3 Whole rock K/Ca (modern) versus K/Ca (protolith) based on εCa [Equation 2]. NC granulites (n = 10), NC migmatites (n = 2), SP (n = 4), IVZ (n = 3). Contours indicate relative K-loss (in %) assuming constant Ca. Uncertainties in protolith K/Ca are calculated using Equation 2, using our 2 sd on εCa (±1); arrow terminations represent samples within error of BSE. Protolith-metamorphic ages: 3.5-2.5 Ga (NC); 3.0-2.5 Ga (SP); and 0.6-0.3 Ga (IVZ) (SI). Uncertainties for modern K/Ca are <~5 %.
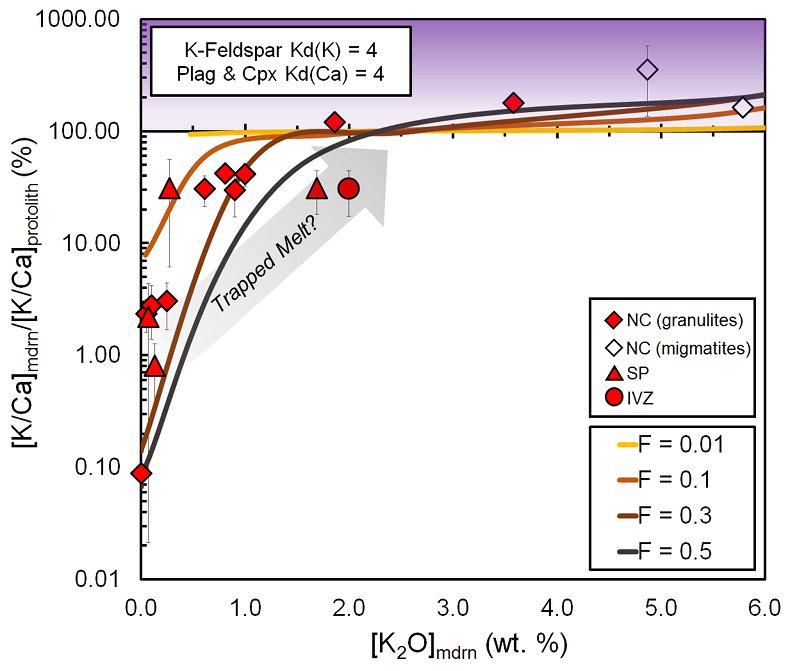
Figure 4 εCa–based [K/Ca]mdrn/[K/Ca]protolith [Equation 2] versus measured [K2O]mdrn (excluding amphibolites and eclogites). Predictions from our melting model [Equations S-2 through S-4], for various starting compositions (same as in Fig. S-5) varying degree of partial melting (F) (coloured lines). NC granulites (n = 10), NC migmatites (n = 2), SP (n = 4), IVZ (IV-16-24, n = 1). Vertical error bars calculated using Equation 2, combining with those from XRF (<~5 % for K2O/CaO); horizontal error bars are smaller than the symbols. Arrow shows effect of trapped melt on residual solids. Samples plotting above 100 % are consistent with model predictions for high K/Ca protoliths, but petrographic evidence (see SM) suggests that these potential enrichments result from (externally derived) trapped melt.