Redox transfer at subduction zones: insights from Fe isotopes in the Mariana forearc
Affiliations | Corresponding Author | Cite as | Funding information- Share this article
Article views:2,463Cumulative count of HTML views and PDF downloads.
- Download Citation
- Rights & Permissions
top
Abstract
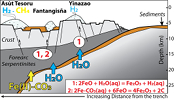
Figures and Tables
![]() Figure 1 Distribution of δ56Fe values in forearc serpentinites. (a) Bathymetry map of the Mariana subduction system showing the locations of the mud volcanoes drilled during IODP Expedition 366 and the ODP Leg 195. Hole locations are indicated in red circles. (b) Iron isotope compositions of bulk ultramafic clasts from the Mariana forearc, organised according to the distance from the trench. DM: Depleted Mantle (Craddock et al., 2013). | ![]() Figure 2 Plots of δ56Fe versus (a) B concentrations, (b) As/Yb and (c) Fe3+/ΣFe ratios in Mariana ultramafic clasts. The black bar represents the FeCl standard reproducibility during analyses. DTS-1, DTS-2b, JP-1: dunite-like reference materials. | ![]() Figure 3 Cartoon illustrating redox and isotopic transfers between the slab and the forearc mantle wedge. |
Figure 1 | Figure 2 | Figure 3 |
top
Introduction
Subduction zones regulate chemical exchange between the Earth’s surface and interior, modulating the long term cycling of redox sensitive elements such as iron, carbon and sulfur (Evans, 2012
Evans, K.A. (2012) The redox budget of subduction zones. Earth-Science Reviews 113, 11–32.
). Recent iron isotope studies (δ56Fe) have shown that iron, which was previously considered to be insoluble, can be mobilised in slab-derived fluids by complexation with chloride and/or oxidising ligands (e.g., Fe(II)-CO3 or Fe(II)-SO4; Debret et al., 2016Debret, B., Millet, M.-A., Pons, M.-L., Bouilhol, P., Inglis, E., Williams, H. (2016) Isotopic evidence for iron mobility during subduction. Geology 44, 215–218.
, 2018Debret, B., Bouilhol, P., Pons, M.L., Williams, H. (2018) Carbonate Transfer during the Onset of Slab Devolatilization: New Insights from Fe and Zn Stable Isotopes. Journal of Petrology 59, 1145–1166.
). Importantly, these complexes preferentially concentrate light (low) δ56Fe, driving stable isotope fractionation (Debret et al., 2016Debret, B., Millet, M.-A., Pons, M.-L., Bouilhol, P., Inglis, E., Williams, H. (2016) Isotopic evidence for iron mobility during subduction. Geology 44, 215–218.
, 2018Debret, B., Bouilhol, P., Pons, M.L., Williams, H. (2018) Carbonate Transfer during the Onset of Slab Devolatilization: New Insights from Fe and Zn Stable Isotopes. Journal of Petrology 59, 1145–1166.
). These findings concur with the high Fe3+/ΣFe (Kelley and Cottrell, 2009Kelley, K.A., Cottrell, E. (2009) Water and the oxidation state of subduction zone magmas. Science 325, 605–607.
) and light δ56Fe (Nebel et al., 2015Nebel, O., Sossi, P.A., Bénard, A., Wille, M., Vroon, P.Z., Arculus, R.J. (2015) Redox-variability and controls in subduction zones from an iron-isotope perspective. Earth and Planetary Science Letters 432, 142–151.
) of arc lavas relative to mid-ocean ridge basalts, although the origin of light δ56Fe in arc lavas remains an active research frontier. Iron stable isotopes are therefore a potentially powerful tool to track the mobility of iron and the fate of oxidised fluids across subduction zones. However, questions remain as to whether iron is sufficiently concentrated in slab-derived fluids to influence the δ56Fe of the sub-arc mantle and if so, do links between the oxidation state and the δ56Fe of mantle wedge peridotites exist?The transfer of redox sensitive elements across subduction zones involves complex and multi-stage processes (Nebel et al., 2015
Nebel, O., Sossi, P.A., Bénard, A., Wille, M., Vroon, P.Z., Arculus, R.J. (2015) Redox-variability and controls in subduction zones from an iron-isotope perspective. Earth and Planetary Science Letters 432, 142–151.
). For example, both the oxidation state and isotope signature of mantle wedge peridotites are likely to be influenced by existing heterogeneities prior to subduction (Williams et al., 2004Williams, H.M., McCammon, C.A., Peslier, A.H., Halliday, A.N., Teutsch, N., Levasseur, S., Burg, J.P. (2004) Iron isotope fractionation and the oxygen fugacity of the mantle. Science 304, 1656–1659.
), as well as by later metasomatism by slab-derived fluids and/or melts (Huang et al., 2019Huang, J., Guo, S., Jin, Q.-Z., Huang, F. (2019) Iron and magnesium isotopic compositions of subduction-zone fluids and implications for arc volcanism. Geochimica et Cosmochimica Acta, doi: 10.1016/j.gca.2019.06.020.
) and/or retrograde processes linked to rock exhumation (Li et al., 2016Li, D.Y., Xiao, Y.L., Li, W.Y., Zhu, X., Williams, H.M., Li, Y.L. (2016) Iron isotopic systematics of UHP eclogites respond to oxidizing fluid during exhumation. Journal of Metamorphic Geology 34, 987–997.
). Despite this, Turner et al. (2018)Turner, S., Williams, H., Piazolo, S., Blichert-Toft, J., Gerdes, M., Adam, J., Liu, X.M., Schaefer, B., Maury, R. (2018) Sub-arc xenolith Fe-Li-Pb isotopes and textures tell tales of their journey through the mantle wedge and crust. Geology 46, 947–950.
recently showed that the unusually light δ56Fe values (down to - 0.31 ‰) of some sub-arc mantle xenoliths were too fractionated to have been caused solely by partial melting. They observed correlations between δ56Fe with 206Pb/204Pb and U/Th, tracers of fluid-related processes, and suggested that sample δ56Fe was modified by reaction with low δ56Fe slab fluids. Similar conclusions were reached by Debret et al. (2018)Debret, B., Bouilhol, P., Pons, M.L., Williams, H. (2018) Carbonate Transfer during the Onset of Slab Devolatilization: New Insights from Fe and Zn Stable Isotopes. Journal of Petrology 59, 1145–1166.
who analysed metamorphic gem olivines in veins from the sub-arc mantle of the Kohistan-Ladakh arc. The vein-forming minerals are interpreted to have crystallised from fluids that migrated through the forearc mantle, and they display light δ56Fe (-0.06 to -0.36 ‰) relative to typical mantle olivine (⁓ +0.01 ‰; Sossi et al., 2015Sossi, P.A., Halverson, G.P., Nebel, O., Eggins, S.M. (2015) Combined separation of Cu, Fe and Zn from rock matrices and improved analytical protocols for stable isotope determination. Geostandards and Geoanalytical Research 39, 129–149.
). These results suggest a strong coupling between slab-derived fluids and the δ56Fe composition of the mantle wedge.To test this idea we carried out a δ56Fe study of serpentinite clasts from the Mariana forearc. The Mariana forearc is the only place in the world where actively metasomatised forearc can be sampled (Savov et al., 2007
Savov, I.P., Ryan, J.G., D’Antonio, M., Fryer, P. (2007) Shallow slab fluid release across and along the Mariana arc-basin system: Insights from geochemistry of serpentinized peridotites from the Mariana fore arc. Journal of Geophysical Research: Solid Earth 112, B09205.
; Fryer et al., 2018Fryer, P., Wheat, C.G., Williams, T., Scientists Expedition, 366 (2018) Mariana Convergent Margin and South Chamorro Seamount. Proceedings of the International Ocean Discovery Program 366.
). It belongs to a non-accretionary subduction system, spreading from near Tokyo (Japan) to beyond Guam (U.S.A.) and involves the subduction of the Mesozoic Pacific plate below the Philippine Sea plate (Fig. 1). Here, fluids released from the subducting slab bring metasomatised forearc rocks to the surface and erupt as mud volcanoes. These rocks provide a direct window into δ56Fe and redox exchanges between the subducted slab and the forearc mantle at various depths. Our study focuses on serpentinised forearc peridotite clasts recovered during the International Oceanic Discovery Program (IODP) Expedition 366 by drilling three mud volcanoes: Yinazao, Fantangisña and Asùt Tesoru, located at increasing distance from the trench (Table S-1, Fig. 1).
Figure 1 Distribution of δ56Fe values in forearc serpentinites. (a) Bathymetry map of the Mariana subduction system showing the locations of the mud volcanoes drilled during IODP Expedition 366 and the ODP Leg 195. Hole locations are indicated in red circles. (b) Iron isotope compositions of bulk ultramafic clasts from the Mariana forearc, organised according to the distance from the trench. DM: Depleted Mantle (Craddock et al., 2013
Craddock, P.R., Warren, J.M., Dauphas, N. (2013) Abyssal peridotites reveal the near-chondritic Fe isotopic composition of the Earth. Earth and Planetary Science Letters 365, 63–76.
).top
Sample Selection
The studied clasts have been petrographically and geochemically characterised by Debret et al. (2019)
Debret, B. et al. (2019) Shallow forearc mantle dynamics and geochemistry: New insights from the IODP expedition 366. Lithos 326–327, 230–245.
and Fryer et al. (2018)Fryer, P., Wheat, C.G., Williams, T., Scientists Expedition, 366 (2018) Mariana Convergent Margin and South Chamorro Seamount. Proceedings of the International Ocean Discovery Program 366.
. They provide a record of deep forearc mantle serpentinisation by slab-derived fluids. Lizardite-bearing serpentinites (Liz-serpentinites) display variable degrees of serpentinisation (from 30 to 100 %) and are primarily composed of low temperature brown serpentine (i.e. lizardite and/or chrysotile) and Fe-rich brucite assemblages forming mesh and bastite textures after olivine and orthopyroxene, respectively. These samples represent the first stage of forearc mantle wedge hydration at low temperature (180-230 °C, based on oxygen isotope estimates) and shallow depths (<13 km; Debret et al., 2019Debret, B. et al. (2019) Shallow forearc mantle dynamics and geochemistry: New insights from the IODP expedition 366. Lithos 326–327, 230–245.
). Antigorite-bearing serpentinites (Atg/Liz- and Atg-serpentinites) are highly serpentinised (⁓ 100 %) and show progressive replacement of lizardite-bearing textures (i.e. mesh and bastite) by antigorite, magnetite and Fe-poor brucite. Oxygen isotope measurements show that the recrystallisation of lizardite to antigorite occurs at temperatures ranging from 200-250 °C to 320-410 °C (Debret et al., 2019Debret, B. et al. (2019) Shallow forearc mantle dynamics and geochemistry: New insights from the IODP expedition 366. Lithos 326–327, 230–245.
), consistent with thermodynamic predictions (Evans, 2004Evans, B.W. (2004) The Serpentinite Multisystem Revisited: Chrysotile Is Metastable. International Geology Review 46, 479–506.
) and antigorite crystallisation temperature estimates derived from meta-ophiolite serpentinites (Schwartz et al., 2013Schwartz, S., Guillot, S., Reynard, B., Lafay, R., Debret, B., Nicollet, C., Lanari, P., Auzende, A.L. (2013) Pressure-temperature estimates of the lizardite/antigorite transition in high pressure serpentinites. Lithos 178, 197–210.
). This provides evidence for the formation of these samples during the progressive burial and hydration of the forearc mantle at depths ranging from 13 to 18 km. The recrystallisation of lizardite to antigorite is accompanied by a decrease in Cs, Li and Sr, and an increase in As and Sb concentrations in the bulk clasts, whereas B concentrations are relatively constant (Debret et al., 2019Debret, B. et al. (2019) Shallow forearc mantle dynamics and geochemistry: New insights from the IODP expedition 366. Lithos 326–327, 230–245.
). The forearc wedge also experienced late stage serpentinisation at low temperature (<180 °C) during clast exhumation which is characterised by the crystallisation of “blue” serpentine and sulfides (blue-serpentinite; Debret et al., 2019Debret, B. et al. (2019) Shallow forearc mantle dynamics and geochemistry: New insights from the IODP expedition 366. Lithos 326–327, 230–245.
). Where present, this late serpentinisation stage largely replaces former textures, i.e. serpentine or mantle minerals, and results in a flattening of rare earth element (REE) spectra and an increase in bulk serpentinite Zn concentration. In order to constrain the impact of such late retrogression stages on the Fe redox state and isotope composition of forearc peridotites, we analysed a suite of these blue-serpentinites. Finally, we tested the chemical and Fe-isotope variability of serpentinised clasts along the Mariana forearc by analysing a suite of ultramafic clasts recovered from South Chamorro, one of the most distant mud volcanoes from the trench located at the extreme south of the Mariana subduction, during the Ocean Drilling Program (ODP) Leg 195 (Fig. 1).top
Results
The δ56Fe and FeO methods and data (samples and standards) are provided in the Supplementary Information. The Mariana ultramafic clasts display large variations in Fe3+/ΣFe (0.24 - 0.67) and δ56Fe (-0.26 ± 0.06, 2s.d., to +0.09 ± 0.02 ‰; Table S-2) with a light mean δ56Fe of -0.06 ± 0.03 ‰ relative to that of the depleted mantle, as estimated from abyssal peridotites (+0.025 ± 0.025 ‰; Craddock et al., 2013
Craddock, P.R., Warren, J.M., Dauphas, N. (2013) Abyssal peridotites reveal the near-chondritic Fe isotopic composition of the Earth. Earth and Planetary Science Letters 365, 63–76.
). No systematic variations in Fe3+/ΣFe and δ56Fe values were observed with increasing distance to the trench or mud volcano location (Fig. 1). In contrast, there is a progressive increase in Fe3+/ΣFe and decrease in δ56Fe from Liz- serpentinites (Fe3+/ΣFe = 0.24 - 0.37; δ56Fe = -0.02 ± 0.12 ‰) to Atg/Liz- (Fe3+/ΣFe = 0.33 - 0.59; δ56Fe = -0.07 ± 0.09 ‰) and Atg- (Fe3+/ΣFe = 0.51 - 0.67; δ56Fe = -0.12 ± 0.07 ‰) serpentinites. These variations are broadly correlated with increasing bulk rock fluid-mobile element (FME) concentrations (Fig. 2). In contrast, blue-serpentinites display a large range of Fe3+/ƩFe (0.33 - 0.77) with depleted mantle-like δ56Fe (-0.01 ± 0.03 ‰) and correlations with FME concentrations are absent (Table S-2, Fig. S-1a-c).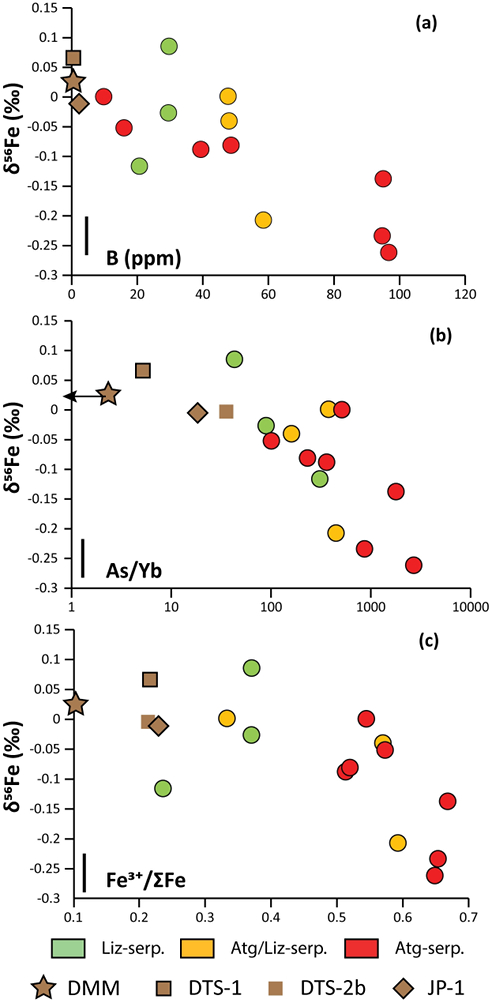
Figure 2 Plots of δ56Fe versus (a) B concentrations, (b) As/Yb and (c) Fe3+/ΣFe ratios in Mariana ultramafic clasts. The black bar represents the FeCl standard reproducibility during analyses. DTS-1, DTS-2b, JP-1: dunite-like reference materials.
top
Discussion
The low δ56Fe signatures of antigorite-bearing clasts agree with our prediction that isotopically light Fe is mobilised in slab fluids (e.g., Debret et al., 2016
Debret, B., Millet, M.-A., Pons, M.-L., Bouilhol, P., Inglis, E., Williams, H. (2016) Isotopic evidence for iron mobility during subduction. Geology 44, 215–218.
, 2018Debret, B., Bouilhol, P., Pons, M.L., Williams, H. (2018) Carbonate Transfer during the Onset of Slab Devolatilization: New Insights from Fe and Zn Stable Isotopes. Journal of Petrology 59, 1145–1166.
). However, before assigning all Fe isotope variability to fluids, several processes must be considered: (1) pre-existing Fe isotope mantle heterogeneities prior to the forearc serpentinisation and (2) the mobility of Fe during late serpentinisation stages accompanying clast exhumation (e.g., blue-serpentine crystallisation). The influence of kinetic effects, related to the preferential mobility of isotopically light species during fluid/rock interactions or diffusive processes, can be ruled out as the direction of diffusive transport from solid (Fe-rich) to fluid (Fe-poor) would result in enrichment in isotopically heavy Fe in the solid, which would generate positive covariations between δ56Fe and Fe3+/ΣFe in the studied samples, which are not observed (Fig. 2c).Forearc mantle wedge peridotites are former sub-arc peridotites that underwent extensive partial melting before being dragged into the forearc by mantle convection (Parkinson and Pearce, 1998
Parkinson, I.J., Pearce, J.A. (1998) Peridotites from the Izu-Bonin-Mariana forearc (ODP Leg 125): evidence for mantle melting and melt-mantle interaction in a supra-subduction zone setting. Journal of Petrology 39, 1577–1618.
). In serpentinised peridotites, protolith fertility is better assessed by Al2O3/SiO2 ratio rather than Al2O3 contents (Niu, 2004Niu, Y. (2004) Bulk-rock major and trace element compositions of abyssal peridotites: Implications for mantle melting, melt extraction and post-melting processes beneath Mid-Ocean ridges. Journal of Petrology 45, 2423–2458.
). The Mariana ultramafic clasts display low Al2O3/SiO2 (<0.03) consistent with a refractory protolith, i.e. dunite or pyroxene-poor harzburgite. However, the studied samples also display a large range in δ56Fe relative to dunite-like reference materials (Table S-2) and no systematic variation between δ56Fe and Al2O3/SiO2 ratio are observed (Fig. S-2a). Similarly, previous studies have shown that high temperature metasomatic events (e.g., melt percolation) can lead to an enrichment in isotopically light Fe (Poitrasson et al., 2013Poitrasson, F., Delpech, G., Gregoire, M. (2013) On the iron isotope heterogeneity of lithospheric mantle xenoliths : implications for mantle metasomatism , the origin of basalts and the iron isotope composition of the Earth. Contributions to Mineralogy and Petrology 165, 1243–1258.
). However, such processes also generate an increase in FeO and correlation between δ56Fe and XMg (= Mg / [Mg + Fe]) are not observed in the studied samples (Fig. S-2b). This shows that the light δ56Fe of Mariana clasts are not controlled by pre-existing protolith heterogeneities and must have been generated by the mobilisation of Fe in fluids.Late serpentinisation stages that overprint high temperature paragenesis are associated with exhumation of clasts and are characterised by blue-serpentine crystallisation and changes in trace element chemistry (Debret et al., 2019
Debret, B. et al. (2019) Shallow forearc mantle dynamics and geochemistry: New insights from the IODP expedition 366. Lithos 326–327, 230–245.
). However, despite strong enrichments in Zn, blue-serpentinites display similar δ56Fe (from -0.07 ‰ to +0.05 ‰) to depleted mantle, dunite reference materials and abyssal peridotites (Fig. S-3). Similarly, no correlation between δ56Fe and indices of late processes, for example, seafloor carbonation (e.g., Sr/Yb) and/or blue-serpentine (e.g., Zn) crystallisation, are observed (Fig. S-3). This suggests that the Mariana forearc clasts preserved δ56Fe values acquired prior to exhumation. The light δ56Fe of our samples must therefore relate either to the loss or the addition of Fe during the serpentinisation of the forearc mantle at depth.It is well established that forearc mantle serpentinisation by slab-derived fluids is accompanied with an increase in bulk rock FME concentrations (e.g., Savov et al., 2007
Savov, I.P., Ryan, J.G., D’Antonio, M., Fryer, P. (2007) Shallow slab fluid release across and along the Mariana arc-basin system: Insights from geochemistry of serpentinized peridotites from the Mariana fore arc. Journal of Geophysical Research: Solid Earth 112, B09205.
). This enrichment has been attributed to the influx of slab-derived fluids in the forearc mantle at intermediate temperatures (200-600 °C). In Figure 2a,b, the prograde Mariana ultramafic clasts (Liz-, Atg/Liz- and Atg-serpentinites) form a step array that extends from mantle-like to low δ56Fe values (-0.26 ‰); this is accompanied with an increase in FME concentrations. In contrast, no correlation between δ56Fe and FME concentrations is observed in retrograde blue-serpentinites or abyssal serpentinites (Fig. S-1a,b). The light δ56Fe of the studied samples therefore must reflect the addition of isotopically light Fe by slab-derived fluids. Furthermore, this decrease in δ56Fe is associated with an increase in Fe3+/ΣFe (Fig. 2c), which is opposed to isotope theory predicting that isotopically heavy Fe will be concentrated into Fe3+-bearing phases rather than Fe2+-bearing phases (Polyakov and Mineev, 2000Polyakov, V.B., Mineev, S.D. (2000) The use of Mossbauer spectroscopy in stable isotope geochemistry. Geochimica et Cosmochimica Acta 64, 849–865.
). This observation thus rules out the direct transfer of Fe3+ by slab-derived fluids. Instead, it suggests a concomitant transfer of isotopically light Fe2+ complexed with an oxidising component (e.g., sulfate or carbonate) in slab-derived fluids. Both sulfate or carbonate complexes have the potential to modify both the Fe isotope signature and oxidation state of forearc serpentinites, despite the relatively low solubility of Fe in fluids (Debret et al., 2018Debret, B., Bouilhol, P., Pons, M.L., Williams, H. (2018) Carbonate Transfer during the Onset of Slab Devolatilization: New Insights from Fe and Zn Stable Isotopes. Journal of Petrology 59, 1145–1166.
). Although chlorine complexes (e.g., Fe(II)Cl2), also preferentially complex light Fe, they are inefficient oxidisers of Fe, and thus an unlikely means of generating the observed relationships between δ56Fe and Fe3+/ΣFe (Fig. 2c).Differences in the stability fields of hydrous, carbon- and sulfur-bearing minerals mean that the compositions of fluid liberated from the subducting slab change progressively with depth. Because of the pronounced differences in δ56Fe between the Liz- and Atg-serpentinite clasts, it is important to identify the reactions responsible for the transfer of isotopically light and oxidised Fe to the mantle wedge. The serpentinisation of mantle peridotites through their interaction with water is commonly associated with the oxidation of ferrous Fe, leading to a progressive increase of Fe3+/ΣFe in the bulk rock and H2 production in the fluid phase through water reduction (Fig. 3):
Eq. 1
3 FeO + H2O(aq) = Fe3O4 + H2(aq)
This process is expected to be efficient at the relatively low temperature of forearc serpentinisation (i.e. lower than 700 °C; Frost and Ballhaus, 1998
Frost, B.R., Ballhaus, C. (1998) Comment on ‘“Constraints on the origin of the oxidation state of mantle overlying subduction zones : An example from Simcoe , Washington , USA”’. Geochimica et Cosmochimica Acta 62, 329–331.
). It can explain why the Mariana ultramafic clasts have high Fe3+/ΣFe ratios (0.24 - 0.67) relative to mantle peridotites (⁓ 0.1; Canil et al., 1994Canil, D., O’Neill, H.S.C., Pearson, D.G., Rudnick, R.L., McDonough, W.F., Carswell, D.A. (1994) Ferric iron in peridotites and mantle oxidation states. Earth and Planetary Science Letters 123, 205–220.
). However, no obvious correlations between bulk rock water content and Fe redox state or between bulk rock water content and δ56Fe are observed (Fig. S-4). Light δ56Fe are mainly observed in antigorite-bearing samples while lizardite-bearing serpentinite displays depleted mantle-like values (Fig. 2). This suggests a marked change in the composition of slab-derived fluids with depth, where the composition of fluids derived at greater depths preferentially mobilise Fe relative to fluids released at shallower levels. Fluids released during the early (shallow) stage of slab devolatilisation (<13 km depth) are likely dominated by diagenetic processes and opal dehydration, whereas later (deeper) processes can include decarbonation and clay mineral decomposition reactions (Bebout, 2013Bebout, G.E. (2013) Chemical and Isotopic Cycling in Subduction Zones. Treatise on Geochemistry: Second Edition 4, 703–747, doi: 10.1016/B978-0-08-095975-7.00322-3.
and references therein). Arsenic, Sb and light REE are preferentially enriched in fluids derived from sediment or metabasite decarbonation reactions (Bebout, 2013Bebout, G.E. (2013) Chemical and Isotopic Cycling in Subduction Zones. Treatise on Geochemistry: Second Edition 4, 703–747, doi: 10.1016/B978-0-08-095975-7.00322-3.
). The correlation between As/Yb and δ56Fe in ultramafic clasts (Fig. 2b) may therefore indicate light δ56Fe is transferred to the mantle wedge by carbonate complexes. The transfer of redox sensitive elements, such as carbon, can modify the redox state of the forearc mantle according to the reaction (Fig. 3):Eq. 2
2FeCO3(aq) + 6FeO = 4Fe2O3 + 2C
The exact form of carbon in this equation is speculative. However, the increase of Fe3+/ΣFe in the ultramafic clasts is consistent with the observed increase of complex abiotic hydrocarbon phases in pore fluids from the most distal mud volcanoes (Eickenbusch et al., 2019
Eickenbusch, P., Takai, K., Sissman, O., Suzuki, S., Menzies, C. (2019) Origin of Short-Chain Organic Acids in Serpentinite Mud Volcanoes of the Mariana Convergent Margin. Frontiers in Microbiology 10, 1–21.
), suggesting that carbon may be the relevant oxidising agent in this setting. Furthermore, several studies have speculated the sulfate anions, derived from hydrous breakdown of sulfides, can complex with Fe and facilitate its transfer to the mantle wedge, for example:Eq. 3
FeSO4(aq) + 12FeO = 4Fe3O4 + FeS
Reactions of this type are consistent with several studies which have shown that progressive prograde metamorphism of the subducting slab is associated with the release of sulfate- and/or carbonate-bearing fluids that mobilise light δ56Fe (Debret et al., 2016
Debret, B., Millet, M.-A., Pons, M.-L., Bouilhol, P., Inglis, E., Williams, H. (2016) Isotopic evidence for iron mobility during subduction. Geology 44, 215–218.
, 2018Debret, B., Bouilhol, P., Pons, M.L., Williams, H. (2018) Carbonate Transfer during the Onset of Slab Devolatilization: New Insights from Fe and Zn Stable Isotopes. Journal of Petrology 59, 1145–1166.
). The percolation of such fluids into the forearc can drive Fe oxidation and therefore explain the negative correlation between δ56Fe and Fe3+/ΣFe (Fig. 2c), despite the absence of a correlation with bulk rock water contents (Fig. S-4).The oxygen fugacity (fO2) of slab-derived fluids is believed to increase with depth (e.g., Debret et al., 2016
Debret, B., Millet, M.-A., Pons, M.-L., Bouilhol, P., Inglis, E., Williams, H. (2016) Isotopic evidence for iron mobility during subduction. Geology 44, 215–218.
), with high fO2 conditions (e.g., magnetite/hematite buffer) occurring at eclogite facies conditions (⁓ 80 km depth). Thus, during shallow forearc hydration (13-18 km depth), sulfur is expected to be equilibrated at modest fO2 in slab-derived fluids and mainly transferred under reduced form (e.g., H2S), while carbon will be equilibrated as an oxidised form (CO2). It is thus plausible to consider that carbonate could be the main ligand transferring isotopically light Fe from the slab to the shallow mantle wedge. This is consistent with the high carbon concentrations measured in Mariana pore fluids from the most distant mud volcanoes (Fryer et al., 2018Fryer, P., Wheat, C.G., Williams, T., Scientists Expedition, 366 (2018) Mariana Convergent Margin and South Chamorro Seamount. Proceedings of the International Ocean Discovery Program 366.
). Together, the correlation between Fe isotopes, FME concentrations and ratios, and iron redox state demonstrate a critical role for slab-derived fluids in regulating the redox state of the mantle wedge.
Figure 3 Cartoon illustrating redox and isotopic transfers between the slab and the forearc mantle wedge.
top
Acknowledgements
This research used samples and data provided by the IODP and ODP. We are grateful to the crew of the D/V JOIDES Resolution and to the science party of IODP Expedition 366. Funding for this research was provided by a NERC Moratorium Award (NE/P020860/1, to BD and HMW), the F.R.S.-F.N.R.S. (BD), the Fondation Wiener Anspach (RG97553, NM, BD and HMW), the ERC Consolidator Grant (306655, HMW), a NERC Critical Minerals grant (NE/M010848/1, HMW) and a Schlanger Fellowship Award (IS). We thank K. Evans and F. Poitrasson for critical comments on earlier version of this article and careful editorial handling by M. Boyet. This study contributes to the IdEx Université de Paris ANR-18-IDEX-0001.
Editor: Maud Boyet
top
References
Bebout, G.E. (2013) Chemical and Isotopic Cycling in Subduction Zones. Treatise on Geochemistry: Second Edition 4, 703–747, doi: 10.1016/B978-0-08-095975-7.00322-3.

Fluids released during the early (shallow) stage of slab devolatilisation (<13 km depth) are likely dominated by diagenetic processes and opal dehydration, whereas later (deeper) processes can include decarbonation and clay mineral decomposition reactions (Bebout, 2013 and references therein).
View in article
Arsenic, Sb and light REE are preferentially enriched in fluids derived from sediment or metabasite decarbonation reactions (Bebout, 2013).
View in article
Canil, D., O’Neill, H.S.C., Pearson, D.G., Rudnick, R.L., McDonough, W.F., Carswell, D.A. (1994) Ferric iron in peridotites and mantle oxidation states. Earth and Planetary Science Letters 123, 205–220.

It can explain why the Mariana ultramafic clasts have high Fe3+/ΣFe ratios (0.24 - 0.67) relative to mantle peridotites (⁓ 0.1; Canil et al., 1994).
View in article
Craddock, P.R., Warren, J.M., Dauphas, N. (2013) Abyssal peridotites reveal the near-chondritic Fe isotopic composition of the Earth. Earth and Planetary Science Letters 365, 63–76.

Figure 1 [...] DM: Depleted Mantle (Craddock et al., 2013).
View in article
The Mariana ultramafic clasts display large variations in Fe3+/ΣFe (0.24 - 0.67) and δ56Fe (-0.26 ± 0.06, 2s.d., to +0.09 ± 0.02 ‰; Table S-2) with a light mean δ56Fe of -0.06 ± 0.03 ‰ relative to that of the depleted mantle, as estimated from abyssal peridotites (+0.025 ± 0.025 ‰; Craddock et al., 2013).
View in article
Debret, B., Millet, M.-A., Pons, M.-L., Bouilhol, P., Inglis, E., Williams, H. (2016) Isotopic evidence for iron mobility during subduction. Geology 44, 215–218.

Recent iron isotope studies (δ56Fe) have shown that iron, which was previously considered to be insoluble, can be mobilised in slab-derived fluids by complexation with chloride and/or oxidising ligands (e.g., Fe(II)-CO3 or Fe(II)-SO4; Debret et al., 2016, 2018).
View in article
Importantly, these complexes preferentially concentrate light (low) δ56Fe, driving stable isotope fractionation (Debret et al., 2016, 2018).
View in article
The low δ56Fe signatures of antigorite-bearing clasts agree with our prediction that isotopically light Fe is mobilised in slab fluids (e.g., Debret et al., 2016, 2018).
View in article
Reactions of this type are consistent with several studies which have shown that progressive prograde metamorphism of the subducting slab is associated with the release of sulfate- and/or carbonate-bearing fluids that mobilise light δ56Fe (Debret et al., 2016, 2018).
View in article
The oxygen fugacity (fO2) of slab-derived fluids is believed to increase with depth (e.g., Debret et al., 2016), with high fO2 conditions (e.g., magnetite/hematite buffer) occurring at eclogite facies conditions (⁓ 80 km depth).
View in article
Debret, B., Bouilhol, P., Pons, M.L., Williams, H. (2018) Carbonate Transfer during the Onset of Slab Devolatilization: New Insights from Fe and Zn Stable Isotopes. Journal of Petrology 59, 1145–1166.

Recent iron isotope studies (δ56Fe) have shown that iron, which was previously considered to be insoluble, can be mobilised in slab-derived fluids by complexation with chloride and/or oxidising ligands (e.g., Fe(II)-CO3 or Fe(II)-SO4; Debret et al., 2016, 2018).
View in article
Importantly, these complexes preferentially concentrate light (low) δ56Fe, driving stable isotope fractionation (Debret et al., 2016, 2018).
View in article
Similar conclusions were reached by Debret et al. (2018) who analysed metamorphic gem olivines in veins from the sub-arc mantle of the Kohistan-Ladakh arc.
View in article
The low δ56Fe signatures of antigorite-bearing clasts agree with our prediction that isotopically light Fe is mobilised in slab fluids (e.g., Debret et al., 2016, 2018).
View in article
Both sulfate or carbonate complexes have the potential to modify both the Fe isotope signature and oxidation state of forearc serpentinites, despite the relatively low solubility of Fe in fluids (Debret et al., 2018).
View in article
Reactions of this type are consistent with several studies which have shown that progressive prograde metamorphism of the subducting slab is associated with the release of sulfate- and/or carbonate-bearing fluids that mobilise light δ56Fe (Debret et al., 2016, 2018).
View in article
Debret, B. et al. (2019) Shallow forearc mantle dynamics and geochemistry: New insights from the IODP expedition 366. Lithos 326–327, 230–245.

The studied clasts have been petrographically and geochemically characterised by Debret et al. (2019) and Fryer et al. (2018).
View in article
These samples represent the first stage of forearc mantle wedge hydration at low temperature (180-230 °C, based on oxygen isotope estimates) and shallow depths (<13 km; Debret et al., 2019).
View in article
Oxygen isotope measurements show that the recrystallisation of lizardite to antigorite occurs at temperatures ranging from 200-250 °C to 320-410 °C (Debret et al., 2019), consistent with thermodynamic predictions (Evans, 2004) and antigorite crystallisation temperature estimates derived from meta-ophiolite serpentinites (Schwartz et al., 2013).
View in article
The recrystallisation of lizardite to antigorite is accompanied by a decrease in Cs, Li and Sr, and an increase in As and Sb concentrations in the bulk clasts, whereas B concentrations are relatively constant (Debret et al., 2019).
View in article
The forearc wedge also experienced late stage serpentinisation at low temperature (<180 °C) during clast exhumation which is characterised by the crystallisation of “blue” serpentine and sulfides (blue-serpentinite; Debret et al., 2019).
View in article
Late serpentinisation stages that overprint high temperature paragenesis are associated with exhumation of clasts and are characterised by blue-serpentine crystallisation and changes in trace element chemistry (Debret et al., 2019).
View in article
Eickenbusch, P., Takai, K., Sissman, O., Suzuki, S., Menzies, C. (2019) Origin of Short-Chain Organic Acids in Serpentinite Mud Volcanoes of the Mariana Convergent Margin. Frontiers in Microbiology 10, 1–21.

However, the increase of Fe3+/ΣFe in the ultramafic clasts is consistent with the observed increase of complex abiotic hydrocarbon phases in pore fluids from the most distal mud volcanoes (Eickenbusch et al., 2019), suggesting that carbon may be the relevant oxidising agent in this setting.
View in article
Evans, B.W. (2004) The Serpentinite Multisystem Revisited: Chrysotile Is Metastable. International Geology Review 46, 479–506.

Oxygen isotope measurements show that the recrystallisation of lizardite to antigorite occurs at temperatures ranging from 200-250 °C to 320-410 °C (Debret et al., 2019), consistent with thermodynamic predictions (Evans, 2004) and antigorite crystallisation temperature estimates derived from meta-ophiolite serpentinites (Schwartz et al., 2013).
View in article
Evans, K.A. (2012) The redox budget of subduction zones. Earth-Science Reviews 113, 11–32.

Subduction zones regulate chemical exchange between the Earth’s surface and interior, modulating the long term cycling of redox sensitive elements such as iron, carbon and sulfur (Evans, 2012).
View in article
Frost, B.R., Ballhaus, C. (1998) Comment on ‘“Constraints on the origin of the oxidation state of mantle overlying subduction zones : An example from Simcoe , Washington , USA”’. Geochimica et Cosmochimica Acta 62, 329–331.

This process is expected to be efficient at the relatively low temperature of forearc serpentinisation (i.e. lower than 700 °C; Frost and Ballhaus, 1998).
View in article
Fryer, P., Wheat, C.G., Williams, T., Scientists Expedition, 366 (2018) Mariana Convergent Margin and South Chamorro Seamount. Proceedings of the International Ocean Discovery Program 366.

The Mariana forearc is the only place in the world where actively metasomatised forearc can be sampled (Savov et al., 2007; Fryer et al., 2018).
View in article The studied clasts have been petrographically and geochemically characterised by Debret et al. (2019) and Fryer et al. (2018).
View in article
This is consistent with the high carbon concentrations measured in Mariana pore fluids from the most distant mud volcanoes (Fryer et al., 2018).
View in article
Huang, J., Guo, S., Jin, Q.-Z., Huang, F. (2019) Iron and magnesium isotopic compositions of subduction-zone fluids and implications for arc volcanism. Geochimica et Cosmochimica Acta, doi: 10.1016/j.gca.2019.06.020.

For example, both the oxidation state and isotope signature of mantle wedge peridotites are likely to be influenced by existing heterogeneities prior to subduction (Williams et al., 2004), as well as by later metasomatism by slab-derived fluids and/or melts (Huang et al., 2019) and/or retrograde processes linked to rock exhumation (Li et al., 2016).
View in article
Kelley, K.A., Cottrell, E. (2009) Water and the oxidation state of subduction zone magmas. Science 325, 605–607.

These findings concur with the high Fe3+/ΣFe (Kelley and Cottrell, 2009) and light δ56Fe (Nebel et al., 2015) of arc lavas relative to mid-ocean ridge basalts, although the origin of light δ56Fe in arc lavas remains an active research frontier.
View in article
Li, D.Y., Xiao, Y.L., Li, W.Y., Zhu, X., Williams, H.M., Li, Y.L. (2016) Iron isotopic systematics of UHP eclogites respond to oxidizing fluid during exhumation. Journal of Metamorphic Geology 34, 987–997.

For example, both the oxidation state and isotope signature of mantle wedge peridotites are likely to be influenced by existing heterogeneities prior to subduction (Williams et al., 2004), as well as by later metasomatism by slab-derived fluids and/or melts (Huang et al., 2019) and/or retrograde processes linked to rock exhumation (Li et al., 2016).
View in article
Nebel, O., Sossi, P.A., Bénard, A., Wille, M., Vroon, P.Z., Arculus, R.J. (2015) Redox-variability and controls in subduction zones from an iron-isotope perspective. Earth and Planetary Science Letters 432, 142–151.

These findings concur with the high Fe3+/ΣFe (Kelley and Cottrell, 2009) and light δ56Fe (Nebel et al., 2015) of arc lavas relative to mid-ocean ridge basalts, although the origin of light δ56Fe in arc lavas remains an active research frontier.
View in article
The transfer of redox sensitive elements across subduction zones involves complex and multi-stage processes (Nebel et al., 2015).
View in article
Niu, Y. (2004) Bulk-rock major and trace element compositions of abyssal peridotites: Implications for mantle melting, melt extraction and post-melting processes beneath Mid-Ocean ridges. Journal of Petrology 45, 2423–2458.

In serpentinised peridotites, protolith fertility is better assessed by Al2O3/SiO2 ratio rather than Al2O3 contents (Niu, 2004).
View in article
Parkinson, I.J., Pearce, J.A. (1998) Peridotites from the Izu-Bonin-Mariana forearc (ODP Leg 125): evidence for mantle melting and melt-mantle interaction in a supra-subduction zone setting. Journal of Petrology 39, 1577–1618.

Forearc mantle wedge peridotites are former sub-arc peridotites that underwent extensive partial melting before being dragged into the forearc by mantle convection (Parkinson and Pearce, 1998).
View in article
Poitrasson, F., Delpech, G., Gregoire, M. (2013) On the iron isotope heterogeneity of lithospheric mantle xenoliths : implications for mantle metasomatism , the origin of basalts and the iron isotope composition of the Earth. Contributions to Mineralogy and Petrology 165, 1243–1258.

Similarly, previous studies have shown that high temperature metasomatic events (e.g., melt percolation) can lead to an enrichment in isotopically light Fe (Poitrasson et al., 2013).
View in article
Polyakov, V.B., Mineev, S.D. (2000) The use of Mossbauer spectroscopy in stable isotope geochemistry. Geochimica et Cosmochimica Acta 64, 849–865.

Furthermore, this decrease in δ56Fe is associated with an increase in Fe3+/ΣFe (Fig. 2c), which is opposed to isotope theory predicting that isotopically heavy Fe will be concentrated into Fe3+-bearing phases rather than Fe2+-bearing phases (Polyakov and Mineev, 2000).
View in article
Savov, I.P., Ryan, J.G., D’Antonio, M., Fryer, P. (2007) Shallow slab fluid release across and along the Mariana arc-basin system: Insights from geochemistry of serpentinized peridotites from the Mariana fore arc. Journal of Geophysical Research: Solid Earth 112, B09205.

The Mariana forearc is the only place in the world where actively metasomatised forearc can be sampled (Savov et al., 2007; Fryer et al., 2018).
View in article
It is well established that forearc mantle serpentinisation by slab-derived fluids is accompanied with an increase in bulk rock FME concentrations (e.g., Savov et al., 2007).
View in article
Schwartz, S., Guillot, S., Reynard, B., Lafay, R., Debret, B., Nicollet, C., Lanari, P., Auzende, A.L. (2013) Pressure-temperature estimates of the lizardite/antigorite transition in high pressure serpentinites. Lithos 178, 197–210.

Oxygen isotope measurements show that the recrystallisation of lizardite to antigorite occurs at temperatures ranging from 200-250 °C to 320-410 °C (Debret et al., 2019), consistent with thermodynamic predictions (Evans, 2004) and antigorite crystallisation temperature estimates derived from meta-ophiolite serpentinites (Schwartz et al., 2013).
View in article
Sossi, P.A., Halverson, G.P., Nebel, O., Eggins, S.M. (2015) Combined separation of Cu, Fe and Zn from rock matrices and improved analytical protocols for stable isotope determination. Geostandards and Geoanalytical Research 39, 129–149.

The vein-forming minerals are interpreted to have crystallised from fluids that migrated through the forearc mantle, and they display light δ56Fe (-0.06 to -0.36 ‰) relative to typical mantle olivine (⁓ +0.01 ‰; Sossi et al., 2015).
View in article
Turner, S., Williams, H., Piazolo, S., Blichert-Toft, J., Gerdes, M., Adam, J., Liu, X.M., Schaefer, B., Maury, R. (2018) Sub-arc xenolith Fe-Li-Pb isotopes and textures tell tales of their journey through the mantle wedge and crust. Geology 46, 947–950.

Despite this, Turner et al. (2018) recently showed that the unusually light δ56Fe values (down to - 0.31 ‰) of some sub-arc mantle xenoliths were too fractionated to have been caused solely by partial melting.
View in article
Williams, H.M., McCammon, C.A., Peslier, A.H., Halliday, A.N., Teutsch, N., Levasseur, S., Burg, J.P. (2004) Iron isotope fractionation and the oxygen fugacity of the mantle. Science 304, 1656–1659.

For example, both the oxidation state and isotope signature of mantle wedge peridotites are likely to be influenced by existing heterogeneities prior to subduction (Williams et al., 2004), as well as by later metasomatism by slab-derived fluids and/or melts (Huang et al., 2019) and/or retrograde processes linked to rock exhumation (Li et al., 2016).
View in article
top
Supplementary Information
The Supplementary Information includes:
- Method
- Tables S-1 and S-2
- Figures S-1 to S-4
- Supplementary Information References
Download the Supplementary Information (PDF).
Figures and Tables
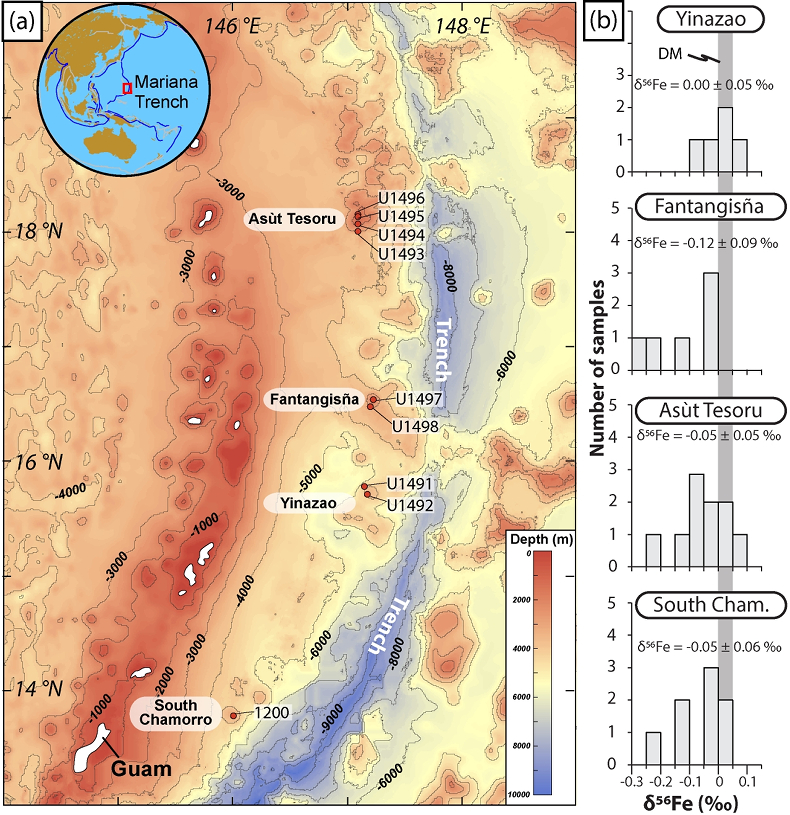
Figure 1 Distribution of δ56Fe values in forearc serpentinites. (a) Bathymetry map of the Mariana subduction system showing the locations of the mud volcanoes drilled during IODP Expedition 366 and the ODP Leg 195. Hole locations are indicated in red circles. (b) Iron isotope compositions of bulk ultramafic clasts from the Mariana forearc, organised according to the distance from the trench. DM: Depleted Mantle (Craddock et al., 2013
Craddock, P.R., Warren, J.M., Dauphas, N. (2013) Abyssal peridotites reveal the near-chondritic Fe isotopic composition of the Earth. Earth and Planetary Science Letters 365, 63–76.
).
Figure 2 Plots of δ56Fe versus (a) B concentrations, (b) As/Yb and (c) Fe3+/ΣFe ratios in Mariana ultramafic clasts. The black bar represents the FeCl standard reproducibility during analyses. DTS-1, DTS-2b, JP-1: dunite-like reference materials.
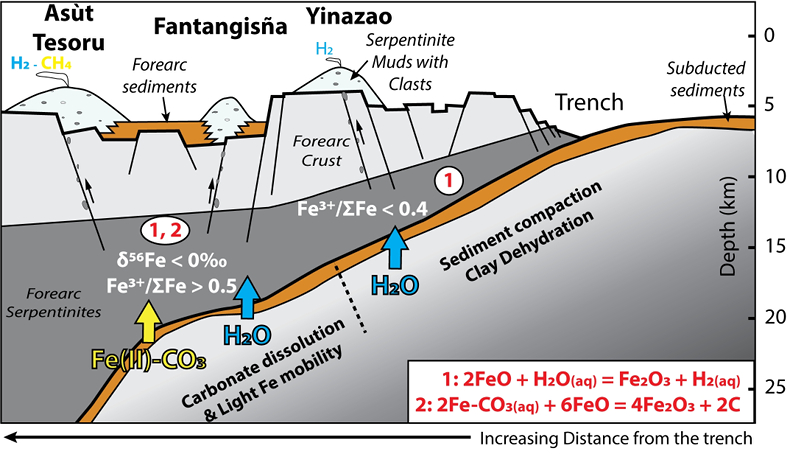
Figure 3 Cartoon illustrating redox and isotopic transfers between the slab and the forearc mantle wedge.