Microbial nitrogen cycle enhanced by continental input recorded in the Gunflint Formation
Affiliations | Corresponding Author | Cite as | Funding information- Share this article
Article views:8,543Cumulative count of HTML views and PDF downloads.
- Download Citation
- Rights & Permissions
top
Abstract

Figures and Tables
![]() Figure 1 Results of the stepwise combustion analyses of 13 kerogen samples from the Gunflint Formation (samples #0704 and #0708 are cited from Ishida et al., 2012). Nitrogen concentrations (white bars) and d15N values (black squares) are shown. The average d15N values and errors of both the lower-temperature and the higher-temperature fractions are shown as light green and dark green bars, respectively. Original data are summarised in Table S-2. | ![]() Table 1 Summary of light and trace element concentrations and the isotope compositions of bulk rock and kerogen samples. | ![]() Figure 2 Correlation diagrams between the Pr/Sm ratios and (a) the d15NLT values and (b) the d15NHT values, respectively. A positive correlation is observed between the Pr/Sm ratios and the d15NLT values (R2 = 0.60, excluding #0714), whereas no correlation is observed between the d15NHT values. | ![]() Figure 3 Correlation diagrams between the Pr/Sm ratios and (a) the Eu/Eu* ratios, (b) TiO2, and (c) Zr concentrations. The Eu/Eu* is defined as Eu/0.5(Gd+Sm). Grey square areas indicate the range of modern ocean values. Dashed and solid arrows indicate the directions of hydrothermal fluid and crustal contributions, respectively (Bolhar et al., 2005). Positive correlations exist between the Pr/Sm and TiO2 and Zr concentrations. |
Figure 1 | Table 1 | Figure 2 | Figure 3 |
Supplementary Figures and Tables
![]() Figure S-1 Geological map of the Thunder Bay region (modified after Geological map of Thunder Bay district “Map 2065 ATIKOKAN-LAKEHEAD SHEET Geological Compilation Series”). Geological surveys were conducted in five sections: Pass Lake section; Blende Lake North section; Current River section; Terry Fox section; Kakabeka Falls and Highway 590 east section. | ![]() Figure S-2 Thin section images of representative samples. Scale bars in each photo are 1 millimetre unless otherwise noted. (a) Sample #0721: Oolite from Pass Lake section. Fe-rich dolomite covers lithic core concentrically in a quartz matrix. Inserted image: Back-scattered electron (BSE) image of ooid grain. Pyrite is contained in the rim of an ooid grain. Ooid grains contain tiny haematite crystals in the carbonate rims. Scale bar is 200 µm. (b) Sample #0722: BIF iron layer from Pass Lake section. The upper iron-rich layer consists of haematite in a microcrystalline quartz matrix. The lower chert layer consists of microcrystalline quartz. (c) Sample #0704: Sandstone from the Current River section. Sandy grains consist of microcrystalline quartz. Each diameter is approximately 50 to 100 µm. Tiny ferrodolomitic carbonate grains surround the grains. (d) Sample #0709: Organic matter (OM) is observed with detrital lithic fragments, such as quartz and clay minerals. The matrix is carbonate mud. (e) Sample #0713: Phosphorite pebble from the Current River section. The matrix consists of ferrodolomite and detrital quartz. The phosphorite pebbles consist of an apatite matrix, fragments of quartz, clay minerals, OM, and pyrite. Inserted image: Framboidal pyrite found around the phosphorite (white arrow). Both euhedral and framboidal pyrite crystals are observed. Arsenic is present in the framboidal pyrite as a minor component (less than 2 wt. %), but not in the euhedral pyrite. Scale bar is 50 µm. (f) Sample #0715: Finely laminated sedimentary layers with OM. The matrix consists of quartz and chlorite. Carbonate minerals and pyrite are scarce. (g) Sample #0726: Ferrodolomitic layer in BIF from the Terry Fox section. Euhedral dolomite crystals are present in a microcrystalline quartz matrix. Inserted image: BSE image of single ferrodolomite crystal. Outer rim of crystal is richer in iron than the crystal interior. Scale bar is 100 µm. (h) Sample #0726: Chert layer in a BIF sample. Microcrystalline quartz grains with fibrous cement are observed in a quartz matrix. (i) Sample #0725: Black chert from the Terry Fox section (reflected light). OM is present in a chert matrix. Pyrite crystals are observed in the OM layer. (j) Sample #0601: Iron layer from the Kakabeka Falls section. Matrix consists of iron-rich silicate. OM exists as a laminated structure. Minor siderites were observed with no relation to the stratigraphic layer. | ![]() Table S-1 Summary of major and trace element concentrations of bulk rock samples. Eu/Eu* is defined as Eu/0.5(Gd+Sm), Pr/Pr* is defined as Pr/0.5(Ce+Nd), and Ce/Ce* is defined as Ce/0.5(La+Pr). Values are normalised by PAAS reported by Pourmand et al. (2012). | ![]() Figure S-3 Correlation diagrams of geochemical data from the examined samples. Data are sorted based on lithology: blue rhombs = carbonate, red circle = chert, black triangle = shale, and square = BIF (yellow: chert/carbonate, black: shale containing iron layer). (a) Correlation diagram of total organic carbon content (TOC) versus pyrite sulphur content (Spyr). A positive correlation exists, except for two samples that contain higher amounts of sulphur (samples #0721 and #0725). (b) Correlation diagram of TOC versus d13C values for organic matter. No correlation is observed. Correlation diagrams of (c) kerogen C/N ratios vs. d15N values and (d) d13C vs. d15N values. | ![]() Figure S-4 Correlation diagram of N/C and H/C ratios of kerogens. Data are sorted by lithology; legends are the same as Figure S-3. Grey squares are data from Mesoproterozoic to Palaeoproterozoic kerogen samples cited from Schopf and Klein (1992). |
Figure S-1 | Figure S-2 | Table S-1 | Figure S-3 | Figure S-4 |
![]() Table S-2 Summary of the stepwise combustion analyses performed on kerogen (sample IDs #0701, #0703, #0705, #0706, #0709, #0711, #0712, #0713, #0714, #0721, and #0723). | ![]() Figure S-5 An alternative version of Figure 4. The lower- and the higher-temperature fractions are divided depending on the shift of d15N values at approximately 575 °C. The nitrogen concentrations (white bars) and d15N values (black squares) are shown. | ![]() Table S-3 Summary of the d15NLT and d15NHT values of the 13 samples analysed with the stepwise combustion method. ‘d15N (‰): divided by temperature’ refers to the lower- and higher-temperature fractions divided based on the temperature: lower temperature = 500–575 °C, higher temperature = higher than 575 to 1100 °C. ‘d15N (‰): divided by d15N values’ means the data are divided and calculated into two fractions according to the shift in d15N values (refer to Fig. S-5). The average d15N values were calculated based on the weighted average of the released amount of nitrogen. | ![]() Figure S-6 The correlations between the (a) d15NLT values, and (b) the d15NHT values, and the Pr/Sm ratios. Both d15NLT and d15NHT are divided based on the temperatures of the shift of the d15N values that were observed on each sample. The results of calculations are summarised in the Table S-3. The same trends as in Figure 2 were observed: a positive correlation is observed between the Pr/Sm ratios and d15NLT values (R2 = 0.59, excluding #0714), whereas no correlation is observed between the Pr/Sm ratios and d15NHT values. (c) There is no correlation between the d15Nbulk values and the Pr/Sm ratios. | ![]() Figure S-7 Transmitted electron microscopic image of the kerogen extracted from sample #0708. There are at least two types of carbon structures: a highly graphitised portion (white arrow) and folded structures (black arrow). This difference in structure might be responsible for the differences in the nitrogen isotopes. The scale bar is 200 nanometres. Insert: Selected area electron diffraction pattern of the highly graphitised area. |
Table S-2 | Figure S-5 | Table S-3 | Figure S-6 | Figure S-7 |
![]() Table S-4 Summary of REE concentrations of the examined samples normalised by the PAAS values. | ![]() Figure S-8 Correlation diagrams of Al2O3 versus K2O, Fe2O3, and TiO2 in bulk rock and Zr versus V, Th, Sc, Ni, total amount of rare earth elements (total REEs), and P2O5 concentrations. K2O (a) and TiO2 (c) show positive correlations with Al2O3, whereas Fe2O3 (b) does not exhibit a correlation with Al2O3. V (d), Th (e), Sc (f), and Ni (g) have positive correlations with Zr. Total REEs and P2O5 have weak positive correlations. Data below the detection limit were omitted. Data are sorted by lithology; legends are the same as Figure S-3. | ![]() Figure S-9 Diagrams of the rare earth elements and Y in each surveyed section: (a) Pass Lake section, (b) Blende Lake North section, (c) Current River section, (d) Terry Fox section, and (e) Kakabeka Falls section. | ![]() Figure S-10 Correlation diagrams of (a) Pr/Pr* and Ce/Ce* ratios and (b) Ce/Ce* and Eu/Eu* ratios. Pr/Pr* is defined as Pr/0.5(Ce+Nd), and Ce/Ce* is defined as Ce/0.5(La+Pr). A negative correlation exists between them. Data are sorted by lithology; legends are the same as Figure S-3. | ![]() Table S-5 Correlation coefficient values of the Pr/Sm ratios against the major and trace elements concentrations. Elements which have positive correlations with the Pr/Sm ratios are displayed in a thick font. |
Table S-4 | Figure S-8 | Figure S-9 | Figure S-10 | Table S-5 |
top
Introduction
The nitrogen isotope compositions (d15N values) of organic matter (OM) found in ancient sedimentary rocks are used as proxies for reconstructing the microbial nitrogen cycle and the palaeo-oceanic environment (e.g., Beaumont and Robert, 1999
Beaumont, V., Robert, F. (1999) Nitrogen isotope ratios of kerogens in Precambrian cherts: a record of the evolution of atmosphere chemistry? Precambrian Research 96, 63–82.
, Shen et al., 2006Shen, Y., Pinti, D.L., Hashizume, K. (2006) Biogeochemical cycles of sulfur and nitrogen in the Archean ocean and atmosphere. In: Benn, K., Mareschal, J.C., Condie, K. (Eds.) Archean Geodynamics and Environments, Geophyscal Monograph Series 164, 305–320.
; Ader et al., 2016Ader, M., Thomazo, C., Sansjofre, P., Busigny, V., Papineau, D., Laffont, R., Cartigny P., Halverson, G.P. (2016) Interpretation of the nitrogen isotopic composition of Precambrian sedimentary rocks: assumptions and perspectives. Chemical Geology 429, 93–10.
; Stüeken et al., 2016Stüeken, E.E., Kipp, M.A., Koehler, M.C., Buick, R. (2016) The evolution of Earth’s biogeochemical nitrogen cycle. Earth-Science Reviews 160, 220–239.
). OM in sedimentary rock is considered to be a complex mixture of several organisms, however, they are poorly understood. In the stepwise combustion method, components hosted by different carriers, which are intimately mixed in a sample and cannot be separated by other physical methods, can be resolved based on the combustion temperature. A preliminary study was performed using kerogen samples extracted from two rock samples from the Gunflint Formation, indicating temperature-dependent d15N heterogeneities within each kerogen sample (Ishida et al., 2012Ishida, A., Hashizume, K., Kakegawa, T. (2012) Stepwise combustion analyses of distinct nitrogen isotopic compositions on Paleoproterozoic organic matter, Geochemical Journal 46, 249–253.
). In this study, we further examined 11 samples in search of internal d15N heterogeneities in the Gunflint kerogen.The Gunflint Formation, belonging to the Animikie Group, is located on the northwestern shore of Lake Superior, Ontario, Canada (Goodwin, 1956
Goodwin, A.M. (1956) Facies relations in the Gunflint iron formation. Economic Geology 51, 565-595.
). The age of the Gunflint Formation is estimated as 1878 ± 1.3 Ma by U-Pb dating (Fralick et al., 2002Fralick, P., Davis, D.W., Kissin, S.A. (2002) The age of Gunflint Formation, Ontario, Canada: single zircon U-Pb age determinations from reworked volcanic ash. Canadian Journal of Earth Science 39, 1085–1091.
). An evolution model for the Animikie Basin has been proposed by, e.g., Schulz and Cannon (2007)Schulz, K.J., Cannon, W.F. (2007) The Penokean orogeny in the Lake Superior region. Precambrian Research 157, 4–25.
. The tectonic evolution in this region leads to transition from foreland-type volcanism to the back-arc type volcanism, resulting in active supply of detrital material from the continent. In the present study, samples were collected covering the variation representative of shallow to deep lithology. Lithology and whole rock major and trace element geochemistry, which were consistent with previous studies, are summarised in Supplementary Information.top
Stepwise Nitrogen Analysis of Kerogen
The stepwise combustion method discriminates organic nitrogen fractions with different chemical natures. The analytical protocols and performances are described in Ishida et al. (2012)
Ishida, A., Hashizume, K., Kakegawa, T. (2012) Stepwise combustion analyses of distinct nitrogen isotopic compositions on Paleoproterozoic organic matter, Geochemical Journal 46, 249–253.
, and references therein. Kerogen samples were combusted in an oxygen atmosphere with temperatures from 450 ºC to 1100 ºC. No isotope measurement was performed at 450 ºC, where surficial contamination, such as adsorbed nitrogen and/or ammonium ions, dominates. Empirically two distinct nitrogen isotope plateaus appear in kerogen analyses: one appears at temperatures between 475 ºC and 575 ºC (lower temperature fraction: LT-fraction), and the other appears at temperatures more than 575 ºC (higher temperature fraction: HT-fraction) (Ishida et al., 2012Ishida, A., Hashizume, K., Kakegawa, T. (2012) Stepwise combustion analyses of distinct nitrogen isotopic compositions on Paleoproterozoic organic matter, Geochemical Journal 46, 249–253.
). Between 475 ºC and 575 ºC, combustion temperatures were increased in 25 ºC steps to precisely resolve LT- and HT-fractions. Beyond this temperature range, one batch of combustion was performed at 1100 ºC for analyses of the HT-fraction. Besides the stepwise combustion, d15N values of bulk kerogen were also determined.top
Nitrogen Isotope Heterogeneity in Gunflint Kerogen
The d15N values of the bulk kerogen samples, ranging between +2.9 and +8.0 ‰, are consistent with previous reports (e.g., Beaumont and Robert, 1999
Beaumont, V., Robert, F. (1999) Nitrogen isotope ratios of kerogens in Precambrian cherts: a record of the evolution of atmosphere chemistry? Precambrian Research 96, 63–82.
; Godfrey et al., 2013Godfrey, L.V., Poulton, S.W., Bebout, G.E., Fralick, P.W. (2013) Stability of the nitrogen cycle during development of sulfidic water in the redox-stratified late Paleoproterozoic Ocean. Geology 41, 655–658.
). The isotope shift by metamorphism can induce preferential release of 14N from the OM. However, the low metamorphic grade of the Gunflint Formation (below greenschist facies) cannot be responsible for a significant fractionation, up to 5 ‰ (e.g., Haendal et al., 1986Haendal, D., Muhle, K., Nitzsche, H., Stiehl, G., Wand, U. (1986) Isotopic variations of the fixed nitrogen in metamorphic rocks. Geochimica et Cosmochimica Acta 50, 749–758.
, Ader et al., 2016Ader, M., Thomazo, C., Sansjofre, P., Busigny, V., Papineau, D., Laffont, R., Cartigny P., Halverson, G.P. (2016) Interpretation of the nitrogen isotopic composition of Precambrian sedimentary rocks: assumptions and perspectives. Chemical Geology 429, 93–10.
). The lack of systematic correlation between d15N values and C/N ratios among kerogen samples expected by the metamorphism (e.g., Haendal et al., 1986Haendal, D., Muhle, K., Nitzsche, H., Stiehl, G., Wand, U. (1986) Isotopic variations of the fixed nitrogen in metamorphic rocks. Geochimica et Cosmochimica Acta 50, 749–758.
) supports the above argument (Fig. S-3).The modern marine biosphere involves a nitrogen biological cycle that consists mainly of fixation (N2?NH4+), nitrification (NH4+?NO2-?NO3-), and denitrification (NO3-, NO2-?N2). When biological N2 fixation is dominant, the d15N values of OM tend to be close to the atmospheric value (0 ‰), because of minimal isotopic fractionation expected in fixation. However, when nitrification and denitrification are coupled with fixation, the d15N value of OM may grow to positive values. This isotopic shift is considered as evidence of isotopic fractionation driven by biological nitrification and denitrification (e.g., Shen et al., 2006
Shen, Y., Pinti, D.L., Hashizume, K. (2006) Biogeochemical cycles of sulfur and nitrogen in the Archean ocean and atmosphere. In: Benn, K., Mareschal, J.C., Condie, K. (Eds.) Archean Geodynamics and Environments, Geophyscal Monograph Series 164, 305–320.
; Brandes et al., 2007Brandes, J.A., Devol, A.H., Deutsch, C. (2007) New Developments in the Marine Nitrogen Cycle. Chemical Reviews 107, 577–589.
; Sigman et al., 2009Sigman, D.M., Karsh, K.L., Casciotti, K.L. (2009) Ocean process tracers: nitrogen isotopes in the ocean. In: Steele, J.H., Thorpe, S.A., Turekian, K.K. (Eds.) Encyclopedia of Ocean Science. Elsevier, Amsterdam, 4138–4153.
; Hashizume et al., 2016Hashizume, K., Pinti, D.L., Orberger, B., Cloquet, C., Jayananda, M., Soyama, H. (2016) A biological switch at the ocean surface as a cause of laminations in a Precambrian iron formation. Earth Planetary Science Letters 446, 27–36.
). The d15N values of OM can vary depending on the balance between the rate of N2 fixation and the rates of nitrification/denitrification (e.g., Lehmann, et al., 2002Lehmann, M.R., Bemasconi, S.M., Barbieri, A., McKenzie, J.A. (2002) Preservation of organic matter and alteration of its carbon and nitrogen isotope composition during simulated and in situ early sedimentary diagenesis. Geochimica et Cosmochimica Acta 66, 3573–3584.
, Pinti and Hashizume, 2011Pinti, D.L., Hashizume, K. (2011) Early life record from nitrogen isotopes. In: Golding, S., Glikson, M. (Eds.) Earliest Life on Earth: Habitats, Environments and Methods of Detection. Springer, Netherlands, 183–205.
). For example, Papineau et al. (2013)Papineau, D., Purohit, R., Fogel, M.L., Shields-Zuo, G.A. (2013) High phosphate availability as a possible cause for massive cyanobacterial production of oxygen in the Paleoproterozoic atmosphere. Earth and Planetary Science Letters 362, 225–236.
reported that the d15N values of OM in Palaeoproterozoic Indian sedimentary formations were approximately 0 ‰. These values were interpreted to be the result of a system dominated by N2 fixation associated with cyanobacterial blooms. In contrast, our data show much more positive d15N values for the bulk kerogen samples, reaching values as high as +8 ‰. Such positive values suggest that nitrite and nitrate were available in the Gunflint ocean. Kerogen from Palaeoproterozoic samples, except for phosphatic shales, also exhibit positive values, up to +12 ‰ (e.g., Beaumont and Robert, 1999Beaumont, V., Robert, F. (1999) Nitrogen isotope ratios of kerogens in Precambrian cherts: a record of the evolution of atmosphere chemistry? Precambrian Research 96, 63–82.
; Papineau et al., 2009Papineau, D., Purohit, R., Goldberg, T., Pi, D., Shields, G.A., Bhu, H., Steele, A., Fogel, M.L. (2009) High primary productivity and nitrogen cycling after the Paleoproterozoic phosphogenic event in the Aravalli Supergroup, India. Precambrian Research 171, 37–56.
, Godfrey et al., 2013Godfrey, L.V., Poulton, S.W., Bebout, G.E., Fralick, P.W. (2013) Stability of the nitrogen cycle during development of sulfidic water in the redox-stratified late Paleoproterozoic Ocean. Geology 41, 655–658.
, Stüeken et al., 2016Stüeken, E.E., Kipp, M.A., Koehler, M.C., Buick, R. (2016) The evolution of Earth’s biogeochemical nitrogen cycle. Earth-Science Reviews 160, 220–239.
). To operate such a biological nitrogen cycle, oxygenated surface water is required at this time.Stepwise combustion analyses of d15N were performed on kerogen samples mainly from the Current River section (see Supplementary Information and Table S-2). In this section, the lithology changed from shale (#0714, #0715) to carbonate-sand zone (#0711 to #0703) to arkosic sandstone (#0701, #0702). Such lithological changes represent a local marine regression. Figure 1 shows the results of the stepwise combustion analyses performed on 13 kerogen samples in our study. The average d15N values of the LT-fractions and the HT-fractions (d15NLT and d15NHT, respectively) were calculated using the d15N values and the released amounts for the relevant temperature steps. Some samples exhibited higher d15NHT than d15NLT, while other samples exhibited the opposite trend. The ranges of the d15N values for both fractions are similar to the overall range of bulk kerogen d15N values. In most samples, the HT-fractions have lower amounts of nitrogen than those of the LT-fractions. The differences in the combustion temperature possibly correspond to differences in the carbon structure for fractions of kerogen that co-exist in OM (Ishida et al., 2012
Ishida, A., Hashizume, K., Kakegawa, T. (2012) Stepwise combustion analyses of distinct nitrogen isotopic compositions on Paleoproterozoic organic matter, Geochemical Journal 46, 249–253.
).
Figure 1 Results of the stepwise combustion analyses of 13 kerogen samples from the Gunflint Formation (samples #0704 and #0708 are cited from Ishida et al., 2012
Ishida, A., Hashizume, K., Kakegawa, T. (2012) Stepwise combustion analyses of distinct nitrogen isotopic compositions on Paleoproterozoic organic matter, Geochemical Journal 46, 249–253.
). Nitrogen concentrations (white bars) and d15N values (black squares) are shown. The average d15N values and errors of both the lower-temperature and the higher-temperature fractions are shown as light green and dark green bars, respectively. Original data are summarised in Table S-2.The nitrogen released in the HT-fraction possibly has stronger bonds with carbon in the form of graphitised kerogen, compared to the LT-fraction. Ishida et al. (2012)
Ishida, A., Hashizume, K., Kakegawa, T. (2012) Stepwise combustion analyses of distinct nitrogen isotopic compositions on Paleoproterozoic organic matter, Geochemical Journal 46, 249–253.
concluded that the HT-fraction might not be a metamorphosed portion of OM with the same origin as the LT-fraction, since the HT-fraction does not always show higher d15N values than the LT-fraction. Because the chemical structures of kerogen are known to be heterogeneous, some components of kerogen are easy to graphitise but the others are non-graphitised (e.g., Bustin et al., 1995Bustin, R.M., Ross, J.V. Rouzaud, J.N. (1995) Mechanisms of graphite formation from kerogen: Experimental evidence. International Journal of Coal Geology 28, 1–36.
; Beyssac et al., 2002Beyssac, O., Rouzaud, J.N., Goffe’, B., Brunet, F., Chopin, C. (2002) Graphitization in a high-pressure, low-temperature metamorphic gradient: a Raman microspectroscopy and HRTEM study. Contributions to Mineralogy and Petrology 143, 19–31.
). We interpret the OM observed in the HT-fraction to represent the residue of graphitised parts of kerogen. Such graphitisation could be promoted by the heterotrophic microbial degradation during diagenesis. Thus, we interpret the nitrogen in the LT-fractions to represent more indigenous nitrogen isotope compositions in the ecosystem at that time.A positive correlation was observed between the d15NLT and the Pr/Sm ratios of bulk rock (Fig. 2a). The Pr/Sm ratios among our samples are interpreted to represent the contribution of continental inputs to the ocean, supported by the negative correlation with Eu/Eu* (Bolhar et al., 2005
Bolhar, R., Van Krenendonk, M., Kamber, B.S. (2005) A trace element study of siderite-jasper banded iron formation in the 3.45 Ga Warrawoona Group, Pilbara Craton-Formation from hydrothermal fluids and shallow seawater. Precambrian Research 137, 93–114.
) (Fig. 3a), and positive correlations with abundances of elements that represent detrital inputs (Fig. 3b,c). The d15NHT showed a poor correlation with the Pr/Sm ratios (Fig. 2b). The variable d15NLT values in a positive range and their correlation to the Pr/Sm ratios could be caused by intense nitrification/denitrification when the nutrient supply from the land enhanced.Table 1 Summary of light and trace element concentrations and the isotope compositions of bulk rock and kerogen samples.
section | sample ID | litho facies | bulk rock | kerogen | |||||||||||||
Total C | TOC | Ccarb | Spyr | Pr/Sm | Eu/Eu* | C | N | H | S | C/N | d13C | d34S (pyr) | d15N | ± | |||
wt. % | (PAAS normalised)1 | wt. % | atom | ‰ | |||||||||||||
Pass Lake Road | 0716 | Chert | 0.1 | 0.0 | 0.1 | 0.0 | 1.22 | 0.87 | n.a. | n.a. | n.a. | n.a. | - | n.a. | n.a. | n.a. | n.a. |
0718 | Carbonate | 6.6 | 0.0 | 6.6 | 0.0 | 0.61 | 2.22 | n.a. | n.a. | n.a. | n.a. | - | n.a. | n.a. | n.a. | n.a. | |
0719 | Silicified carbonate | 3.1 | 0.0 | 3.1 | 0.0 | 0.66 | 2.24 | n.a. | n.a. | n.a. | n.a. | - | n.a. | n.a. | n.a. | n.a. | |
0720 | Silicified carbonate | 3.7 | 0.1 | 3.6 | 0.0 | 0.67 | 2.18 | n.a. | n.a. | n.a. | n.a. | - | n.a. | n.a. | n.a. | n.a. | |
0721 | Taconite | 3.0 | 0.2 | 2.9 | 0.6 | 0.82 | 1.78 | 16.8 | 0.2 | 0.3 | 32.6 | 109.6 | -32.6 | 3.0 | 4.7 | 0.60 | |
0722 | Chert (BIF) | 0.2 | 0.0 | 0.2 | 0.0 | 0.88 | 1.73 | n.a. | n.a. | n.a. | n.a. | - | n.a. | n.a. | 6.8 | 1.00 | |
0723 | Carbonate (BIF) | 5.2 | 0.1 | 5.1 | 0.1 | 0.82 | 1.41 | n.a. | n.a. | n.a. | n.a. | - | -25.1 | n.a. | 5.4 | 0.40 | |
Blend River North | 0301 | Black shale | 0.3 | 0.3 | 0.0 | 0.0 | 1.76 | 1.25 | 70.7 | 1.2 | 3.5 | 5.6 | 67.4 | -31.6 | 2.1 | 2.9 | 0.70 |
0302 | Black shale | 0.8 | 0.3 | 0.5 | 0.2 | 0.49 | 1.48 | 53.1 | 0.6 | 2.4 | 18.2 | 96.2 | -31.6 | 4.7 | n.a. | n.a. | |
0303 | Ferruginous shale | 4.1 | 0.3 | 3.8 | 0.1 | 0.76 | 1.54 | 69.4 | 1.2 | 3.2 | 9.6 | 67.6 | -31.3 | 7.1 | n.a. | n.a. | |
0304 | Black shale | 1.1 | 0.9 | 0.1 | 0.0 | 1.43 | 1.19 | 73.1 | 1.1 | 3.2 | 3.2 | 75.5 | -32.1 | 8.6 | 5.9 | 0.70 | |
0305 | Carbonate | 9.1 | 0.8 | 8.3 | 0.4 | 0.69 | 1.59 | 49.8 | 0.9 | 2.0 | 18.8 | 64.7 | -31.9 | 8.2 | 6.5 | 0.70 | |
0306 | Ferruginous carbonate | 10.4 | 0.3 | 10.2 | 0.1 | 0.78 | 1.32 | 60.5 | 1.1 | 2.9 | 13.3 | 63.9 | -29.8 | n.a. | n.a. | n.a. | |
0307 | Silicified carbonate | 5.0 | 0.1 | 4.9 | 0.0 | 0.78 | 1.54 | n.a. | n.a. | n.a. | n.a. | - | n.a. | n.a. | n.a. | n.a. | |
0308 | Carbonate/shale | 4.0 | 0.0 | 3.9 | 0.0 | 0.83 | 1.18 | 44.1 | 0.0 | 0.0 | 15.2 | - | n.a. | n.a. | n.a. | n.a. | |
0309 | Ferruginous carbonate | 9.9 | 0.2 | 9.7 | 0.0 | 0.83 | 1.27 | 76.0 | 1.4 | 3.5 | 5.7 | 65.6 | n.a. | n.a. | n.a. | n.a. | |
0310 | Chert | 3.2 | 0.1 | 3.1 | 0.0 | 0.83 | 1.29 | n.a. | n.a. | n.a. | n.a. | - | n.a. | n.a. | n.a. | n.a. | |
0311 | Chert | 1.9 | 0.0 | 1.8 | 0.0 | 0.68 | 0.72 | 60.3 | 0.7 | 2.2 | 8.5 | 94.9 | n.a. | n.a. | n.a. | n.a. | |
Current River | 0701 | Carbonate-rich arkosic sandstone | 4.7 | 0.5 | 4.2 | 0.0 | 0.86 | 1.28 | 67.3 | 0.9 | 2.2 | 5.6 | 91.2 | -33.0 | 26.9 | 5.6 | 0.80 |
0702 | Arkosic sandstone | 0.8 | 0.2 | 0.6 | 0.0 | 1.87 | 1.11 | 56.6 | 0.6 | 3.2 | 2.5 | 101.7 | -32.2 | 8.3 | 6.7 | 0.70 | |
0703 | Sandy carbonate | 4.2 | 0.2 | 4.0 | 0.0 | 0.99 | 1.24 | 82.7 | 1.1 | 3.7 | 2.8 | 88.5 | -32.3 | 10.9 | 7.1 | 0.50 | |
0704 | Sandy carbonate | 5.9 | 0.2 | 5.7 | 0.0 | 0.98 | 1.18 | 82.9 | 1.0 | 3.8 | 3.2 | 98.4 | -32.5 | 11.2 | 4.9 | 1.00 | |
0705 | Sandy carbonate | 4.4 | 0.1 | 4.3 | 0.0 | 1.23 | 1.42 | 52.6 | 0.7 | 2.2 | 14.8 | 86.8 | -31.6 | 9.7 | 6.0 | 0.70 | |
0706 | Sandy carbonate | 5.4 | 0.3 | 5.1 | 0.2 | 1.03 | 1.52 | 47.8 | 0.6 | 2.0 | 19.6 | 87.6 | -32.6 | 15.1 | 6.9 | 0.60 | |
0707 | Sandy carbonate | 2.9 | 0.2 | 2.8 | 0.0 | 0.92 | 1.18 | 66.1 | 0.8 | 2.8 | 10.2 | 98.3 | -31.9 | 5.8 | 5.9 | 0.80 | |
0708 | Sandy carbonate | 7.1 | 0.3 | 6.7 | 0.0 | 0.76 | 1.76 | 85.7 | 1.0 | 4.1 | 1.6 | 98.8 | -32.9 | 16.0 | 6.9 | 0.50 | |
0709 | Laminated carbonate with detrital quartz | 1.8 | 0.2 | 1.6 | 0.1 | 1.03 | 1.31 | 59.9 | 0.8 | 2.5 | 14.2 | 86.7 | -32.4 | 14.8 | 7.3 | 0.60 | |
0710 | Laminated carbonate with detrital quartz | 0.3 | 0.1 | 0.2 | 0.0 | 1.18 | 1.48 | 72.1 | 1.0 | 4.4 | 5.9 | 80.3 | -32.0 | - | 3.2 | 0.60 | |
0711 | Massive carbonate | 9.0 | 0.1 | 8.9 | 0.1 | 0.93 | 1.63 | 54.3 | 0.8 | 2.9 | 14.6 | 80.9 | -32.6 | 13.4 | 8.0 | 0.40 | |
0712 | Laminated carbonate with detrital quartz | 0.7 | 0.3 | 0.4 | 0.1 | 1.16 | 1.63 | 66.0 | 1.0 | 3.4 | 9.4 | 78.3 | -32.5 | 11.3 | 7.2 | 0.30 | |
0713 | Carbonate shale (phosphorous) | 6.2 | 0.2 | 6.0 | 0.1 | 1.02 | 1.68 | 43.2 | 0.6 | 1.8 | 20.7 | 90.1 | -32.4 | 9.2 | 6.4 | 0.60 | |
0714 | Black shale | 4.5 | 0.9 | 3.7 | 0.1 | 1.42 | 1.22 | 68.6 | 1.0 | 3.0 | 4.8 | 78.2 | -33.6 | 9.3 | 4.8 | 0.90 | |
0715 | Black shale | 0.2 | 0.3 | 0.0 | 0.0 | 1.20 | 1.28 | 8.2 | 0.2 | 1.2 | 1.6 | 61.3 | -32.2 | 11.5 | 5.9 | 0.70 | |
Telly Fox | 0724 | Carbonate | 9.7 | 0.3 | 9.3 | 0.2 | 0.93 | 1.53 | 37.8 | 0.6 | 1.7 | 25.4 | 77.5 | -31.4 | -1.1 | n.a. | n.a. |
0725 | Silicified shale | 0.2 | 0.2 | 0.0 | 0.4 | 0.63 | 1.23 | n.a. | n.a. | n.a. | n.a. | - | -31.5 | 20.7 | 5.5 | 0.70 | |
0726 | Carbonate/shale | 0.3 | 0.4 | 0.0 | 0.2 | 0.71 | 1.40 | 60.1 | 0.9 | 2.9 | 12.5 | 78.6 | -31.6 | 10.8 | 4.5 | 0.30 | |
0729 | Silicified carbonate | 0.3 | 0.1 | 0.3 | 0.1 | 0.24 | 0.72 | n.a. | n.a. | n.a. | n.a. | - | -28.9 | n.a. | 4.3 | 0.30 | |
0730 | Silicified carbonate | 0.3 | 0.1 | 0.2 | 0.0 | 0.20 | 0.99 | n.a. | n.a. | n.a. | n.a. | - | n.a. | n.a. | n.a. | n.a. | |
Kakabeka Fall | 0601 | Shale (BIF) | 7.3 | 0.8 | 6.6 | 0.2 | 0.92 | 1.67 | 64.8 | 1.2 | 2.8 | 9.9 | 62.5 | -31.3 | 5.9 | 6.3 | 0.80 |
0602 | Chert (BIF) | 0.3 | 0.1 | 0.2 | 0.0 | 0.41 | 0.87 | 57.5 | 1.0 | 2.5 | 8.5 | 68.2 | -29.7 | n.a. | n.a. | n.a. | |
0603 | Shale (BIF) | 5.5 | 0.4 | 5.1 | 0.1 | 0.84 | 1.46 | 60.6 | 1.0 | 2.0 | 12.2 | 70.8 | -30.9 | 6.1 | n.a. | n.a. | |
0604 | Ferruginous chert | 2.7 | 0.1 | 2.6 | 0.1 | 1.16 | 1.37 | 46.4 | 0.9 | 1.6 | 15.6 | 60.3 | -27.8 | n.a. | n.a. | n.a. | |
0605 | Ferruginous chert | 0.0 | 0.0 | 0.0 | 0.0 | 0.75 | 0.72 | n.a. | n.a. | n.a. | n.a. | - | n.a. | n.a. | n.a. | n.a. |
Analytical error of d13C and d34S are within 0.2 ‰.
Underlined values: below instumental reproducibility (0.1 %), n.a.: not analysed because of low amount of organic matter.
1 Details are described in Tables S-1 and S-4.
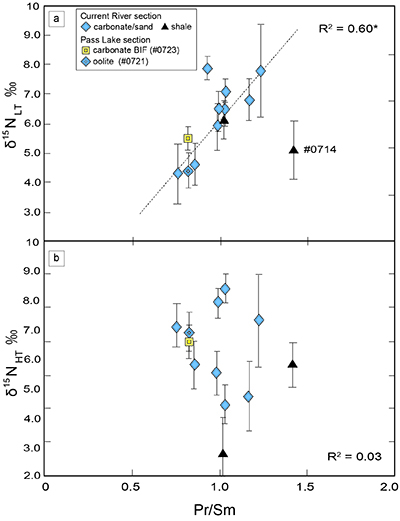
Figure 2 Correlation diagrams between the Pr/Sm ratios and (a) the d15NLT values and (b) the d15NHT values, respectively. A positive correlation is observed between the Pr/Sm ratios and the d15NLT values (R2 = 0.60, excluding #0714), whereas no correlation is observed between the d15NHT values.

Figure 3 Correlation diagrams between the Pr/Sm ratios and (a) the Eu/Eu* ratios, (b) TiO2, and (c) Zr concentrations. The Eu/Eu* is defined as Eu/0.5(Gd+Sm). Grey square areas indicate the range of modern ocean values. Dashed and solid arrows indicate the directions of hydrothermal fluid and crustal contributions, respectively (Bolhar et al., 2005
Bolhar, R., Van Krenendonk, M., Kamber, B.S. (2005) A trace element study of siderite-jasper banded iron formation in the 3.45 Ga Warrawoona Group, Pilbara Craton-Formation from hydrothermal fluids and shallow seawater. Precambrian Research 137, 93–114.
). Positive correlations exist between the Pr/Sm and TiO2 and Zr concentrations.top
Palaeo-ecosystem in the Gunflint Ocean
The carbon isotope compositions (d13C values) of the examined kerogen samples, ranging from -33.6 to -25.1 ‰, are consistent with the carbon isotope values fixed by cyanobacteria (e.g., Schidlowski, 1988
Schidlowski, M. (1988) A 3,800-million-year isotopic record of life from carbon in sedimentary rocks. Nature 333, 313–318.
; House et al., 2000House, H.C., Schopf, J.W., McKeegan, K.D., Coath, C.D., Harrison, T.M., Stetter, K.O. (2000) Carbon isotopic composition of individual Precambrian microossils. Geology 28, 707–710.
). The d13C of kerogen in the sedimentary rocks, in general, corresponds to the values of contemporary primary producers. The presence of oxygenic primary producers in the Gunflint ocean is consistent with the biological nitrogen cycle proposed in the present study, and with the mineralogical and trace element geochemistry (see Supplementary Information).The OM sinking through the water column would consume the dissolved oxygen, producing a temporary or partial sub-oxic zone in the Gunflint ocean, similar to a red tide event in the modern ocean (Minagawa and Wada, 1986
Minagawa, M., Wada, E. (1986) Nitrogen isotope ratios of red tide organisms in the east china sea: A characterization of biological nitrogen fixation. Marine Chemistry 19, 245–259.
). In the water column with spatially variable redox conditions ranging from oxic to anoxic, nitrification/denitrification processes could be enhanced, thereby inducing 15N enrichment in the OM (e.g., Shen et al., 2006Shen, Y., Pinti, D.L., Hashizume, K. (2006) Biogeochemical cycles of sulfur and nitrogen in the Archean ocean and atmosphere. In: Benn, K., Mareschal, J.C., Condie, K. (Eds.) Archean Geodynamics and Environments, Geophyscal Monograph Series 164, 305–320.
; Sigman et al., 2009Sigman, D.M., Karsh, K.L., Casciotti, K.L. (2009) Ocean process tracers: nitrogen isotopes in the ocean. In: Steele, J.H., Thorpe, S.A., Turekian, K.K. (Eds.) Encyclopedia of Ocean Science. Elsevier, Amsterdam, 4138–4153.
). One of the triggers of red tide in the modern ocean environment is considered to be an increased amount of nutrient supply to the ocean from the land (e.g., Taylor et al., 1995Taylor, D., Nixon, S., Granger, S., Buckley, B. (1995) Nutrient limitation and the eutrophication of coastal lagoons. Marine Ecology Progress Series 127, 235–244.
; Valiela et al., 1997Valiela, I., McClelland, J., Hauxwell, J., Behr, P.J., Hersh, D., Foreman, K. (1997) Macroalgal blooms in shallow estuaries: Controls and ecophysiological and ecosystem consequences. Limnology and Oceanography 42, 1105–1118.
; Hauxwell et al., 1998Hauxwell, J., McClelland, J., Behr, P.J., Valiela, I. (1998) Relative importance of grazing and nutrient controls of macroalgal biomass in three temperate shallow estuaries. Estuaries 21, 347–360.
). The rapid growth of continents in the Animikie basin enhanced the supply of elements from the terrestrial region to the Gunflint ocean. Our data suggest that increased continental input brought more phosphorus and trace metal elements to the sedimentary environment (Fig. S-3, Table S-5), rather than modern-like nutrients such as dissolved OM, which are less likely at this time. The increased flux of such elements into the Gunflint ocean would promote the high production and nitrogen cycling in the biosphere of the shallow ocean (e.g., Papineau et al., 2009Papineau, D., Purohit, R., Goldberg, T., Pi, D., Shields, G.A., Bhu, H., Steele, A., Fogel, M.L. (2009) High primary productivity and nitrogen cycling after the Paleoproterozoic phosphogenic event in the Aravalli Supergroup, India. Precambrian Research 171, 37–56.
; Edwards et al., 2012Edwards, C.T., Pufahl, P.K., Hiatt, E.E., Kyser, T.K. (2012) Paleoenvironmental and taphonomic controls on the occurrence of Paleoproterozoic microbial communities in the 1.88 Ga Ferriman Group, Labrador Trough, Canada. Precambrian Research 212–213, 91–106.
; Stüeken et al., 2015Stüeken, E.E., Buick, R., Guy, B.M., Koehler, M.C. (2015) Isotopic evidence for biological nitrogen fixation by molybdenum-nitrogenase from 3.2 Gyr. Nature 520, 666–674.
), as represented by the positive correlation between Pr/Sm ratios and d15NLT of kerogen observed in this study.The active production of OM may have facilitated the formation of the organic-rich sediments as well. The high flux of OM would keep the interior of sediments under suboxic to anoxic conditions, where the activity of sulphate-reducing bacteria was promoted. Such conditions would produce the heavier d34S values in the final products, such as pyrite (see Table 1 and Supplementary Information). A local involvement of methanogens and methanotrophs in such a benthic or subsurface environment is also plausible and is supported by the carbon isotope study of microfossils (House et al., 2000
House, H.C., Schopf, J.W., McKeegan, K.D., Coath, C.D., Harrison, T.M., Stetter, K.O. (2000) Carbon isotopic composition of individual Precambrian microossils. Geology 28, 707–710.
). The primary OM precipitated to the sea floor would be decomposed by those heterotrophic bacteria, resulting in nitrogen isotope heterogeneities in the kerogen left in the sediments. The combined approaches of stepwise nitrogen isotope analyses and multiple geochemical analyses in this study can be used as a proxy to understand the evolution of biosphere and ocean environment in the Precambrian age.top
Acknowledgements
Constructive reviews by Dr. Stüeken and an anonymous reviewer greatly helped to improve the quality of the article. This research was supported by Grant-in-Aid for JSPS (DC1) to A.I. (21-2645), and by KAKENHI to T.K. (24403013, 15H021440), and K.H. (JP17340168).
Editor: Bruce Watson
top
References
Ader, M., Thomazo, C., Sansjofre, P., Busigny, V., Papineau, D., Laffont, R., Cartigny P., Halverson, G.P. (2016) Interpretation of the nitrogen isotopic composition of Precambrian sedimentary rocks: assumptions and perspectives. Chemical Geology 429, 93–10.

The nitrogen isotope compositions (d15N values) of organic matter (OM) found in ancient sedimentary rocks are used as proxies for reconstructing the microbial nitrogen cycle and the palaeo-oceanic environment (e.g., Beaumont and Robert, 1999, Shen et al., 2006; Ader et al., 2016; Stüeken et al., 2016).
View in article
However, the low metamorphic grade of the Gunflint Formation (below greenschist facies) cannot be responsible for a significant fractionation, up to 5 ‰ (e.g., Haendal et al., 1986, Ader et al., 2016).
View in article
Beaumont, V., Robert, F. (1999) Nitrogen isotope ratios of kerogens in Precambrian cherts: a record of the evolution of atmosphere chemistry? Precambrian Research 96, 63–82.

The nitrogen isotope compositions (d15N values) of organic matter (OM) found in ancient sedimentary rocks are used as proxies for reconstructing the microbial nitrogen cycle and the palaeo-oceanic environment (e.g., Beaumont and Robert, 1999, Shen et al., 2006; Ader et al., 2016; Stüeken et al., 2016).
View in article
The d15N values of the bulk kerogen samples, ranging between +2.9 and +8.0 ‰, are consistent with previous reports (e.g., Beaumont and Robert, 1999; Godfrey et al., 2013).
View in article
Kerogen from Palaeoproterozoic samples, except for phosphatic shales, also exhibit positive values, up to +12 ‰ (e.g., Beaumont and Robert, 1999; Papineau et al., 2009, Godfrey et al., 2013, Stüeken et al., 2016).
View in article
Beyssac, O., Rouzaud, J.N., Goffe’, B., Brunet, F., Chopin, C. (2002) Graphitization in a high-pressure, low-temperature metamorphic gradient: a Raman microspectroscopy and HRTEM study. Contributions to Mineralogy and Petrology 143, 19–31.

Because the chemical structures of kerogen are known to be heterogeneous, some components of kerogen are easy to graphitise but the others are non-graphitised (e.g., Bustin et al., 1995; Beyssac et al., 2002).
View in article
Bolhar, R., Van Krenendonk, M., Kamber, B.S. (2005) A trace element study of siderite-jasper banded iron formation in the 3.45 Ga Warrawoona Group, Pilbara Craton-Formation from hydrothermal fluids and shallow seawater. Precambrian Research 137, 93–114.

The Pr/Sm ratios among our samples are interpreted to represent the contribution of continental inputs to the ocean, supported by the negative correlation with Eu/Eu* (Bolhar et al., 2005) (Fig. 3a), and positive correlations with abundances of elements that represent detrital inputs (Fig. 3b,c).
View in article
Figure 3 [...] Dashed and solid arrows indicate the directions of hydrothermal fluid and crustal contributions, respectively (Bolhar et al., 2005).
View in article
Brandes, J.A., Devol, A.H., Deutsch, C. (2007) New Developments in the Marine Nitrogen Cycle. Chemical Reviews 107, 577–589.

This isotopic shift is considered as evidence of isotopic fractionation driven by biological nitrification and denitrification (e.g., Shen et al., 2006; Brandes et al., 2007; Sigman et al., 2009; Hashizume et al., 2016).
View in article
Bustin, R.M., Ross, J.V. Rouzaud, J.N. (1995) Mechanisms of graphite formation from kerogen: Experimental evidence. International Journal of Coal Geology 28, 1–36.

Because the chemical structures of kerogen are known to be heterogeneous, some components of kerogen are easy to graphitise but the others are non-graphitised (e.g., Bustin et al., 1995; Beyssac et al., 2002).
View in article
Edwards, C.T., Pufahl, P.K., Hiatt, E.E., Kyser, T.K. (2012) Paleoenvironmental and taphonomic controls on the occurrence of Paleoproterozoic microbial communities in the 1.88 Ga Ferriman Group, Labrador Trough, Canada. Precambrian Research 212–213, 91–106.

The increased flux of such elements into the Gunflint ocean would promote the high production and nitrogen cycling in the biosphere of the shallow ocean (e.g., Papineau et al., 2009; Edwards et al., 2012; Stüeken et al., 2015), as represented by the positive correlation between Pr/Sm ratios and d15NLT of kerogen observed in this study.
View in article
Fralick, P., Davis, D.W., Kissin, S.A. (2002) The age of Gunflint Formation, Ontario, Canada: single zircon U-Pb age determinations from reworked volcanic ash. Canadian Journal of Earth Science 39, 1085–1091.

The age of the Gunflint Formation is estimated as 1878 ± 1.3 Ma by U-Pb dating (Fralick et al., 2002).
View in article
Godfrey, L.V., Poulton, S.W., Bebout, G.E., Fralick, P.W. (2013) Stability of the nitrogen cycle during development of sulfidic water in the redox-stratified late Paleoproterozoic Ocean. Geology 41, 655–658.

The d15N values of the bulk kerogen samples, ranging between +2.9 and +8.0 ‰, are consistent with previous reports (e.g., Beaumont and Robert, 1999; Godfrey et al., 2013).
View in article
Kerogen from Palaeoproterozoic samples, except for phosphatic shales, also exhibit positive values, up to +12 ‰ (e.g., Beaumont and Robert, 1999; Papineau et al., 2009, Godfrey et al., 2013, Stüeken et al., 2016).
View in article
Goodwin, A.M. (1956) Facies relations in the Gunflint iron formation. Economic Geology 51, 565-595.

The Gunflint Formation, belonging to the Animikie Group, is located on the northwestern shore of Lake Superior, Ontario, Canada (Goodwin, 1956).
View in article
Haendal, D., Muhle, K., Nitzsche, H., Stiehl, G., Wand, U. (1986) Isotopic variations of the fixed nitrogen in metamorphic rocks. Geochimica et Cosmochimica Acta 50, 749–758.

However, the low metamorphic grade of the Gunflint Formation (below greenschist facies) cannot be responsible for a significant fractionation, up to 5 ‰ (e.g., Haendal et al., 1986, Ader et al., 2016).
View in article
The lack of systematic correlation between d15N values and C/N ratios among kerogen samples expected by the metamorphism (e.g., Haendal et al., 1986) supports the above argument (Fig. S-3).
View in article
Hashizume, K., Pinti, D.L., Orberger, B., Cloquet, C., Jayananda, M., Soyama, H. (2016) A biological switch at the ocean surface as a cause of laminations in a Precambrian iron formation. Earth Planetary Science Letters 446, 27–36.

This isotopic shift is considered as evidence of isotopic fractionation driven by biological nitrification and denitrification (e.g., Shen et al., 2006; Brandes et al., 2007; Sigman et al., 2009; Hashizume et al., 2016).
View in article
Hauxwell, J., McClelland, J., Behr, P.J., Valiela, I. (1998) Relative importance of grazing and nutrient controls of macroalgal biomass in three temperate shallow estuaries. Estuaries 21, 347–360.

One of the triggers of red tide in the modern ocean environment is considered to be an increased amount of nutrient supply to the ocean from the land (e.g., Taylor et al., 1995; Valiela et al., 1997; Hauxwell et al., 1998).
View in article
House, H.C., Schopf, J.W., McKeegan, K.D., Coath, C.D., Harrison, T.M., Stetter, K.O. (2000) Carbon isotopic composition of individual Precambrian microossils. Geology 28, 707–710.

The carbon isotope compositions (d13C values) of the examined kerogen samples, ranging from -33.6 to -25.1 ‰, are consistent with the carbon isotope values fixed by cyanobacteria (e.g., Schidlowski, 1988; House et al., 2000).
View in article
A local involvement of methanogens and methanotrophs in such a benthic or subsurface environment is also plausible and is supported by the carbon isotope study of microfossils (House et al., 2000).
View in article
Ishida, A., Hashizume, K., Kakegawa, T. (2012) Stepwise combustion analyses of distinct nitrogen isotopic compositions on Paleoproterozoic organic matter, Geochemical Journal 46, 249–253.

A preliminary study was performed using kerogen samples extracted from two rock samples from the Gunflint Formation, indicating temperature-dependent d15N heterogeneities within each kerogen sample (Ishida et al., 2012).
View in article
The analytical protocols and performances are described in Ishida et al. (2012), and references therein.
View in article
Empirically two distinct nitrogen isotope plateau appear in kerogen analyses: one appears at temperatures between 475 ºC and 575 ºC (lower temperature fraction: LT-fraction), and the other appears at temperatures more than 575 ºC (higher temperature fraction: HT-fraction) (Ishida et al., 2012)
View in article
The differences in the combustion temperature possibly correspond to differences in the carbon structure for fractions of kerogen that co-exist in OM (Ishida et al., 2012).
View in article
Figure 1 Results of the stepwise combustion analyses of 13 kerogen samples from the Gunflint Formation (samples #0704 and #0708 are cited from Ishida et al., 2012).
View in article
Ishida et al. (2012) concluded that the HT-fraction might not be a metamorphosed portion of OM with the same origin as the LT-fraction, since the HT-fraction does not always show higher d15N values than the LT-fraction.
View in article
Lehmann, M.R., Bemasconi, S.M., Barbieri, A., McKenzie, J.A. (2002) Preservation of organic matter and alteration of its carbon and nitrogen isotope composition during simulated and in situ early sedimentary diagenesis. Geochimica et Cosmochimica Acta 66, 3573–3584.

The d15N values of OM can vary depending on the balance between the rate of N2 fixation and the rates of nitrification/denitrification (e.g., Lehmann, et al., 2002, Pinti and Hashizume, 2011).
View in article
Minagawa, M., Wada, E. (1986) Nitrogen isotope ratios of red tide organisms in the east china sea: A characterization of biological nitrogen fixation. Marine Chemistry 19, 245–259.

The OM sinking through the water column would consume the dissolved oxygen, producing a temporary or partial sub-oxic zone in the Gunflint ocean, similar to a red tide event in the modern ocean (Minagawa and Wada, 1986).
View in article
Papineau, D., Purohit, R., Goldberg, T., Pi, D., Shields, G.A., Bhu, H., Steele, A., Fogel, M.L. (2009) High primary productivity and nitrogen cycling after the Paleoproterozoic phosphogenic event in the Aravalli Supergroup, India. Precambrian Research 171, 37–56.

Kerogen from Palaeoproterozoic samples, except for phosphatic shales, also exhibit positive values, up to +12 ‰ (e.g., Beaumont and Robert, 1999; Papineau et al., 2009, Godfrey et al., 2013, Stüeken et al., 2016).
View in article
The increased flux of such elements into the Gunflint ocean would promote the high production and nitrogen cycling in the biosphere of the shallow ocean (e.g., Papineau et al., 2009; Edwards et al., 2012; Stüeken et al., 2015), as represented by the positive correlation between Pr/Sm ratios and d15NLT of kerogen observed in this study.
View in article
Papineau, D., Purohit, R., Fogel, M.L., Shields-Zuo, G.A. (2013) High phosphate availability as a possible cause for massive cyanobacterial production of oxygen in the Paleoproterozoic atmosphere. Earth and Planetary Science Letters 362, 225–236.

For example, Papineau et al. (2013) reported that the d15N values of OM in Palaeoproterozoic Indian sedimentary formations were approximately 0 ‰.
View in article
Pinti, D.L., Hashizume, K. (2011) Early life record from nitrogen isotopes. In: Golding, S., Glikson, M. (Eds.) Earliest Life on Earth: Habitats, Environments and Methods of Detection. Springer, Netherlands, 183–205.

The d15N values of OM can vary depending on the balance between the rate of N2 fixation and the rates of nitrification/denitrification (e.g., Lehmann, et al., 2002, Pinti and Hashizume, 2011).
View in article
Schidlowski, M. (1988) A 3,800-million-year isotopic record of life from carbon in sedimentary rocks. Nature 333, 313–318.

The carbon isotope compositions (d13C values) of the examined kerogen samples, ranging from -33.6 to -25.1 ‰, are consistent with the carbon isotope values fixed by cyanobacteria (e.g., Schidlowski, 1988; House et al., 2000).
View in article
Schulz, K.J., Cannon, W.F. (2007) The Penokean orogeny in the Lake Superior region. Precambrian Research 157, 4–25.

An evolution model for the Animikie Basin has been proposed by, e.g., Schulz and Cannon (2007).
View in article
Shen, Y., Pinti, D.L., Hashizume, K. (2006) Biogeochemical cycles of sulfur and nitrogen in the Archean ocean and atmosphere. In: Benn, K., Mareschal, J.C., Condie, K. (Eds.) Archean Geodynamics and Environments, Geophyscal Monograph Series 164, 305–320.

The nitrogen isotope compositions (d15N values) of organic matter (OM) found in ancient sedimentary rocks are used as proxies for reconstructing the microbial nitrogen cycle and the palaeo-oceanic environment (e.g., Beaumont and Robert, 1999, Shen et al., 2006; Ader et al., 2016; Stüeken et al., 2016).
View in article
This isotopic shift is considered as evidence of isotopic fractionation driven by biological nitrification and denitrification (e.g., Shen et al., 2006; Brandes et al., 2007; Sigman et al., 2009; Hashizume et al., 2016).
View in article
In the water column with spatially variable redox conditions ranging from oxic to anoxic, nitrification/denitrification processes could be enhanced, thereby inducing 15N enrichment in the OM (e.g., Shen et al., 2006; Sigman et al., 2009).
View in article
Sigman, D.M., Karsh, K.L., Casciotti, K.L. (2009) Ocean process tracers: nitrogen isotopes in the ocean. In: Steele, J.H., Thorpe, S.A., Turekian, K.K. (Eds.) Encyclopedia of Ocean Science. Elsevier, Amsterdam, 4138–4153.

This isotopic shift is considered as evidence of isotopic fractionation driven by biological nitrification and denitrification (e.g., Shen et al., 2006; Brandes et al., 2007; Sigman et al., 2009; Hashizume et al., 2016).
View in article
In the water column with spatially variable redox conditions ranging from oxic to anoxic, nitrification/denitrification processes could be enhanced, thereby inducing 15N enrichment in the OM (e.g., Shen et al., 2006; Sigman et al., 2009).
View in article
Stüeken, E.E., Buick, R., Guy, B.M., Koehler, M.C. (2015) Isotopic evidence for biological nitrogen fixation by molybdenum-nitrogenase from 3.2 Gyr. Nature 520, 666–674.

The increased flux of such elements into the Gunflint ocean would promote the high production and nitrogen cycling in the biosphere of the shallow ocean (e.g., Papineau et al., 2009; Edwards et al., 2012; Stüeken et al., 2015), as represented by the positive correlation between Pr/Sm ratios and d15NLT of kerogen observed in this study.
View in article
Stüeken, E.E., Kipp, M.A., Koehler, M.C., Buick, R. (2016) The evolution of Earth’s biogeochemical nitrogen cycle. Earth-Science Reviews 160, 220–239.

The nitrogen isotope compositions (d15N values) of organic matter (OM) found in ancient sedimentary rocks are used as proxies for reconstructing the microbial nitrogen cycle and the palaeo-oceanic environment (e.g., Beaumont and Robert, 1999, Shen et al., 2006; Ader et al., 2016; Stüeken et al., 2016).
View in article
Kerogen from Palaeoproterozoic samples, except for phosphatic shales, also exhibit positive values, up to +12 ‰ (e.g., Beaumont and Robert, 1999; Papineau et al., 2009, Godfrey et al., 2013, Stüeken et al., 2016).
View in article
Taylor, D., Nixon, S., Granger, S., Buckley, B. (1995) Nutrient limitation and the eutrophication of coastal lagoons. Marine Ecology Progress Series 127, 235–244.

One of the triggers of red tide in the modern ocean environment is considered to be an increased amount of nutrient supply to the ocean from the land (e.g., Taylor et al., 1995; Valiela et al., 1997; Hauxwell et al., 1998).
View in article
Valiela, I., McClelland, J., Hauxwell, J., Behr, P.J., Hersh, D., Foreman, K. (1997) Macroalgal blooms in shallow estuaries: Controls and ecophysiological and ecosystem consequences. Limnology and Oceanography 42, 1105–1118.

One of the triggers of red tide in the modern ocean environment is considered to be an increased amount of nutrient supply to the ocean from the land (e.g., Taylor et al., 1995; Valiela et al., 1997; Hauxwell et al., 1998).
View in article
top
Supplementary Information
1. The Gunflint Formation
The Gunflint Formation is located on the north-western shore of Lake Superior, Ontario, Canada (Fig. S-1). Together with the Rove Formation, the Gunflint Formation belongs to the Animikie Group (Goodwin, 1956). The Animikie Group unconformably overlies the Archean Superior craton, which consists of the accretion of volcanic and sedimentary rocks originally formed by the Algoman orogeny (e.g., Ojakangas et al., 2001). The Gunflint Formation sedimentation began with quartz sand in a tidal environment and was often associated with stromatolites. Owing to water depth fluctuations, the sedimentary rocks transitioned to chert carbonate, iron formations, or taconitic carbonate. The Gunflint Formation is overlain by the shale-dominated Rove Formation. In particular, laterally uniform banded iron formations (BIFs) and iron-rich layers formed from Ontario to Minnesota in the same basin (Schulz and Cannon, 2007).
The sedimentary rocks of the Animikie Group formed in close association with the Penokean orogenic events, which involved rifting, submarine hydrothermal activity, subduction, accretion of arc volcanic and magmatic rocks, and the oldest Palaeoproterozoic accretionary orogeny (e.g., Blackwelder, 1914; Hoffman, 1988; Ojakangas et al., 2001). The Animikie Basin, in particular during the depositional period of the Gunflint Formation, is interpreted to have formed in a back-arc basin (Hemming et al., 1995; Van Wyck and Johnson, 1997). Submarine volcanism caused black smoker-type submarine hydrothermal activity in deep parts of the Animikie back-arc basin, forming Kuroko-type volcanogenic massive sulphide deposits (De Matties, 1994). At the end of arc/back-arc activity, arc-related crustal blocks (Pembine-Wausau Terrane) collided with the Superior craton. This tectonic event changed the back-arc basin into a foreland basin (Ojakangas et al., 2001). The protoliths of the sedimentary rocks in the Gunflint Formation in the Animikie Group also changed rapidly, from Archean rocks and arc-type volcanic rocks to Penokean fold-and-thrust belt rocks (Schulz and Cannon, 2007). These tectonic settings supplied large quantities of diverse detrital materials to the Gunflint ocean, resulting in a unique and complex sedimentary environment with radical tectonically driven changes in sea level (e.g., Pufahl and Fralick, 2000; Fralick et al., 2017). The upper part of the Gunflint Formation contains evidence of a potential meteorite impact, such as tektite, accretionary lapilli tuff and stormy sediments. These features were caused by the Sudbury meteorite, which fell at 1850 ± 1 Ma (Addison et al., 2005). This age is consistent with the last deposition period of the Gunflint Formation. An ash layer near the base of the Rove Formation yielded a U-Pb zircon age of 1836 ± 5 Ma (Addison et al., 2005), and a volcanic ash layer in the Gunflint Formation yielded an U/Pb zircon age of 1878 ± 1.3 Ma (Fralick et al., 2002). Both radiogenic ages constrain upper and lower age limits of the Gunflint Formation.
The Gunflint Formation in Canada is one of the most well-known Palaeoproterozoic sedimentary formations that has not suffered high-grade metamorphism (e.g., Floran and Papike, 1975; Fralick et al., 2002; Alleon et al., 2016). The first report of Precambrian microfossils was from silicified stromatolites in this formation (Tyler and Barghoon, 1954). Following this find, several geochemical analyses have been performed on these microstructures and kerogen (e.g., House et al., 2000; Godfrey et al., 2013; Wacey et al. 2013, 2016; Williford et al., 2013). Geochemical studies on the organic matter in the Gunflint Formation have also been performed but only a few have focused on the nitrogen isotope compositions of the organic matter (e.g., Beaumont and Robert, 1999; Ishida et al., 2012; Godfrey et al., 2013). The sedimentary rocks of the Gunflint Formation were deposited in a tectonically active foreland or back-arc basin (e.g., Ojakangas, 1994; Fralick et al., 2002). In these environments, marine transgressions and regressions occurred frequently, and weathering inputs from terrestrial regions intensified, often in the same sedimentary basin.
Studied samples were collected from the following 6 sections (Fig. S-1): (1) Pass Lake section, (2) Blende Lake North section, (3) Current River section, (4) Terry Fox section, (5) Kakabeka Falls section and (6) Highway 590 east section. The lithology, mineralogy and chemistry of each section are described in Section 4. The occurrence of pyrobitumen was reported in the Lake Superior region (Mancuso, 1989), but we did not include samples with pyrobitumen in the following chemical and isotope analyses. Sampling was performed avoiding and removing the weathering surface carefully.

Figure S-1 Geological map of the Thunder Bay region (modified after Geological map of Thunder Bay district “Map 2065 ATIKOKAN-LAKEHEAD SHEET Geological Compilation Series”). Geological surveys were conducted in five sections: Pass Lake section; Blende Lake North section; Current River section; Terry Fox section; Kakabeka Falls and Highway 590 east section.
2. Methods
Mineralogical observations
Thin sections of the samples were observed under both a petrographic microscope (Olympus, BX50) and a scanning electron microscope with an energy-dispersive X-ray spectrometer (SEM/EDX) to determine the mineral assemblages and chemical compositions. A portion of the extracted kerogen was observed under a transmission electron microscope (TEM; JEOL, JEM-2010) to determine the carbon structures. The kerogen sample was mounted on a copper grid with a support membrane. The primary TEM electron beam was accelerated to 200 keV.
Elemental concentrations
Kerogen samples were extracted following the procedures of Vandenbroucke (2003). Detailed procedure is described in Ishida et al. (2012). Analyses of C, H, S and N concentrations were performed on both the extracted kerogen and bulk sample powder using an elemental analyser (EA; CarloErba EA1108). Approximately 3 milligrams of kerogen and 20 milligrams of powdered bulk rock samples were combusted. The reproducibility and accuracy of the analyses are within 0.1 % for each element.
The chemical compositions of 43 representative bulk rock samples were analysed using an inductivity coupled plasma mass spectrometer (ICP-MS). Approximately 100 grams of whole rock material was homogeneously powdered, and 20 grams of powder was analysed. The analyses were performed by Activation Laboratories Ltd., Canada. The concentrations of major and trace elements, including rare earth elements (REEs), were measured. The values of Post-Archean Australian Shale (PAAS; Pourmand et al., 2012) were used as the reference compositions for normalising the sample values.
Carbon and sulphur isotopic composition analyses
Carbon and sulphur stable isotope compositions were determined using an EA and a MAT252-ConfloIII system. Samples were combusted in the EA. The generated CO2 or SO2 gases were carried into the IRMS by a helium flow. The carbon isotope compositions are expressed relative to the international Pee Dee Belemnite standard (PDB; 13C/12C = 0.011237) in the standard delta (d) notation as follows:
The sulphur isotopic compositions are expressed relative to the international standard Canyon Diablo Troilite (CDT; 34S/32S=0.045005) in the standard delta (d) notation as follows:
The reproducibility and accuracy of these analyses are within 0.2 ‰ (1s).
Nitrogen isotope analyses of kerogen
The nitrogen isotope compositions were determined by applying both bulk and stepwise combustion methods. Because of the low concentrations of nitrogen in the kerogen, a high-sensitivity method using a static-type mass spectrometer was required. The Balzers QMG420 mass spectrometer at Osaka University, Japan, was used. The analytical protocols and performances are described in detail in Ishida et al. (2012) and references therein. For the bulk kerogen analysis, samples were heated in an oxygen atmosphere at 1200 ºC for 90 minutes to extract all gases at one time. In the stepwise analysis, the combustion involved 6 or 7 temperature steps, typically at 450, 475, 500, 525, 550, 575 and 1100 ºC. These temperature steps were chosen according to the results of Ishida et al. (2012) to capture the observed shift in the 15N/14N ratios at 575 to 600 ºC in examined kerogen samples. No measurement was performed at the first 450 ºC step, where surficial contamination, such as adsorbed nitrogen and/or ammonium ions, can dominate the sample gas. Samples were then combusted from 475 ºC to 575 ºC at 25 ºC steps to observe the shift in d15N values across the low and high temperature ranges. Samples were finally combusted at 1100 ºC to confirm the complete release of nitrogen from the samples. The nitrogen isotope compositions are expressed relative to atmospheric N2 (15N/14N = 0.00367) by the standard delta (d) notation as follows:
The d15N values and the amounts of released N and C for each temperature fraction were measured. The typical reproducibility (1s) of the isotope ratio for 1 ng of standard air is 0.7–0.9 ‰ (Ishida et al., 2012). The blank level of nitrogen was usually negligible (typically <0.01 ng at <1000 ºC) compared to the amount released from organic matter.
Unless otherwise noted, the error bars in this study represent 1s errors, and the elemental ratios represent compositional ratios.
3. Lithology, Mineralogy, and Whole Rock Chemistry
The data of bulk chemical compositions in the following sections are summarised in Table S-1.
Pass Lake section
Alternating layers of carbonate and sandstone, banded chert/carbonate, and black shale were observed (48.60289ºN, 88.779709ºW to 48.593987ºN, 88.768745ºW). In the southern part, banded chert and carbonate cropped out with alternations of iron-rich and silica-rich layers on the scale of a few centimetres. The iron-rich layers consisted of iron oxide with minor euhedral siderite (Fig. S-2b). Thin black shale layers were occasionally intercalated in iron layers. In the black shale, euhedral to semi-euhedral pyrite crystals were the major iron-bearing minerals. The SiO2 concentrations ranged from 54.2 to 97.2 % for the banded chert/carbonate and 8.3 to 26.3 % for the black shale layer. Carbonate, taconite and tuffaceous shale were dominant in the northern part (closer to Highway 11). These layers were stratigraphically lower than the banded chert/carbonate (Goodwin, 1956). In the banded carbonate section, dolomitic massive carbonate and granular chert layers were present in alternating layers. Most parts of the granular chert layer were silicified, and the components were composed of microcrystalline quartz. The silicification had resulted in variable SiO2 concentrations in the carbonate samples, ranging from 2.8 to 73.6 %. Well-preserved oolite was found in the microcrystalline quartz matrix (Fig. S-2a, sample #0721). The diameter of each grain was several hundreds of µm. The core material of the ooids was typically fragmented quartz, with occasional carbonate and minor pyrite. These core materials were concentrically covered by ferrodolomitic carbonate. The chemical compositions of the dolomitic carbonate were analysed by SEM/EDS. In general, their compositions were similar to the ferrodolomitic massive carbonate observed in this section. Diagenetic pyrite crystals were commonly observed throughout all examined layers. The Fe2O3 concentrations of the silicified carbonate layers ranged from 2.8 to 45.2 %.
Blende Lake North section
The outcrop was a few hundred metres northwest of the Pass Lake section (48.59729ºN, 88.790367ºW). It consisted of black shale with minor beds of ash layers and ferruginous chert. The chert layers contained haematitic iron oxide. The black shale layers contained alternations of tuff-rich layers and friable organic/clay-rich layers. High Fe2O3 contents (up to 45 %) were observed in samples #0301 to #0311. High Al2O3, TiO2 and K2O contents were observed in the black shale layers (samples #0301 to #0304), indicating the presence of abundant clay.
Current River section
The Current River section was a continuous exposure of the Gunflint Formation along a riverbed. The examined section ranged from Trowbridge Falls (48.489132°N, 89.188187°W) to the end of the North Current River (48.490452°N, 89.194267°W). The lithologies changed gradually from a lower black shale-dominated zone to an upper carbonate-sand zone (sample #0701 to #0712). The carbonate-sand zone consisted of alternating layers of carbonate and taconitic or cherty sandstone. Some carbonate layers consisted of millimetre-scale laminated and brecciated structures. The cherty sandstone consisted of a microcrystalline quartz matrix and coarser quartz grains with minor grains of carbonate. Euhedral to semi-euhedral ferrodolomite crystals were observed in the laminated layer with detrital quartz (sample #0704; Fig. S-2d). The massive carbonate layers often contained reworked veins associated with brecciation. In the middle layers of this section, shale and carbonate were observed. Layers of organic matter contained detrital lithic fragments, such as quartz and clay minerals (sample #0709; Fig. S-2d). Phosphoritic pebbles were found together with shale pebbles in the lowermost layer of carbonate. Phosphoritic pebbles consisted of calcium apatite, iron oxide, carbonate, quartz, pyrite, clay minerals and organic matter (sample #0713; Fig. S-2e). Framboidal pyrite crystals were observed around the phosphoritic pebbles but not inside of the pebbles (Fig. S-2e, 48.489521°N, 89.188225°W). The framboidal pyrites were approximately 10 µm in diameter and contain less than 1 wt. % arsenic, whereas the euhedral pyrite did not contain arsenic. In the lower layers, black shale was dominant and consisted of fine grains of detrital quartz, illite, chlorite, and organic matter with minor carbonate grains (sample #0715; Fig. S-2f). Silicification was significant in all samples, with variable SiO2 concentrations ranging from 15.2 to 74.9 %. Overall, the Fe2O3 concentrations ranged from 8.9 to 30.3 %, suggesting high Fe fluxes during deposition.
Terry Fox section
The Terry Fox section covered the Gunflint and Rove Formations (48.484425°N, 89.167976°W). The stratigraphic top part of the Gunflint Formation was exposed here and consisted of dolomite to ferrodolomite layers and a granular chert layer (Fig. S-2g). The dolomitic layers consisted of euhedral dolomite and anhedral quartz with minor organic matter with euhedral pyrite crystals (Fig. S-2i). The chert layer consisted of microcrystalline quartz grains and a quartz matrix (Fig. S-2h). A Logan diabase sill formed a cliff exposure of the Rove Formation (Floran and Papike, 1975). The Fe2O3 concentrations (6.2 to 13 %) in the carbonate samples were less than those of other carbonates. Silicification was also significant, and the SiO2 concentrations were variable (18.3 to 96.7 %).
Kakabeka Falls and Highway 590 east sections
Banded cherts with iron-rich and carbonaceous layers were observed near the parking area at the park entrance (48.40279°N, 89.623459°W). Boulders that were plausibly derived from this outcrop were found outside of the park and were analysed in this study. The iron-rich layers consisted of iron oxide, minor euhedral siderite, quartz, chlorite and organic matter (Fig. S-2j). Those samples had high Fe2O3 concentrations of up to 40 %. The chert layer consisted of equigranular quartz and features high SiO2 concentrations (72.9 to 96.6 %). The P2O5 concentrations of the iron-rich layers were higher than those of the chert layers. At the intersection of Highways 11 and 590, silicified stromatolites, a chert layer and a minor black shale layer were observed (48.404877°N, 89.632538°W). The black shale layer consisted of fine grains of detrital quartz and organic matter with minor pyrite grains.

Figure S-2 Thin section images of representative samples. Scale bars in each photo are 1 millimetre unless otherwise noted. (a) Sample #0721: Oolite from Pass Lake section. Fe-rich dolomite covers lithic core concentrically in a quartz matrix. Inserted image: Back-scattered electron (BSE) image of ooid grain. Pyrite is contained in the rim of an ooid grain. Ooid grains contain tiny haematite crystals in the carbonate rims. Scale bar is 200 µm. (b) Sample #0722: BIF iron layer from Pass Lake section. The upper iron-rich layer consists of haematite in a microcrystalline quartz matrix. The lower chert layer consists of microcrystalline quartz. (c) Sample #0704: Sandstone from the Current River section. Sandy grains consist of microcrystalline quartz. Each diameter is approximately 50 to 100 µm. Tiny ferrodolomitic carbonate grains surround the grains. (d) Sample #0709: Organic matter (OM) is observed with detrital lithic fragments, such as quartz and clay minerals. The matrix is carbonate mud. (e) Sample #0713: Phosphorite pebble from the Current River section. The matrix consists of ferrodolomite and detrital quartz. The phosphorite pebbles consist of an apatite matrix, fragments of quartz, clay minerals, OM, and pyrite. Inserted image: Framboidal pyrite found around the phosphorite (white arrow). Both euhedral and framboidal pyrite crystals are observed. Arsenic is present in the framboidal pyrite as a minor component (less than 2 wt. %), but not in the euhedral pyrite. Scale bar is 50 µm. (f) Sample #0715: Finely laminated sedimentary layers with OM. The matrix consists of quartz and chlorite. Carbonate minerals and pyrite are scarce. (g) Sample #0726: Ferrodolomitic layer in BIF from the Terry Fox section. Euhedral dolomite crystals are present in a microcrystalline quartz matrix. Inserted image: BSE image of single ferrodolomite crystal. Outer rim of crystal is richer in iron than the crystal interior. Scale bar is 100 µm. (h) Sample #0726: Chert layer in a BIF sample. Microcrystalline quartz grains with fibrous cement are observed in a quartz matrix. (i) Sample #0725: Black chert from the Terry Fox section (reflected light). OM is present in a chert matrix. Pyrite crystals are observed in the OM layer. (j) Sample #0601: Iron layer from the Kakabeka Falls section. Matrix consists of iron-rich silicate. OM exists as a laminated structure. Minor siderites were observed with no relation to the stratigraphic layer.
Table S-1 Summary of major and trace element concentrations of bulk rock samples. Eu/Eu* is defined as Eu/0.5(Gd+Sm), Pr/Pr* is defined as Pr/0.5(Ce+Nd), and Ce/Ce* is defined as Ce/0.5(La+Pr). Values are normalised by PAAS reported by Pourmand et al. (2012).
sample ID | SiO2 | Al2O3 | Fe2O3 | MnO | MgO | CaO | Na2O | K2O | TiO2 | P2O5 |
wt. % | ||||||||||
0716 | 97.74 | 0.24 | 0.85 | 0.009 | 0.02 | 0.15 | 0.08 | 0.12 | 0.009 | 0.02 |
0718 | 43.08 | 0.19 | 6.18 | 1.341 | 7.98 | 15.83 | 0.11 | 0.05 | 0.005 | 0.01 |
0719 | 74.27 | 0.13 | 2.88 | 0.537 | 3.91 | 7.57 | 0.09 | 0.04 | 0.001 | 0.01 |
0720 | 68.08 | 0.13 | 5.16 | 0.715 | 4.34 | 8.71 | 0.08 | 0.07 | 0.005 | 0.01 |
0721 | 73.66 | 0.11 | 4.98 | 0.766 | 3.06 | 6.87 | 0.01 | 0.01 | 0.005 | 0.01 |
0722 | 97.15 | 0.31 | 0.69 | 0.017 | 0.01 | 1.07 | 0.05 | 0.04 | 0.001 | 0.02 |
0723 | 54.19 | 0.11 | 21.39 | 1.388 | 3.55 | 2.93 | 0.01 | 0.06 | 0.009 | 0.53 |
0301 | 63.37 | 14.67 | 8.31 | 0.082 | 1.89 | 0.18 | 0.11 | 5.74 | 1.167 | 0.09 |
0302 | 48.44 | 15.94 | 15.22 | 0.23 | 2.1 | 0.99 | 0.08 | 8.14 | 2.523 | 0.34 |
0303 | 45.29 | 5.22 | 26.32 | 1.17 | 3.42 | 1.93 | 0.02 | 0.5 | 0.255 | 0.1 |
0304 | 55.12 | 14.02 | 15.48 | 0.212 | 2.66 | 0.57 | 0.07 | 3.6 | 0.659 | 0.06 |
0305 | 16.74 | 1.27 | 28.04 | 2.83 | 6.82 | 12.75 | 0.13 | 0.06 | 0.012 | 1.23 |
0306 | 2.80 | 0.94 | 43.48 | 3.567 | 6.96 | 6.75 | 0.24 | 0.06 | 0.006 | 0.33 |
0307 | 51.43 | 0.04 | 24.87 | 1.602 | 2.45 | 2.58 | 0.02 | 0.01 | 0.003 | 0.75 |
0308 | 62.17 | 0.22 | 17.06 | 1.31 | 2.54 | 2.68 | 0.2 | 0.04 | 0.007 | 0.15 |
0309 | 5.04 | 1.43 | 45.22 | 3.325 | 6.88 | 5.49 | 0.01 | 0.01 | 0.003 | 0.34 |
0310 | 70.31 | 0.08 | 16.22 | 0.9 | 1.74 | 1.1 | 0.01 | 0.01 | 0.003 | 0.16 |
0311 | 82.50 | 0.1 | 9.69 | 0.594 | 0.98 | 0.56 | 0.22 | 0.04 | 0.007 | 0.07 |
0701 | 50.14 | 2.22 | 25.99 | 1.067 | 2.93 | 1.41 | 0.02 | 0.02 | 0.091 | 0.13 |
0702 | 71.07 | 5.23 | 14.79 | 0.107 | 2.66 | 1.19 | 0.04 | 0.06 | 0.222 | 0.04 |
0703 | 52.52 | 2.73 | 21.16 | 0.613 | 4.07 | 4.36 | 0.08 | 0.03 | 0.132 | 0.03 |
0704 | 41.28 | 1.47 | 27.22 | 0.836 | 4.29 | 4.85 | 0.12 | 0.04 | 0.05 | 0.07 |
0705 | 44.56 | 3.06 | 30.28 | 0.668 | 3.55 | 1.29 | 0.03 | 0.03 | 0.124 | 0.13 |
0706 | 45.52 | 2.04 | 13.18 | 0.438 | 5.39 | 12.47 | 0.01 | 0.07 | 0.07 | 0.09 |
0707 | 62.39 | 2.93 | 16.18 | 0.463 | 3.19 | 4.05 | 0.02 | 0.01 | 0.225 | 0.03 |
0708 | 26.51 | 2.98 | 29.33 | 0.87 | 6.96 | 8.26 | 0.09 | 0.06 | 0.083 | 0.09 |
0709 | 65.35 | 4.7 | 19.08 | 0.232 | 2.74 | 0.74 | 0.12 | 0.19 | 0.2 | 0.11 |
0710 | 74.91 | 5.75 | 10.75 | 0.03 | 1.83 | 0.47 | 0.1 | 1.22 | 0.355 | 0.07 |
0711 | 15.22 | 2.16 | 15.78 | 0.747 | 9.15 | 22.39 | 0.12 | 0.3 | 0.113 | 0.02 |
0712 | 75.36 | 5.53 | 10.47 | 0.071 | 1.7 | 0.66 | 0.07 | 1.73 | 0.29 | 0.05 |
0713 | 39.03 | 1.46 | 18.8 | 0.792 | 5.68 | 10.88 | 0.09 | 0.12 | 0.055 | 0.45 |
0714 | 50.18 | 4.99 | 23.59 | 0.458 | 3.53 | 2.95 | 0.03 | 0.62 | 0.164 | 0.06 |
0715 | 69.70 | 11.04 | 8.86 | 0.01 | 1.72 | 0.14 | 0.01 | 5.22 | 0.641 | 0.08 |
0724 | 18.30 | 0.29 | 11.3 | 1.524 | 10.85 | 22.79 | 0.03 | 0.01 | 0.009 | 0.54 |
0725 | 76.26 | 5.49 | 12.97 | 0.023 | 2.67 | 0.18 | 0.06 | 0.07 | 0.139 | 0.08 |
0726 | 44.25 | 1.91 | 6.22 | 0.484 | 8.91 | 14.81 | 0.06 | 0.55 | 0.065 | 0.03 |
0729 | 96.74 | 0.16 | 1.29 | 0.024 | 0.1 | 1.08 | 0.45 | 0.06 | 0.007 | 0.02 |
0730 | 96.60 | 0.13 | 0.68 | 0.017 | 0.01 | 0.69 | 0.14 | 0.03 | 0.005 | 0.03 |
0601 | 27.53 | 2.95 | 39.9 | 0.46 | 5.3 | 1.43 | 0.14 | 0.14 | 0.053 | 0.33 |
0602 | 96.59 | 0.34 | 1.79 | 0.019 | 0.08 | 0.03 | 0.13 | 0.08 | 0.009 | 0.02 |
0603 | 47.39 | 0.64 | 30.08 | 0.534 | 2.58 | 1.58 | 0.13 | 0.11 | 0.026 | 0.36 |
0604 | 72.91 | 0.31 | 16.58 | 0.352 | 1.12 | 0.78 | 0.04 | 0.06 | 0.01 | 0.17 |
0605 | 84.92 | 0.09 | 13.26 | 0.014 | 0.06 | 0.01 | 0.08 | 0.01 | 0.005 | 0.02 |
sample ID | La | Ce | Pr | Nd | Sm | Eu | Gd | Tb | Dy | Y | Ho | Er | Tm | Yb | Lu | SREE | Pr/Sm | Eu/Eu* | Ce/Ce* | Pr/Pr* |
ppm | (PAAS normalised) | |||||||||||||||||||
0716 | 1 | 3.2 | 0.18 | 0.6 | 0.1 | 0.05 | 0.1* | 0.1* | 0.1* | 2* | 0.1* | 0.1* | 0.05* | 0.1* | 0.04* | 5.13 | 1.22 | 0.87 | 1.81 | 0.68 |
0718 | 8.5 | 18.3 | 2.15 | 9.3 | 2.4 | 1.04 | 2.7 | 0.4 | 2.5 | 18 | 0.5 | 1.2 | 0.14 | 0.7 | 0.08 | 67.91 | 0.61 | 2.22 | 1.03 | 0.93 |
0719 | 5.7 | 11.5 | 1.27 | 5.1 | 1.3 | 0.55 | 1.5 | 0.2 | 1.2 | 7 | 0.2 | 0.6 | 0.08 | 0.4 | 0.05 | 36.65 | 0.66 | 2.24 | 1.03 | 0.94 |
0720 | 5.1 | 10 | 1.19 | 4.7 | 1.2 | 0.51 | 1.3 | 0.2 | 1.5 | 9 | 0.3 | 0.9 | 0.12 | 0.6 | 0.08 | 36.7 | 0.67 | 2.18 | 0.98 | 0.98 |
0721 | 3.1 | 6.1 | 0.85 | 2.7 | 0.7 | 0.31 | 0.9 | 0.2 | 1 | 7 | 0.2 | 0.7 | 0.1 | 0.5 | 0.07 | 24.43 | 0.82 | 1.78 | 0.90 | 1.18 |
0722 | 1.9 | 4.1 | 0.39 | 1.5 | 0.3 | 0.14 | 0.4 | 0.1* | 0.3 | 2* | 0.1* | 0.2 | 0.05* | 0.1* | 0.04* | 9.23 | 0.88 | 1.73 | 1.15 | 0.89 |
0723 | 4.9 | 11.9 | 1.09 | 4.1 | 0.9 | 0.28 | 1.1 | 0.2 | 0.9 | 7 | 0.2 | 0.5 | 0.07 | 0.3 | 0.04* | 33.44 | 0.82 | 1.41 | 1.24 | 0.88 |
0301 | 47.5 | 80.7 | 6.49 | 17.2 | 2.5 | 0.6 | 2 | 0.4 | 2.5 | 16 | 0.5 | 1.7 | 0.27 | 1.9 | 0.27 | 180.53 | 1.76 | 1.25 | 1.07 | 0.93 |
0302 | 16.9 | 50.9 | 6.47 | 31.4 | 8.9 | 2.37 | 8.4 | 1.2 | 6.5 | 33 | 1.3 | 3.6 | 0.55 | 3.5 | 0.5 | 175.49 | 0.49 | 1.48 | 1.13 | 0.90 |
0303 | 12.1 | 26.6 | 2.79 | 11.2 | 2.5 | 0.67 | 2.3 | 0.3 | 1.8 | 11 | 0.3 | 1 | 0.14 | 0.9 | 0.13 | 73.73 | 0.76 | 1.54 | 1.10 | 0.91 |
0304 | 26.6 | 42.7 | 3.37 | 9.8 | 1.6 | 0.39 | 1.4 | 0.3 | 2 | 14 | 0.4 | 1.4 | 0.23 | 1.5 | 0.22 | 105.91 | 1.43 | 1.19 | 1.04 | 0.89 |
0305 | 21.8 | 62.5 | 8.43 | 36.6 | 8.3 | 2.36 | 8.1 | 1.1 | 5.7 | 36 | 1 | 2.5 | 0.29 | 1.5 | 0.2 | 196.38 | 0.69 | 1.59 | 1.07 | 0.98 |
0306 | 7.9 | 17.2 | 1.62 | 6.1 | 1.4 | 0.4 | 1.5 | 0.3 | 1.6 | 11 | 0.3 | 0.9 | 0.13 | 0.7 | 0.1 | 51.15 | 0.78 | 1.32 | 1.16 | 0.89 |
0307 | 3.3 | 7.7 | 0.69 | 2.6 | 0.6 | 0.18 | 0.6 | 0.1* | 0.5 | 5 | 0.1* | 0.3 | 0.05* | 0.1* | 0.04* | 21.47 | 0.78 | 1.54 | 1.23 | 0.87 |
0308 | 2.5 | 5.7 | 0.49 | 1.7 | 0.4 | 0.11 | 0.4 | 0.1* | 0.4 | 3 | 0.1* | 0.3 | 0.05* | 0.3 | 0.04* | 15.3 | 0.83 | 1.18 | 1.24 | 0.88 |
0309 | 10.5 | 24.3 | 2.32 | 8.6 | 1.9 | 0.46 | 1.9 | 0.3 | 2 | 15 | 0.4 | 1.2 | 0.16 | 1 | 0.14 | 70.18 | 0.83 | 1.27 | 1.19 | 0.90 |
0310 | 2.3 | 5.6 | 0.49 | 1.8 | 0.4 | 0.12 | 0.4 | 0.1* | 0.3 | 3 | 0.1* | 0.2 | 0.05* | 0.1* | 0.04* | 14.61 | 0.83 | 1.29 | 1.27 | 0.86 |
0311 | 1 | 2.5 | 0.2 | 0.7 | 0.2 | 0.05 | 0.1* | 0.1* | 0.1* | 2* | 0.1* | 0.1* | 0.05* | 0.1* | 0.04* | 4.65 | 0.68 | 0.72 | 1.34 | 0.84 |
0701 | 2.9 | 8 | 0.76 | 2.9 | 0.6 | 0.15 | 0.5 | 0.1* | 0.4 | 3 | 0.1* | 0.3 | 0.05* | 0.2 | 0.04* | 19.71 | 0.86 | 1.28 | 1.30 | 0.89 |
0702 | 15.1 | 27.8 | 1.65 | 4.1 | 0.6 | 0.13 | 0.3 | 0.1* | 0.4 | 3 | 0.1* | 0.3 | 0.05* | 0.3 | 0.05 | 53.73 | 1.87 | 1.11 | 1.26 | 0.77 |
0703 | 3.4 | 8.5 | 0.73 | 2.4 | 0.5 | 0.13 | 0.4 | 0.1* | 0.4 | 3 | 0.1* | 0.3 | 0.05* | 0.3 | 0.04* | 20.06 | 0.99 | 1.24 | 1.30 | 0.90 |
0704 | 2.7 | 6.5 | 0.58 | 1.9 | 0.4 | 0.11 | 0.3 | 0.1* | 0.3 | 2* | 0.1* | 0.2 | 0.05* | 0.1* | 0.04* | 12.99 | 0.98 | 1.18 | 1.25 | 0.92 |
0705 | 8.1 | 17.8 | 1.45 | 4.6 | 0.8 | 0.2 | 0.7 | 0.1* | 0.4 | 3 | 0.1* | 0.2 | 0.05* | 0.2 | 0.04* | 37.45 | 1.23 | 1.42 | 1.24 | 0.88 |
0706 | 7.8 | 16.5 | 1.52 | 5.1 | 1 | 0.25 | 0.8 | 0.1* | 0.6 | 4 | 0.1* | 0.3 | 0.05* | 0.3 | 0.04* | 38.17 | 1.03 | 1.52 | 1.15 | 0.93 |
0707 | 3.4 | 7.8 | 0.54 | 2 | 0.4 | 0.11 | 0.4 | 0.1* | 0.5 | 4 | 0.1* | 0.3 | 0.05* | 0.3 | 0.05 | 19.8 | 0.92 | 1.18 | 1.36 | 0.75 |
0708 | 4.1 | 10.6 | 1.12 | 4.4 | 1 | 0.29 | 1.1 | 0.1* | 0.7 | 5 | 0.1* | 0.3 | 0.05* | 0.2 | 0.04* | 28.81 | 0.76 | 1.76 | 1.19 | 0.93 |
0709 | 5.9 | 14.2 | 1.37 | 4.6 | 0.9 | 0.2 | 0.7 | 0.1* | 0.4 | 2* | 0.1* | 0.3 | 0.05* | 0.2 | 0.04* | 28.77 | 1.03 | 1.31 | 1.20 | 0.95 |
0710 | 6.8 | 14 | 1.22 | 3.8 | 0.7 | 0.19 | 0.5 | 0.1* | 0.5 | 3 | 0.1* | 0.7 | 0.05* | 0.3 | 0.05 | 31.76 | 1.18 | 1.48 | 1.16 | 0.92 |
0711 | 10.1 | 21.2 | 1.92 | 6.6 | 1.4 | 0.42 | 1.3 | 0.2 | 1.3 | 12 | 0.3 | 0.7 | 0.09 | 0.5 | 0.07 | 58.1 | 0.93 | 1.63 | 1.16 | 0.91 |
0712 | 5.6 | 11.6 | 1.03 | 3.2 | 0.6 | 0.19 | 0.5 | 0.1* | 0.5 | 4 | 0.1* | 0.3 | 0.05* | 0.3 | 0.04* | 27.82 | 1.16 | 1.63 | 1.16 | 0.93 |
0713 | 17.4 | 38.1 | 3.16 | 10.5 | 2.1 | 0.65 | 2 | 0.3 | 1.6 | 13 | 0.3 | 0.8 | 0.11 | 0.6 | 0.08 | 90.7 | 1.02 | 1.68 | 1.23 | 0.87 |
0714 | 18.2 | 30.4 | 2.51 | 7.3 | 1.2 | 0.23 | 0.8 | 0.1* | 0.4 | 2* | 0.1* | 0.3 | 0.05* | 0.2 | 0.04* | 61.54 | 1.42 | 1.22 | 1.05 | 0.92 |
0715 | 12.7 | 22.6 | 2.13 | 6.7 | 1.2 | 0.3 | 0.9 | 0.2 | 0.9 | 7 | 0.2 | 0.7 | 0.12 | 0.8 | 0.13 | 56.58 | 1.20 | 1.28 | 1.03 | 0.96 |
0724 | 8.1 | 17.7 | 1.51 | 5.2 | 1.1 | 0.34 | 1.4 | 0.2 | 1.4 | 12 | 0.3 | 0.8 | 0.1 | 0.6 | 0.07 | 50.82 | 0.93 | 1.53 | 1.21 | 0.88 |
0725 | 0.8 | 2.4 | 0.28 | 1.3 | 0.3 | 0.1 | 0.4 | 0.1* | 0.6 | 2* | 0.1* | 0.4 | 0.06 | 0.3 | 0.05 | 6.99 | 0.63 | 1.23 | 1.19 | 0.89 |
0726 | 3.8 | 8 | 0.84 | 3.3 | 0.8 | 0.26 | 0.9 | 0.2 | 1 | 7 | 0.2 | 0.6 | 0.08 | 0.4 | 0.06 | 27.44 | 0.71 | 1.40 | 1.08 | 0.92 |
0729 | 0.4 | 0.6* | 0.07 | 0.5 | 0.2 | 0.05 | 0.2 | 0.1* | 0.2 | 2* | 0.1* | 0.1* | 0.05* | 0.2 | 0.04* | 1.82 | 0.24 | 0.72 | 0.86 | 0.68 |
0730 | 0.3* | 0.6* | 0.09 | 0.7 | 0.3 | 0.08 | 0.3 | 0.1* | 0.2 | 2* | 0.1* | 0.1* | 0.05* | 0.2 | 0.04* | 1.87 | 0.20 | 0.99 | 0.87 | 0.69 |
0601 | 7.8 | 17.5 | 1.76 | 6.3 | 1.3 | 0.41 | 1.5 | 0.2 | 1.4 | 10 | 0.3 | 0.8 | 0.1 | 0.6 | 0.09 | 50.06 | 0.92 | 1.67 | 1.14 | 0.94 |
0602 | 0.3* | 0.6* | 0.06 | 0.2* | 0.1 | 0.05 | 0.1* | 0.1* | 0.1 | 2* | 0.1* | 0.1* | 0.05* | 0.1* | 0.04* | 0.31 | 0.41 | 0.87 | 1.08 | 0.97 |
0603 | 11.3 | 30.2 | 2.71 | 10.3 | 2.2 | 0.45 | 2.5 | 0.1* | 0.7 | 15 | 0.1* | 0.4 | 0.06 | 0.4 | 0.12 | 76.34 | 0.84 | 1.46 | 1.31 | 0.86 |
0604 | 4 | 11.6 | 1.03 | 3.2 | 0.6 | 0.16 | 0.6 | 0.1* | 0.7 | 4 | 0.1* | 0.4 | 0.06 | 0.4 | 0.05 | 26.8 | 1.16 | 1.37 | 1.37 | 0.93 |
0605 | 1.3 | 3.9 | 0.22 | 0.9 | 0.2 | 0.05 | 0.1* | 0.1* | 0.3 | 2* | 0.1* | 0.2 | 0.05* | 0.2 | 0.04* | 7.27 | 0.75 | 0.72 | 1.74 | 0.63 |
sample ID | Sc | As | Hf | Th | U | Zr | Cr | Co | Cd | Cu | Ni | Zn | Ag | Pb | V | Ba | Sr | Ga | Ge | Rb | Mo |
ppm | |||||||||||||||||||||
0716 | 1* | 5 | 0.2* | 0.3 | 0.1* | 12 | 20* | 1* | 0.5 | 5 | 1* | 1* | 0.3* | 5* | 6 | 31 | 5 | 1* | 4 | 3 | 2* |
0718 | 1* | 5 | 0.2* | 0.1* | 0.3 | 4 | 20* | 1* | 0.6 | 4 | 3 | 2 | 0.9 | 5* | 32 | 15 | 12 | 1* | 1* | 2* | 2* |
0719 | 1* | 5 | 0.2* | 0.1* | 0.4 | 9 | 20* | 1* | 0.5 | 9 | 3 | 1* | 0.6 | 5* | 28 | 20 | 10 | 1* | 2 | 2* | 2* |
0720 | 1* | 5 | 0.2* | 0.1* | 0.1* | 5 | 20* | 1* | 0.6 | 7 | 4 | 49 | 0.5 | 14 | 16 | 15 | 12 | 1* | 1* | 2* | 2* |
0721 | 1* | 43 | 0.2* | 0.1* | 0.4 | 4 | 20* | 9 | 0.5 | 11 | 5 | 10 | 0.5 | 6 | 11 | 24 | 8 | 1* | 2 | 2* | 2* |
0722 | 1* | 5 | 0.2* | 0.1* | 0.3 | 4 | 20* | 1* | 0.5 | 8 | 3 | 3 | 0.3* | 5* | 5 | 114 | 11 | 1* | 1* | 2* | 2* |
0723 | 1* | 5 | 0.2* | 0.1* | 0.5 | 4 | 20* | 3 | 1.7 | 6 | 7 | 4 | 0.7 | 7 | 6 | 21 | 17 | 1* | 1* | 2* | 2* |
0301 | 19 | 5 | 4.4 | 9.8 | 2.4 | 169 | 150 | 8 | 0.7 | 127 | 29 | 18 | 0.3* | 8 | 142 | 466 | 33 | 23 | 4 | 190 | 2* |
0302 | 23 | 6 | 5.3 | 7.2 | 3.3 | 175 | 70 | 28 | 1.1 | 208 | 64 | 19 | 0.5 | 10 | 340 | 1620 | 18 | 17 | 3 | 11 | 27 |
0303 | 1* | 14 | 0.8 | 2.7 | 1.7 | 33 | 40 | 6 | 0.5 | 3 | 1* | 1* | 0.3* | 5* | 56 | 64 | 11 | 13 | 3 | 18 | 2* |
0304 | 15 | 5 | 3 | 7.8 | 3.1 | 124 | 70 | 18 | 1.3 | 22 | 44 | 23 | 0.4 | 5* | 131 | 333 | 20 | 23 | 4 | 124 | 4 |
0305 | 2 | 16 | 0.2* | 0.3 | 3 | 4 | 20* | 4 | 1.6 | 6 | 15 | 6 | 1.3 | 13 | 72 | 19 | 65 | 4 | 1* | 2* | 2* |
0306 | 1* | 9 | 0.2* | 0.1* | 2.3 | 4 | 20* | 4 | 2.1 | 11 | 13 | 8 | 1.9 | 12 | 76 | 15 | 25 | 3 | 1* | 2* | 2* |
0307 | 1* | 5 | 0.2* | 0.1* | 0.2 | 4 | 20* | 2 | 1.6 | 3 | 2 | 4 | 0.7 | 8 | 5 | 10 | 16 | 1* | 1* | 2* | 2* |
0308 | 1* | 5 | 0.2* | 0.1* | 0.2 | 4 | 20* | 2 | 1.4 | 6 | 5 | 4 | 0.7 | 5* | 5 | 22 | 14 | 1* | 2 | 2* | 2* |
0309 | 2 | 5 | 0.2* | 0.2 | 0.9 | 4 | 20* | 4 | 2.2 | 5 | 9 | 6 | 1.5 | 12 | 28 | 11 | 25 | 3 | 1* | 2* | 2* |
0310 | 1* | 5 | 0.2* | 0.1* | 0.1* | 4 | 20* | 2 | 1.3 | 5 | 3 | 4 | 0.5 | 6 | 5 | 13 | 17 | 1* | 2 | 2* | 2* |
0311 | 1* | 5 | 0.2* | 0.1* | 0.1* | 4 | 20* | 3 | 0.8 | 9 | 4 | 3 | 0.3* | 5* | 5 | 14 | 5 | 1* | 1* | 2* | 2* |
0701 | 3 | 5 | 0.6 | 1.9 | 0.2 | 37 | 20* | 6 | 1.8 | 1* | 9 | 7 | 0.9 | 7 | 24 | 21 | 11 | 5 | 2 | 2* | 2* |
0702 | 4 | 5 | 1.6 | 3.6 | 0.5 | 74 | 40 | 9 | 1.2 | 9 | 15 | 52 | 0.3* | 5* | 47 | 25 | 7 | 12 | 6 | 5 | 2* |
0703 | 3 | 5 | 0.8 | 2.4 | 0.5 | 40 | 20* | 6 | 1.7 | 6 | 9 | 10 | 0.4 | 5* | 24 | 18 | 13 | 7 | 3 | 2* | 2* |
0704 | 1* | 5 | 0.4 | 1 | 0.3 | 19 | 20* | 6 | 1.8 | 4 | 8 | 6 | 0.8 | 9 | 10 | 14 | 14 | 4 | 2 | 2* | 2* |
0705 | 4 | 5 | 1 | 2.8 | 0.4 | 53 | 20* | 12 | 1.8 | 3 | 11 | 10 | 0.5 | 8 | 29 | 23 | 9 | 8 | 3 | 2* | 2* |
0706 | 3 | 15 | 0.5 | 1.4 | 0.8 | 20 | 20* | 21 | 1.1 | 17 | 13 | 8 | 0.5 | 12 | 25 | 18 | 45 | 4 | 2 | 2* | 2* |
0707 | 4 | 5 | 1.4 | 3.3 | 0.6 | 59 | 40 | 6 | 1.4 | 4 | 11 | 10 | 0.4 | 5 | 29 | 29 | 21 | 7 | 4 | 2* | 2* |
0708 | 6 | 5 | 0.4 | 1.9 | 0.4 | 24 | 20* | 7 | 1.9 | 5 | 12 | 10 | 0.6 | 6 | 27 | 11 | 14 | 6 | 3 | 2* | 2* |
0709 | 4 | 5 | 1.3 | 4.1 | 0.9 | 60 | 30 | 10 | 1.6 | 18 | 15 | 13 | 0.4 | 5* | 34 | 42 | 9 | 9 | 3 | 5 | 2* |
0710 | 3 | 5 | 2.1 | 5.3 | 1 | 100 | 40 | 9 | 1 | 21 | 19 | 14 | 0.3* | 5* | 42 | 131 | 13 | 12 | 5 | 22 | 2* |
0711 | 6 | 5 | 0.8 | 1.9 | 0.8 | 36 | 20* | 1* | 1.1 | 4 | 10 | 7 | 0.6 | 7 | 31 | 37 | 51 | 6 | 1* | 6 | 2* |
0712 | 3 | 5 | 2 | 3.5 | 1.4 | 78 | 50 | 6 | 0.9 | 13 | 17 | 13 | 0.3* | 5* | 54 | 167 | 14 | 13 | 5 | 35 | 2* |
0713 | 3 | 22 | 0.9 | 1.5 | 1.7 | 37 | 20* | 19 | 1.3 | 9 | 15 | 5 | 0.8 | 11 | 18 | 19 | 24 | 5 | 2 | 2* | 2* |
0714 | 14 | 5 | 0.7 | 2.4 | 1.2 | 33 | 20* | 11 | 1.7 | 16 | 21 | 11 | 0.7 | 7 | 84 | 74 | 16 | 11 | 4 | 14 | 2* |
0715 | 11 | 5 | 3.3 | 9.7 | 1.7 | 133 | 80 | 6 | 0.7 | 21 | 28 | 18 | 0.3* | 5* | 87 | 493 | 28 | 13 | 4 | 122 | 2* |
0724 | 1 | 16 | 0.2* | 0.2 | 1.3 | 4 | 20* | 1* | 1.2 | 5 | 8 | 2 | 0.7 | 6 | 21 | 15 | 42 | 1* | 1* | 2* | 2* |
0725 | 4 | 38 | 0.8 | 2 | 0.8 | 35 | 20* | 20 | 1 | 14 | 49 | 17 | 0.5 | 16 | 45 | 18 | 13 | 13 | 4 | 2* | 2* |
0726 | 4 | 5 | 0.4 | 1 | 1.2 | 23 | 20* | 1* | 0.5 | 9 | 10 | 5 | 0.3* | 5* | 46 | 62 | 56 | 3 | 1* | 11 | 2* |
0729 | 1* | 14 | 0.2* | 0.1* | 0.6 | 4 | 20* | 3 | 0.5 | 8 | 4 | 21 | 0.3* | 5* | 6 | 16 | 6 | 1* | 2 | 2* | 2* |
0730 | 1* | 5 | 0.3 | 0.1* | 0.3 | 4 | 20* | 1* | 0.5 | 6 | 2 | 1* | 0.3* | 5* | 5 | 8 | 3 | 1* | 2 | 2* | 2* |
0601 | 4 | 12 | 0.3 | 0.8 | 1.5 | 11 | 20* | 4 | 2.1 | 7 | 18 | 19 | 0.5 | 15 | 60 | 35 | 23 | 5 | 2 | 3 | 2* |
0602 | 1* | 5 | 0.2* | 0.2 | 0.1* | 5 | 20* | 3 | 0.5 | 13 | 3 | 47 | 0.3* | 9 | 5 | 76 | 14 | 1* | 6 | 3 | 2* |
0603 | 1* | 9 | 0.2* | 0.6 | 0.3 | 11 | 20* | 4 | 1.9 | 9 | 9 | 24 | 0.4 | 12 | 13 | 29 | 28 | 1* | 2 | 2* | 2* |
0604 | 1* | 14 | 2.7 | 0.3 | 0.3 | 99 | 20* | 3 | 1.3 | 11 | 7 | 27 | 0.3* | 6 | 5 | 15 | 12 | 2 | 5 | 2* | 2* |
0605 | 1* | 2 | 0.2* | 0.1* | 0.1* | 4 | 20* | 3 | 1.1 | 7 | 4 | 2 | 0.3* | 5* | 9 | 5 | 2 | 1* | 3 | 2* | 2* |
* : below detection limit
Download in Excel4. Chemical and Light Element Isotope Compositions of Bulk Rock and Kerogen
Light element concentrations in bulk rock and kerogen
The total organic carbon (TOC), carbonate carbon (Ccarb), total carbon (TC), total nitrogen (TN), and pyrite sulphur (Spyr) concentrations in the bulk rock samples are summarised in Table 1. The TOC and Spyr concentrations ranged from 0.0 to 8.9 wt. % and from 0.0 to 1.1 wt. %, respectively. The TN concentrations of all bulk rock samples were below detection limit (<0.1 wt. %). In Figure S-3a, the TOC concentrations were plotted against the Spyr concentrations. Generally, the shallower lithofacies, such as the stromatolite and sandstone facies, exhibited low TOC and Spyr concentrations, whereas the deeper lithofacies, such as the carbonate and shale facies, exhibited a range of concentrations.
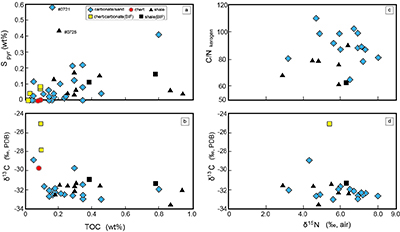
Figure S-3 Correlation diagrams of geochemical data from the examined samples. Data are sorted based on lithology: blue rhombs = carbonate, red circle = chert, black triangle = shale, and square = BIF (yellow: chert/carbonate, black: shale containing iron layer). (a) Correlation diagram of total organic carbon content (TOC) versus pyrite sulphur content (Spyr). A positive correlation exists, except for two samples that contain higher amounts of sulphur (samples #0721 and #0725). (b) Correlation diagram of TOC versus d13C values for organic matter. No correlation is observed. Correlation diagrams of (c) kerogen C/N ratios vs. d15N values and (d) d13C vs. d15N values.
The hydrogen, carbon, nitrogen and sulphur concentrations of the extracted kerogens were also summarised in Table 1. The observed H, C, and N concentrations in the kerogen samples ranged from 0.0 to 4.4 wt. %, 8.2 to 89 wt. %, and 0.0 to 1.4 wt. %, respectively. The predominant portion of the sulphur in Table 1 appears to be primarily associated with pyrite and to a lesser degree with organic sulphur as pyrite could not be removed from the kerogen by our procedure. The correlation between the H/C and N/C ratios for the examined kerogen samples compared with a previous data set of kerogen samples from the Palaeoproterozoic to Mesoproterozoic (Schopf and Klein, 1992), is shown in Figure S-4. The previous data plots showed a graphitising trend in the N/C and H/C ratios related to the differences in metamorphic grade of each geological section. The N/C and H/C ratios observed in this diagram indicate that the kerogen examined in this study was moderately mature, which was expected based on the metamorphic grade of the Gunflint Formation, which was as low as greenschist facies (Floran and Papike, 1975, Easton et al., 2000).

Figure S-4 Correlation diagram of N/C and H/C ratios of kerogens. Data are sorted by lithology; legends are the same as Figure S-3. Grey squares are data from Mesoproterozoic to Palaeoproterozoic kerogen samples cited from Schopf and Klein (1992).
Carbon and sulphur isotope compositions
The d13C values of the kerogen samples and d34S values of pyrite are shown in Table 1. The d13C values ranged from -33.6 to -25.1 ‰. In general, the d13C values of the ferruginous or chert samples tend to be heavier than those of shale and carbonate samples. Only one outlier value, -25.1 ‰, was observed in sideritic BIF (sample #0723) from the Pass Lake section. No correlation was observed between the d13C and TOC values (Fig. S-3b). The d13C values of Fe-limestones and siderites, ranging from -6.0 to 0.5 ‰ with mean value of -3.3 ‰, were summarised by the previous studies (e.g., Winter and Knauth, 1992, Shield and Veizer, 2002, Ohmoto et al., 2004). These values of dissolved carbon are responsible for the source of organic carbon.
The d34S values of pyrite ranged from -1.1 to +26.9 ‰ with a mean value of +9.9 ‰ (Table 1). The highest value of +26.9 ‰ was obtained from a sample of the carbonate-sand zone from the Current River section (sample #0701). The lowest value of -1.1 ‰ was obtained from an iron-rich layer in a BIF sample from the Pass Lake section (sample #0724).
5. Nitrogen Isotope Compositions and TEM Observations
The bulk d15N values of kerogen, ranging from +2.9 to +8.0 ‰, are summarised in Table 1. The heaviest value of +8.0 ‰ was observed in the kerogen from a carbonate-sand zone (sample #0711), whereas the lowest value of +2.9 ‰ was observed in the black shale (sample #0301). Generally, there are no lithofacies-dependent variations of d15N values.
Figure 1 (in the main text) shows the results of the stepwise combustion analyses performed on 11 kerogen samples in this study (Table S-2). The data from the 2 samples analysed by Ishida et al. (2012) were replotted and were included in Figure 1 for comparison. The nitrogen release amounts, the d15N values for each temperature step, and the average d15N values for the lower (500–575 ºC) and higher (600–1100 ºC) temperature fractions are shown. The average d15N values of the lower and higher-temperature fractions were calculated using the d15N values and the release amounts for the relevant temperature steps. Some samples exhibited higher d15N values for the higher-temperature fraction than for the lower-temperature fraction, while other samples exhibited the opposite trend, similar to the situation observed in Ishida et al. (2012). The release amounts of carbon appear to be fairly well correlated with those of nitrogen, suggesting that the nitrogen was hosted by carbon structures (Ishida et al., 2012).
In Figure 1, we set the boundary between the higher and lower-temperature fractions at 575 ºC, the temperature at which the d15N shift is most likely to occur. However, this boundary may vary within a range of ±25 ºC among the samples, according to the previous observations by Ishida et al. (2012). However, the overall trend of the average d15N values among the samples seems to be insensitive to the exact boundary temperatures adopted for the statistics. To illustrate this pattern, in Figure S-5 and Table S-3, we prepared another version of the average d15N values for the lower and higher temperature ranges. In this version, we determined the boundary of the lower- and the higher-fractions from the stepwise d15N profiles. Figure S-6a and b also demonstrate that the correlation between the d15NLT and the Pr/Sm ratios is the same, while no correlation was observed between the d15NHT and the Pr/Sm ratios. Thus, there is no significant difference between these two types of deviations. Figure S-6c demonstrates that the relationship between the d15N values of bulk kerogen (d15Nbulk) and the Pr/Sm, showing that there is no correlation between them.
Table S-2 Summary of the stepwise combustion analyses performed on kerogen (sample IDs #0701, #0703, #0705, #0706, #0709, #0711, #0712, #0713, #0714, #0721, and #0723).
Temp | C | H | N | C/N | d15N | ± |
°C | ppm | (atomic) | ‰ | |||
0701 (0.047 mg) | ||||||
500 | 82705 | 69 | 81 | 1193 | -5.6 | 0.7 |
525 | 85022 | 121 | 84 | 1180 | 5.1 | 0.8 |
550 | 125467 | 221 | 72 | 2040 | 4.9 | 0.9 |
575 | 164260 | 212 | 389 | 493 | 6.6 | 0.7 |
1100 | 348763 | 436 | 3547 | 115 | 5.8 | 0.8 |
total | 806217 | 1059 | 4172 | |||
average | 225 | 5.6 | 0.8 | |||
0703 (0.226 mg) | ||||||
475 | 79345 | 2544 | 54 | 1724 | 0.5 | 1.8 |
500 | 92155 | 1235 | 82 | 1312 | 3.8 | 0.4 |
525 | 151538 | 1392 | 215 | 822 | 5.9 | 0.6 |
550 | 188591 | 1150 | 466 | 472 | 6.6 | 0.4 |
575 | 218032 | 939 | 823 | 309 | 7.0 | 0.7 |
1100 | 135681 | 576 | 1141 | 139 | 7.9 | 0.5 |
total | 865342 | 7835 | 2780 | |||
average | 363 | 7.1 | 0.5 | |||
0705 (0.286 mg) | ||||||
450 | pre-combustion | |||||
475 | 115150 | 182 | 61 | 2200 | 5.9 | 1.2 |
500 | 141665 | 245 | lost | |||
525 | 46160 | 359 | 200 | 269 | 6.9 | 1.0 |
550 | 10286 | 559 | 388 | 31 | 5.8 | 1.4 |
575 | 5026 | 844 | 1445 | 4 | 8.4 | 1.7 |
1100 | 34316 | 2720 | 2177 | 18 | 7.3 | 1.6 |
total | 352604 | 4908 | - | |||
average | 902 | 5.4 | 1.4 | |||
0706 (0.555 mg) | ||||||
475 | 40784 | 22 | 59 | 803 | 0.1 | 0.8 |
500 | 91937 | 655 | 217 | 495 | 5.0 | 0.4 |
525 | 110533 | 829 | 401 | 322 | 7.6 | 0.4 |
550 | 89845 | 573 | 356 | 295 | 7.9 | 0.4 |
575 | 25852 | 147 | 37 | 804 | 6.4 | 0.5 |
1100 | 22105 | 474 | 42 | 617 | 8.4 | 0.5 |
total | 381056 | 2701 | 1112 | |||
average | 400 | 6.9 | 0.4 | |||
0709 (0.067 mg) | ||||||
500 | 180948 | 197 | 480 | 440 | 2.9 | 0.9 |
525 | 172407 | 137 | 1058 | 190 | 7.4 | 0.6 |
550 | 89034 | 50 | 409 | 254 | 8.7 | 0.4 |
575 | 30518 | 54 | 42 | 850 | 5.6 | 0.5 |
1100 | 53034 | 154 | 59 | 1045 | 4.4 | 0.7 |
total | 525940 | 593 | 2049 | |||
average | 299 | 7.3 | 0.6 | |||
0711 (0.412 mg) | ||||||
450 | pre-combustion | |||||
475 | 60541 | 93 | 126 | 803 | 7.2 | 0.5 |
500 | 99552 | 477 | 317 | 495 | 7.8 | 0.3 |
525 | 122193 | 545 | 756 | 322 | 6.7 | 0.4 |
550 | 125328 | 578 | 1018 | 295 | 8.8 | 0.3 |
575 | 13805 | 265 | 75 | 804 | 8.1 | 0.4 |
1100 | lost | |||||
total | 421419 | 1958 | 2291 | |||
average | 215 | 8.0 | 0.4 | |||
0712 (0.072 mg) | ||||||
500 | 192440 | 1022 | 544 | 412 | 4.6 | 0.8 |
525 | 263813 | 374 | 2211 | 139 | 6.2 | 0.7 |
550 | 139411 | 250 | 1212 | 134 | 8.7 | 0.5 |
575 | 15570 | 70 | 54 | 339 | 7.7 | 0.7 |
1100 | 40299 | 229 | 21 | 2254 | 4.7 | 1.2 |
total | 651534 | 1944 | 4041 | |||
average | 188 | 7.2 | 0.3 | |||
0713 (0.065 mg) | ||||||
500 | 157778 | 365 | 280 | 656 | 5.0 | 0.7 |
525 | 116425 | 210 | 196 | 691 | 7.6 | 0.5 |
550 | 24157 | 42 | 25 | 1108 | 6.6 | 0.6 |
575 | 6824 | 17 | 8 | 944 | 5.9 | 1.3 |
1100 | 29652 | 98 | 24 | 1457 | 2.7 | 1.3 |
total | 334837 | 731 | 535 | |||
average | 731 | 6.4 | 0.6 | |||
0714 (0.611 mg) | ||||||
450 | pre-combustion | |||||
475 | 96101 | 762 | 115 | 973 | 2.9 | 0.7 |
500 | 85257 | 837 | 122 | 814 | 4.4 | 0.8 |
525 | 103927 | 626 | 145 | 834 | 4.9 | 0.8 |
550 | 135435 | 770 | 231 | 683 | 4.5 | 1.0 |
575 | 117687 | 504 | 258 | 533 | 6.1 | 1.1 |
1100 | 24930 | 144 | 202 | 144 | 5.8 | 0.8 |
total | 563338 | 3644 | 1074 | |||
average | 612 | 4.8 | 0.9 | |||
0721 (0.153 mg) | ||||||
500 | 54442 | 17 | 33 | 1923 | 2.1 | 0.6 |
525 | 57008 | 15 | 62 | 1079 | 5.5 | 0.6 |
550 | 29440 | 6 | 19 | 1785 | 5.6 | 0.7 |
575 | 5187 | 6 | 3 | 1856 | lost | |
1100 | 32579 | 24 | 15 | 2568 | 6.8 | 0.8 |
total | 178657 | 67 | 132 | |||
average | 1579 | 4.7 | 0.6 | |||
0723 (0.412 mg) | ||||||
450 | pre-combustion | |||||
475 | 28654 | 68 | 94 | 357 | 4.6 | 0.3 |
500 | 29798 | 76 | 138 | 252 | 5.2 | 0.5 |
525 | 34056 | 68 | 246 | 161 | 6.6 | 0.5 |
550 | 38036 | 77 | 360 | 123 | 5.1 | 0.3 |
575 | 38036 | 43 | 402 | 110 | 5.4 | 0.3 |
1100 | 45206 | 166 | 30 | 1739 | 6.6 | 0.4 |
total | 213785 | 498 | 1271 | |||
average | 196 | 5.4 | 0.4 |
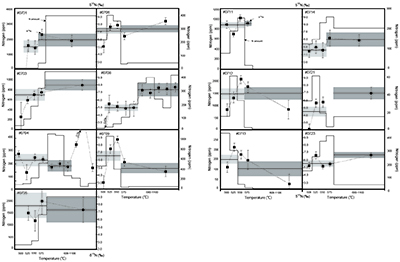
Figure S-5 An alternative version of Figure 1. The lower- and the higher-temperature fractions are divided depending on the shift of d15N values at approximately 575 °C. The nitrogen concentrations (white bars) and d15N values (black squares) are shown.
Table S-3 Summary of the d15NLT and d15NHT values of the 13 samples analysed with the stepwise combustion method. ‘d15N (‰): divided by temperature’ refers to the lower- and higher-temperature fractions divided based on the temperature: lower temperature = 500–575 °C, higher temperature = higher than 575 to 1100 °C. ‘d15N (‰): divided by d15N values’ means the data are divided and calculated into two fractions according to the shift in d15N values (refer to Fig. S-5). The average d15N values were calculated based on the weighted average of the released amount of nitrogen.
Sample ID | Litho facies | d15N (‰): divided by temperature | d15N (‰): divided by d15N values | ||||||||
d15NLT | ± | d15NHT | ± | temperature range | d15NLT | ± | temperature range | d15NHT | ± | ||
0701 | Carbonate sandstone | 4.6 | 0.7 | 5.8 | 0.8 | 500-550 °C | 5.0 | 0.8 | 575-1100 °C | 5.9 | 0.8 |
0703 | Carbonate sandstone | 6.5 | 0.6 | 7.9 | 0.5 | 500-575 °C | 6.5 | 0.6 | 1100 °C | 7.9 | 0.5 |
0704 | Carbonate sandstone | 5.9 | 0.8 | 5.5 | 0.8 | 500-575 °C | 5.9 | 0.8 | 600-650 °C | 5.2 | 0.8 |
0705 | Carbonate sandstone | 7.8 | 1.6 | 7.3 | 1.6 | 500-575 °C | 7.8 | 1.6 | 1100 °C | 7.3 | 1.6 |
0706 | Carbonate sandstone | 7.1 | 0.4 | 8.4 | 0.5 | 500-550 °C | 7.1 | 0.4 | 575-1100 °C | 7.4 | 0.5 |
0708 | Carbonate sandstone | 4.3 | 1.0 | 7.1 | 0.8 | 500-600 °C | 5.0 | 1.0 | 625-1100 °C | 7.3 | 0.8 |
0709 | Carbonate sandstone | 6.5 | 0.6 | 4.4 | 0.7 | 500-575 °C | 6.5 | 0.6 | 1100 °C | 4.9 | 0.7 |
0711 | Carbonate sandstone | 7.9 | 0.4 | - | - | 500-550 °C | 7.9 | 0.4 | - | - | - |
0712 | Carbonate sandstone | 6.8 | 0.7 | 4.7 | 1.2 | 500-550 °C | 6.8 | 0.7 | 575-1100 °C | 6.9 | 0.8 |
0713 | Shale (phosphorous) | 6.1 | 0.6 | 2.7 | 1.3 | 500-525 °C | 6.1 | 0.6 | 550-1100 °C | 4.9 | 1.0 |
0714 | Black shale | 5.1 | 1.0 | 5.8 | 0.8 | 500-550 °C | 4.6 | 0.9 | 575-1100 °C | 6.0 | 0.9 |
0721 | Taconite | 4.4 | 0.6 | 6.8 | 0.8 | 500-550 °C | 4.4 | 0.6 | 1100 °C | 6.8 | 0.8 |
0723 | Carbonate BIF | 5.5 | 0.4 | 6.6 | 0.4 | 500-575 °C | 5.5 | 0.4 | 1100 °C | 6.6 | 0.4 |

Figure S-6 The correlations between the (a) d15NLT values, and (b) the d15NHT values, and the Pr/Sm ratios. Both d15NLT and d15NHT are divided based on the temperatures of the shift of the d15N values that were observed on each sample. The results of calculations are summarised in the Table S-3. The same trends as in Figure 2 were observed: a positive correlation is observed between the Pr/Sm ratios and d15NLT values (R2 = 0.59, excluding #0714), whereas no correlation is observed between the Pr/Sm ratios and d15NHT values. (c) There is no correlation between the d15Nbulk values and the Pr/Sm ratios.
The TEM observation of kerogen supports the heterogeneous carbon structure (Fig. S-7). Two types of carbon structures were observed in kerogen: disordered and folding structure, and well-ordered graphane-stacking structures. The differences in host organic matter would be responsible for the heterogeneity of nitrogen isotope compositions.

Figure S-7 Transmitted electron microscopic image of the kerogen extracted from sample #0708. There are at least two types of carbon structures: a highly graphitised portion (white arrow) and folded structures (black arrow). This difference in structure might be responsible for the differences in the nitrogen isotopes. The scale bar is 200 nanometres. Insert: Selected area electron diffraction pattern of the highly graphitised area.
6. Tectonic and Oceanic Environment in the Animikie Basin
Continental and hydrothermal fluxes
The samples examined in the present study include both siliciclastic and chemical sedimentary rocks. Both types contain variable amounts of detrital minerals. The contribution of detrital minerals to the bulk rock chemistry can be evaluated using major and trace elements. For example, K and Ti are correlated with Al, suggesting K and Ti were also introduced into the sediments by detrital clay and oxide minerals. V, Th, Sc, and Ni show positive correlations with Zr in all examined samples, suggesting that V, Th, and Sc are also associated with detrital minerals, most likely oxides (Fig. S-8). The carbonate samples contain appreciable amounts of Al, K and Ti, suggesting that large amounts of weathering products were added to the shallow part of the Animikie basin. The effects of detrital materials are also observed in the REE patterns of these samples. For example, a portion of the carbonate samples (e.g., samples #0702 and #0705) from the Current River section exhibit enrichment in light REEs (LREEs) relative to heavy REEs (HREEs), comparable to the patterns of the shales (e.g., samples #0301 and #0304). These trends suggest a significant input of continental detritus in these carbonate samples (Fig. S-9, Tables S-1, S-4).
The concentrations of Fe were generally high in carbonate samples (Fig. S-8b), indicating that anoxic submarine hydrothermal plumes supplied dissolved Fe2+ to the shallow ocean (e.g., Simonson, 1985; Derry and Jacobsen, 1990; Isley, 1995; Carrigan and Cameron, 1991; Fralick et al., 2002; Poulton et al., 2010). In addition, most samples exhibit positive Eu anomalies, supporting the above model for carbonate precipitation under the influence of submarine hydrothermal plumes (e.g., Derry and Jacobsen, 1990; Poulton et al., 2010).
The carbonates observed at the Terry Fox section represent the uppermost section of the Gunflint Formation. They are depleted in light REEs (LREEs) compared to heavy REEs (HREEs) (Fig. S-9d). These patterns apparently resemble the REE pattern of the contemporary ocean (Johannesson et al., 2006 and references therein). The Al and Zr concentrations are much lower in this section. Furthermore, the positive Eu anomaly is less clear in these samples, which also have lower Fe concentrations. These observations suggest that submarine hydrothermal and the continental fluxes were comparatively reduced during the deposition of the carbonate in the Terry Fox section.
The REE patterns of shale samples are shown in Figure S-9. Samples #0301 and #0304 are enriched in LREEs and feature flat MREE to HREE patterns. This type is the most dominant among the examined shale samples. Their protoliths were most likely enriched in LREEs relative to PAAS, thereby indicating a greater contribution from felsic to intermediate source rocks. Shale samples from the Terry Fox section (samples #0725 and #0726) and one sample from the Blend Lake North section (sample #0302) show LREE-depleted and flat MREE and HREE patterns. Such a pattern is explained by greater contributions from mafic components, including basaltic tuffs.
An evolution model for the Animikie Basin has been proposed by previous investigators (e.g., Schulz and Cannon, 2007). This model was based on the geology and chronology of the Marquette Range Supergroup in the Wisconsin and Michigan area. The proposed model can be applied to the Gunflint Formation because of its chronological and lithological correlation to the Marquette sections. At ca. 1875 Ma, an arc started to collide with an embayment along the southern edge of the Superior craton, forming a broad and shallow basin in which northerly-derived siliciclastic sediments were deposited on Archean crust. After collision, the northward subduction led to a period of back-arc extension, generating submarine volcanism and the release of hydrothermal plumes in the Animikie Basin. As a result, laterally extensive BIFs and other Fe-rich rocks formed in the Animikie basin. During this period of arc collision and back-arc extension, the Superior craton, which has an intermediate crustal composition, was the main source of detrital material. By 1850 Ma, subduction had ceased, and the Sudbury impact event had occurred. As a result, a foreland basin replaced the back-arc basin, and more detrital material was supplied from “old” portions of the arc, which had more mafic tholeiitic volcanics. These tholeiitic volcanics have different REE patterns than the Superior craton. Thus, the change in sediment source is recorded in the LREE-depleted characteristics of shales in the upper part of the Gunflint Formation. This tectonic history is consistent with the observed LREE-enriched patterns in a majority of the shales (Fig. S-9).
Table S-4 Summary of REE concentrations of the examined samples normalised by the PAAS values.
sample ID | La | Ce | Pr | Nd | Sm | Eu | Gd | Tb | Dy | Y | Ho | Er | Tm | Yb | Lu |
(PAAS normalised) | |||||||||||||||
0716 | 0.022 | 0.036 | 0.018 | 0.016 | 0.015 | 0.041 | 0.017* | 0.112* | 0.019* | 0.073* | 0.095* | 0.033* | 0.111* | 0.033* | 0.091* |
0718 | 0.191 | 0.207 | 0.212 | 0.249 | 0.349 | 0.856 | 0.447 | 0.449 | 0.469 | 0.659 | 0.475 | 0.390 | 0.310 | 0.232 | 0.182 |
0719 | 0.128 | 0.130 | 0.125 | 0.137 | 0.189 | 0.453 | 0.248 | 0.224 | 0.225 | 0.256 | 0.190 | 0.195 | 0.177 | 0.133 | 0.114 |
0720 | 0.114 | 0.113 | 0.117 | 0.126 | 0.174 | 0.420 | 0.215 | 0.224 | 0.282 | 0.330 | 0.285 | 0.293 | 0.266 | 0.199 | 0.182 |
0721 | 0.070 | 0.069 | 0.084 | 0.072 | 0.102 | 0.255 | 0.149 | 0.224 | 0.188 | 0.256 | 0.190 | 0.228 | 0.222 | 0.166 | 0.160 |
0722 | 0.043 | 0.046 | 0.038 | 0.040 | 0.044 | 0.115 | 0.066 | 0.112* | 0.056 | 0.073* | 0.095* | 0.065 | 0.111* | 0.033* | 0.091* |
0723 | 0.110 | 0.135 | 0.107 | 0.110 | 0.131 | 0.230 | 0.182 | 0.224 | 0.169 | 0.256 | 0.190 | 0.163 | 0.155 | 0.100 | 0.091* |
0301 | 1.066 | 0.914 | 0.639 | 0.461 | 0.363 | 0.494 | 0.331 | 0.449 | 0.469 | 0.586 | 0.475 | 0.553 | 0.599 | 0.631 | 0.616 |
0302 | 0.379 | 0.577 | 0.637 | 0.841 | 1.293 | 1.951 | 1.390 | 1.346 | 1.221 | 1.208 | 1.235 | 1.171 | 1.220 | 1.162 | 1.140 |
0303 | 0.272 | 0.301 | 0.275 | 0.300 | 0.363 | 0.551 | 0.381 | 0.337 | 0.338 | 0.403 | 0.285 | 0.325 | 0.310 | 0.299 | 0.296 |
0304 | 0.597 | 0.484 | 0.332 | 0.263 | 0.232 | 0.321 | 0.232 | 0.337 | 0.376 | 0.513 | 0.380 | 0.455 | 0.510 | 0.498 | 0.502 |
0305 | 0.489 | 0.708 | 0.831 | 0.981 | 1.206 | 1.942 | 1.340 | 1.234 | 1.070 | 1.318 | 0.950 | 0.813 | 0.643 | 0.498 | 0.456 |
0306 | 0.177 | 0.195 | 0.160 | 0.163 | 0.203 | 0.329 | 0.248 | 0.337 | 0.300 | 0.403 | 0.285 | 0.293 | 0.288 | 0.232 | 0.228 |
0307 | 0.074 | 0.087 | 0.068 | 0.070 | 0.087 | 0.148 | 0.099 | 0.112* | 0.094 | 0.183 | 0.095* | 0.098 | 0.111* | 0.033* | 0.091* |
0308 | 0.056 | 0.065 | 0.048 | 0.046 | 0.058 | 0.091 | 0.066 | 0.112* | 0.075 | 0.110 | 0.095* | 0.098 | 0.111* | 0.100 | 0.091* |
0309 | 0.236 | 0.275 | 0.229 | 0.230 | 0.276 | 0.379 | 0.314 | 0.337 | 0.376 | 0.549 | 0.380 | 0.390 | 0.355 | 0.332 | 0.319 |
0310 | 0.052 | 0.063 | 0.048 | 0.048 | 0.058 | 0.099 | 0.066 | 0.112* | 0.056 | 0.110 | 0.095* | 0.065 | 0.111* | 0.033* | 0.091* |
0311 | 0.022 | 0.028 | 0.020 | 0.019 | 0.029 | 0.041 | 0.017* | 0.112* | 0.019* | 0.073* | 0.095* | 0.033* | 0.111* | 0.033* | 0.091* |
0701 | 0.065 | 0.091 | 0.075 | 0.078 | 0.087 | 0.123 | 0.083 | 0.112* | 0.075 | 0.110 | 0.095* | 0.098 | 0.111* | 0.066 | 0.091* |
0702 | 0.339 | 0.315 | 0.163 | 0.110 | 0.087 | 0.107 | 0.050 | 0.112* | 0.075 | 0.110 | 0.095* | 0.098 | 0.111* | 0.100 | 0.114 |
0703 | 0.076 | 0.096 | 0.072 | 0.064 | 0.073 | 0.107 | 0.066 | 0.112* | 0.075 | 0.110 | 0.095* | 0.098 | 0.111* | 0.100 | 0.091* |
0704 | 0.061 | 0.074 | 0.057 | 0.051 | 0.058 | 0.091 | 0.050 | 0.112* | 0.056 | 0.073* | 0.095* | 0.065 | 0.111* | 0.033* | 0.091* |
0705 | 0.182 | 0.202 | 0.143 | 0.123 | 0.116 | 0.165 | 0.116 | 0.112* | 0.075 | 0.110 | 0.095* | 0.065 | 0.111* | 0.066 | 0.091* |
0706 | 0.175 | 0.187 | 0.150 | 0.137 | 0.145 | 0.206 | 0.132 | 0.112* | 0.113 | 0.146 | 0.095* | 0.098 | 0.111* | 0.100 | 0.091* |
0707 | 0.076 | 0.088 | 0.053 | 0.054 | 0.058 | 0.091 | 0.066 | 0.112* | 0.094 | 0.146 | 0.095* | 0.098 | 0.111* | 0.100 | 0.114 |
0708 | 0.092 | 0.120 | 0.110 | 0.118 | 0.145 | 0.239 | 0.182 | 0.112* | 0.131 | 0.183 | 0.095* | 0.098 | 0.111* | 0.066 | 0.091* |
0709 | 0.132 | 0.161 | 0.135 | 0.123 | 0.131 | 0.165 | 0.116 | 0.112* | 0.075 | 0.073* | 0.095* | 0.098 | 0.111* | 0.066 | 0.091* |
0710 | 0.153 | 0.159 | 0.120 | 0.102 | 0.102 | 0.156 | 0.083 | 0.112* | 0.094 | 0.110 | 0.095* | 0.228 | 0.111* | 0.100 | 0.114 |
0711 | 0.227 | 0.240 | 0.189 | 0.177 | 0.203 | 0.346 | 0.215 | 0.224 | 0.244 | 0.439 | 0.285 | 0.228 | 0.200 | 0.166 | 0.160 |
0712 | 0.126 | 0.131 | 0.101 | 0.086 | 0.087 | 0.156 | 0.083 | 0.112* | 0.094 | 0.146 | 0.095* | 0.098 | 0.111* | 0.100 | 0.091* |
0713 | 0.390 | 0.432 | 0.311 | 0.281 | 0.305 | 0.535 | 0.331 | 0.337 | 0.300 | 0.476 | 0.285 | 0.260 | 0.244 | 0.199 | 0.182 |
0714 | 0.408 | 0.344 | 0.247 | 0.196 | 0.174 | 0.189 | 0.132 | 0.112* | 0.075 | 0.073* | 0.095* | 0.098 | 0.111* | 0.066 | 0.091* |
0715 | 0.285 | 0.256 | 0.210 | 0.180 | 0.174 | 0.247 | 0.149 | 0.224 | 0.169 | 0.256 | 0.190 | 0.228 | 0.266 | 0.266 | 0.296 |
0724 | 0.182 | 0.201 | 0.149 | 0.139 | 0.160 | 0.280 | 0.232 | 0.224 | 0.263 | 0.439 | 0.285 | 0.260 | 0.222 | 0.199 | 0.160 |
0725 | 0.018 | 0.027 | 0.028 | 0.035 | 0.044 | 0.082 | 0.066 | 0.112* | 0.113 | 0.073* | 0.095* | 0.130 | 0.133 | 0.100 | 0.114 |
0726 | 0.085 | 0.091 | 0.083 | 0.088 | 0.116 | 0.214 | 0.149 | 0.224 | 0.188 | 0.256 | 0.190 | 0.195 | 0.177 | 0.133 | 0.137 |
0729 | 0.009 | 0.007* | 0.007* | 0.013 | 0.029 | 0.041 | 0.033 | 0.112* | 0.038 | 0.073* | 0.095* | 0.033* | 0.111* | 0.066 | 0.091* |
0730 | 0.007* | 0.007* | 0.009 | 0.019 | 0.044 | 0.066 | 0.050 | 0.112* | 0.038 | 0.073* | 0.095* | 0.033* | 0.111* | 0.066 | 0.091* |
0601 | 0.175 | 0.198 | 0.173 | 0.169 | 0.189 | 0.337 | 0.248 | 0.224 | 0.263 | 0.366 | 0.285 | 0.260 | 0.222 | 0.199 | 0.205 |
0602 | 0.007* | 0.007* | 0.006 | 0.005* | 0.015 | 0.041 | 0.017* | 0.112* | 0.019* | 0.073* | 0.095* | 0.033* | 0.111* | 0.033* | 0.091* |
0603 | 0.254 | 0.342 | 0.267 | 0.276 | 0.320 | 0.370 | 0.414 | 0.112* | 0.131 | 0.549 | 0.095* | 0.130 | 0.133 | 0.133 | 0.274 |
0604 | 0.090 | 0.131 | 0.101 | 0.086 | 0.087 | 0.132 | 0.099 | 0.112* | 0.131 | 0.146 | 0.095* | 0.130 | 0.133 | 0.133 | 0.114 |
0605 | 0.029 | 0.044 | 0.022 | 0.024 | 0.029 | 0.041 | 0.017* | 0.112* | 0.019* | 0.073* | 0.095* | 0.065 | 0.111* | 0.066 | 0.091* |
PAAS values | La | Ce | Pr | Nd | Sm | Eu | Gd | Tb | Dy | Y | Ho | Er | Tm | Yb | Lu |
44.56 | 88.25 | 10.15 | 37.32 | 6.884 | 1.215 | 6.043 | 0.8914 | 5.325 | 27.31 | 1.053 | 3.075 | 0.451 | 3.012 | 0.4386 |
* below detection limit
PAAS values were referred from Pourmand et al. (2012)
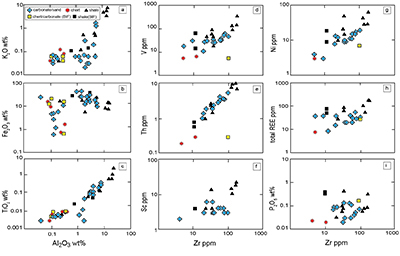
Figure S-8 Correlation diagrams of Al2O3 versus K2O, Fe2O3, and TiO2 in bulk rock and Zr versus V, Th, Sc, Ni, total amount of rare earth elements (total REEs), and P2O5 concentrations. K2O (a) and TiO2 (c) show positive correlations with Al2O3, whereas Fe2O3 (b) does not exhibit a correlation with Al2O3. V (d), Th (e), Sc (f), and Ni (g) have positive correlations with Zr. Total REEs and P2O5 have weak positive correlations. Data below the detection limit were omitted. Data are sorted by lithology; legends are the same as Figure S-3.
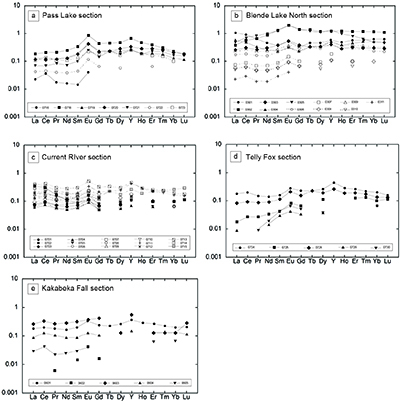
Figure S-9 Diagrams of the rare earth elements and Y in each surveyed section: (a) Pass Lake section, (b) Blende Lake North section, (c) Current River section, (d) Terry Fox section, and (e) Kakabeka Falls section.
REE behaviour and implications for the redox conditions of the ocean
Redox stratification of Palaeoproterozoic oceans has been proposed by previous investigators (e.g., Canfield et al., 1996; Anbar and Knoll, 2002; Poulton et al., 2004, 2010; Hiatt et al., 2015). However, it is unclear whether the shallow part of the Gunflint ocean was widely oxic or anoxic (e.g., Poulton et al., 2004, 2010; Rasmussen et al., 2012; Hiatt et al., 2015).
The mineralogy and chemistry of our samples suggest widespread oxic shallow ocean conditions during the deposition of the Gunflint Formation. Grains of microscopic haematite are common in carbonate oolite (Pass Lake section, Fig. S-2a), and these grains are protected by a ferrodolomite matrix. Therefore, these haematite grains are not modern weathering products. The Ce/Ce* anomaly observed in the REE data further supports the argument of an oxic environment in the Gunflint sedimentary basin. The negative correlation observed between Ce/Ce* and Pr/Pr* in the examined samples (Fig. S-10) indicates that the observed Ce anomaly is a real trend, as opposed to false trends that may occur because of La anomalies (Bolhar et al., 2005). These observations are consistent with the redox-stratified model suggested by previous investigators (Planavsky et al., 2009).

Figure S-10 Correlation diagrams of (a) Pr/Pr* and Ce/Ce* ratios and (b) Ce/Ce* and Eu/Eu* ratios. Pr/Pr* is defined as Pr/0.5(Ce+Nd), and Ce/Ce* is defined as Ce/0.5(La+Pr). A negative correlation exists between them. Data are sorted by lithology; legends are the same as Figure S-3.
The occurrence of phosphorite supports the existence of a redox boundary in the water column (sample #0713). An Fe-pump model has been proposed by previous investigators to explain the initial concentration of phosphates in the Animikie sedimentary rocks (e.g., Bjerrum and Canfield, 1996; Edwards, et al., 2012; Hiatt et al., 2015). In this model, dissolved phosphorous was adsorbed on host Fe-hydroxides in the vicinity of the redox boundary and then deposited in the sediment. The presence of Fe-hydroxides is the key for the Fe-pump model, and oxic surface water is required to form Fe-hydroxides (Nelson et al., 2010). Later diagenetic processes re-mobilised and concentrated the phosphate, forming pristine phosphorite in the Gunflint sediments (Hiatt et al., 2015). Flooding processes brecciated and transported the pristine phosphorites to the deeper sedimentary basin, allowing re-deposition of granular and rounded phosphorites, as seen in Figure S-2e. Arsenic is only detected in the framboidal pyrite around the phosphorites, and other euhedral pyrites, which occur in the carbonate shale hosting phosphorites, do not contain As. Arsenic compounds can be precipitated in sediments associated with Fe-hydroxides and phosphorus because the chemical behaviour of As resembles that of Fe (O’Day, 2006). These local concentrations of As likely originated from submarine hydrothermal activity and were concentrated in the sediments by the Fe-pump process. Although the formation process of framboidal pyrite in the examined samples remains unclear, Fe-hydroxides can act as a precursor (Morse and Wang, 1997, Ohfuji and Rickard, 2005). In modern environments, phosphorus is released from terrestrial rocks by weathering and transported into the oceans by rivers. This phosphorous is then fixed into marine sediments through biological activities (Paytan and McLaughlin, 2007). In Palaeoproterozoic redox-stratified oceans, the adsorption of phosphorous to Fe-hydroxides might have played a more important role in the accumulation of phosphorous in marine sediments (e.g., Papineau et al., 2009, 2013; Edwards et al., 2012). In the present study, we could not observe such an occurrence of phosphorite except for sample #0713. We interpreted that the most of P-related minerals, which originally existed in the Gunflint Formation, were already silicified and lost.
The correlation coefficient values between the Pr/Sm ratio and the major and trace element concentrations, and d15N values among examined samples, are summarised in Table S-5. This table shows that some bioessential trace metals, such as Mo, V, and Ni, have positive correlations with the Pr/Sm ratios, suggesting that the increment of continental input supplied them to the ocean.
Table S-5 Correlation coefficient values of the Pr/Sm ratios against the major and trace elements concentrations. Elements which have positive correlations with the Pr/Sm ratios are displayed in bold.
Major elements | Trace elements | REEs | d15N values | ||||
SiO2 | 0.23 | Sc | 0.67 | La | 0.62 | d15NLT | 0.56 |
Al2O3 | 0.68 | As | -0.23 | Ce | 0.49 | d15NHT | 0.03 |
Fe2O3 | -0.10 | Hf | 0.74 | Pr | 0.25 | d15Nbulk | <0.01 |
MnO | -0.43 | Ta | 0.67 | Nd | 0.04 | ||
MgO | -0.36 | Th | 0.69 | Sm | -0.12 | ||
CaO | -0.36 | U | 0.29 | Eu | -0.23 | ||
Na2O | -0.13 | Zr | 0.76 | Gd | -0.23 | ||
K2O | 0.56 | Cr | 0.64 | Tb | -0.15 | ||
TiO2 | 0.68 | Co | 0.37 | Dy | -0.15 | ||
P2O5 | -0.23 | Cd | 0.01 | Y | -0.16 | ||
Cu | 0.56 | Ho | -0.11 | ||||
Ni | 0.42 | Er | -0.01 | ||||
Zn | 0.48 | Tm | 0.11 | ||||
Ag | -0.36 | Yb | 0.23 | ||||
Pb | -0.27 | Lu | 0.27 | ||||
V | 0.53 | ||||||
Ba | 0.55 | ||||||
Sr | -0.10 | ||||||
Ga | 0.69 | ||||||
Ge | 0.71 | ||||||
Rb | 0.59 | ||||||
Mo | 0.23 |
The components which have positive correlation coefficiencies are displayed in bold.
Download in ExcelAvailability of sulphate
Previous investigators suggested that the sulphate concentration in the Animikie Basin was very low and that the Gunflint Formation records a global transition into a sulfidic ocean in the Palaeoproterozoic (e.g., Poulton et al., 2004, 2010; Johnston et al., 2006). However, some investigators questioned the suitability of using the Gunflint Formation to discuss a global transition into a sulphidic ocean and the availability of sulphate in the Animikie Basin (Pufahl et al., 2010). These arguments are based on C (TOC) and S (Spyr) concentrations (i.e. Spyr/TOC ratios) of sedimentary rocks, d34S values of pyrite, or Fe-speciation analyses in the Gunflint, Rove, and Michigamme Formations. In particular, the Spyr/TOC ratios in Gunflint Formation are the key issue in this discussion.
The TOC values in the present study are compatible with those of Poulton et al. (2004). In contrast, the Spyr concentrations are much higher than those of Poulton et al. (2004). The Spyr concentrations by Poulton et al. (2004) are less than 0.19 wt. % S, whereas those of the present study are up to 0.58 wt. % S. In particular, samples #0721 and #0725 feature high Spyr values, but their TOC values are similar to those of the other samples. Sample #0725 is composed of Fe-rich dolomite. The pyrite crystals found in this sample are closely associated with laminated organic matter (Fig. S-2i). The absence of hydrothermal alteration and the generally high d34S values suggest that these pyrite crystals are products of microbial sulphate reduction. Other carbonate samples with moderate TOC values also have microscopic pyrite in organic-rich laminae that yield moderate Spyr concentrations (up to 0.1 wt. % S). The high Spyr and low TOC concentrations in sample #0725 are explained by the difference in the digestibility of organic matter by sulphate-reducing bacteria. Microbial sulphate reduction generally proceeds through the following reaction:
Reaction S-1
SO42- + 2CH2O-R = 2HCO3- + H2S + R
where R is the residual, non-digestible organic matter included in the TOC. If a large portion of the sedimentary organic matter is digestible by sulphate reducers, large amounts of Spyr will be generated, even if the concentrations of TOC, represented in Reaction S-1 as CH2O-R, are not similarly high (Berner and Raiswell, 1984, Raiswell and Berner, 1986).
The Spyr/TOC ratio of the oolitic sample (sample #0721) was also high. If the pyrite crystals were formed by reaction (1), the original amount of organic matter must have been more than 10 times higher than the observed amount. The N/C ratio of the extracted kerogen from the oolite (N/C = 0.009) is much lower than the values of other kerogen samples examined in this study and in the literature (e.g., Alleon et al., 2016). These facts imply that the Gunflint oolitic samples were originally rich in organic matter, and the organic matter was degraded by sulphate-reducing bacteria during diagenesis. The study by Wacey et al. (2013) supports the above model arguing for the active digestion of organic matter by sulphate-reducing bacteria in the Gunflint sediments.
The scattered relationship between Spyr and TOC concentrations among all the examined samples shows a weak correlation (Fig. S-3a). The overall Spyr/TOC ratio for the Gunflint samples is 0.26 ± 0.08. This ratio is much higher than that of 0.04 obtained by Poulton et al. (2004). The samples examined by Poulton et al. (2004) were mainly carbonate or sandstone, whereas our samples contain black shales and/or thin shale laminae in BIF, and carbonates. Pufahl et al. (2010) also found that deep-water shale in the Michigamme Formation contains abundant pyrite and that deltaic sediments, such as sandstone, do not contain appreciable amounts of pyrite because of the introduction of more fresh river water in the shallow coastal environments. On the other hand, the oolitic samples and carbonates in the present study have appreciable amounts of pyrite, despite their deposition in shallow or low-stand environments. The Animikie Basin experienced several sea level transgression/regression cycles; thus, the occasional mixing between sulphate-rich seawater with sulphate-poor fresh water is possible. This explains the diversity of Spyr concentrations in the literature.
Supplementary Information References
Alleon, J., Bernard, S., Le Guillou, C., Marin-Carbonne, J., Pont, S., Beyssac, O., McKeegan, K., Robert, F. (2016) Molecular preservation of 1.88 Ga Gunflint organic microfossils as a function of temperature and mineralogy. Nature Communications 7, Article number 11977.
Anbar, A.D., Knoll, A.H. (2002) Proterozoic Ocean Chemistry and Evolution: A Bioinorganic Bridge? Science 297, 1137–1142.
Beaumont, V., Robert, F. (1999) Nitrogen isotope ratios of kerogens in Precambrian cherts: a record of the evolution of atmosphere chemistry? Precambrian Research 96, 63–82.
Berner, R.A., Raiswell, R. (1984) C/S method for distinguishing freshwater from marine sedimentary rocks. Geology 12, 365–368.
Bjerrum, C.J., Canfield, D.E. (1999) Ocean productivity before about 1.9 Gyr ago limited by phosphorus adsorption onto iron oxides. Nature 417, 159–162.
Blackwelder, E. (1914) A summary of the orogenic epochs in the geologic history of Northern America. Journal of Geology 22, 633–654.
Bolhar, R., Van Krenendonk, M., Kamber, B.S. (2005) A trace element study of siderite-jasper banded iron formation in the 3.45 Ga Warrawoona Group, Pilbara Craton-Formation from hydrothermal fluids and shallow seawater. Precambrian Research 137, 93–114.
Canfield, D.E., Teske, A. (1996) Late Proterozoic rise in atmospheric oxygen concentration inferred from phylogenetic and sulphur-isotope studies. Nature 382, 127–132.
Carrigan, W.J., Cameron, E.M. (1991) Petrological and stable isotope studies of carbonate and sulfide minerals from Gunflint Formation, Ontario: evidence for the origin of early Proterozoic iron-formation. Precambrian Research 52, 347–380.
Derry, L.A., Jacobsen, B.J. (1990) The chemical evolution of Precambrian seawater: Evidence from REEs in banded iron formations. Geochimica et Cosmochimica Acta 54, 2965–2977.
De Matties, T.A. (1994) Early Proterozoic volcanogenic massive sulfide deposits in Wisconsin; an overview. Economic Geology 89, 1122–1151.
Easton, R.M. (2000) Metamorphism of the Canadian Shield, Ontario Canada. II Proterozoic metamorphic history. Canadian Mineralogist 38, 319–344.
Edwards, C.T., Pufahl, P.K., Hiatt, E.E., Kyser, T.K. (2012) Paleoenvironmental and taphonomic controls on the occurrence of Paleoproterozoic microbial communities in the 1.88 Ga Ferriman Group, Labrador Trough, Canada. Precambrian Research 212–213, 91–106.
Floran, R.J., Papike, J.J. (1975) Petrology of the Low-Grade Rocks of the Gunflint Iron-Formation, Ontario-Minnesota. Geological Society of America Bulletin 86, 1169–1190.
Fralick, P., Davis, D.W., Kissin, S.A. (2002) The age of Gunflint Formation, Ontario, Canada: single zircon U-Pb age determinations from reworked volcanic ash. Canadian Journal of Earth Science 39, 1085–1091.
Fralick, P., Planavsky, N., Burton, J., Jarvis, I., Addison, W.D., Barrett, T.J., Brumpton, G.R. (2017) Geochemistry of Paleoproterozoic Gunflint Formation carbonate: implications for hydrosphere-atmosphere evolution. Precambrian Research 290, 126–146.
Godfrey, L.V., Poulton, S.W., Bebout, G.E., Fralick, P.W. (2013) Stability of the nitrogen cycle during development of sulfidic water in the redox-stratified late Paleoproterozoic Ocean. Geology 41, 655–658.
Goodwin, A.M. (1956) Facies relations in the Gunflint iron formation. Economic Geology 51, 565-595.
Hemming, S.R., McLennan, S.M., Hanson, G.N. (1995) Geochemical and Nd/Pb isotopic evidence for the provenance of the early Proterozoic Virginia formation, Minnesota. Implications for the tectonic setting of the Animikie basin. The Journal of Geology 103, 147–168.
Hiatt, E.E., Pufahl, P.K., Edwards, C.T. (2015) Sedimentary phosphate and associated fossil bacteria in a Paleoproterozoic tidal flat in the 1.85 Ga Michigamme Formation, Michigan, USA. Sedimentary Geology 319, 24–39.
Hoffman, P.F. (1988) United plates of America, the birth of a craton—Early Proterozoic assembly and growth of Laurentia. Annual Review of Earth and Planetary Sciences 16, 543–603.
House, H.C., Schopf, J.W., McKeegan, K.D., Coath, C.D., Harrison, T.M., Stetter, K.O. (2000) Carbon isotopic composition of individual Precambrian microossils. Geology 28, 707–710.
Ishida, A., Hashizume, K., Kakegawa, T. (2012) Stepwise combustion analyses of distinct nitrogen isotopic compositions on Paleoproterozoic organic matter, Geochemical Journal 46, 249–253.
Isley, A.E. (1995) Hydrothermal plumes and the delivery of iron to banded iron formation. The Journal of Geology 103, 169–185.
Johannesson, K.H., Hawkins, D.L., Cortes, A. (2006) Do Archean sediments record ancient seawater rare earth element patterns? Geochimica et Cosmochimica Acta 70, 871–890.
Johnston, D.T., Poulton, S.W., Fralick, P.W., Wing, B.A. Canfield, D.E., Farquhar, J. (2006) Evolution of the oceanic sulfur cycle at the end of the Paleoproterozoic. Geochimica et Cosmochimica Acta 70, 5723–5739.
Mancuso, J.J. (1989) Precambrian vein pyrobitumen: evidence for petroleum generation and migration 2 Ga ago. Precambrian Research 44, 137–146.
Morse, J.W., Wang, Q. (1997) Pyrite formation under conditions approx.imating those in anoxic sediments: ?. Influence of precursor iron minerals and organic matter. Marine Chemistry 57, 187–193.
Nelson, G.J., Pufahl, P.K., Hiatt, E.E. (2010) Paleoceanographic constraints on Precambrian phosphorite accumulation, Baraga Group, Michigan, USA. Sedimentary Geology 226, 9–21.
O’Day, P.A. (2006) Chemistry and mineralogy of arsenic. Elements 2, 77–84.
Ohfuji, H., Rickard, D. (2005) Experimental syntheses of framboids – a review. Earth-Science Reviews 71, 147–170.
Ohmoto, H., Watanabe, Y., Kumazawa, K. (2004) Evidence from massive siderite beds for a CO2-rich atmosphere before -1.8 billion years ago. Nature 429, 395–399.
Ojakangas, R.W. (1994) Sedimentology and provenance of the Early Proterozoic Michigamme Formation and the Goodrich Quartzite—Northern Michigan-Regional Stratigraphic Implications and Suggested Correlations. U.S. Geological Survey Bulletin 1904-R, 31 pp.
Ojakangas, R.W., Morey, G.B., Southwick, D.L. (2001) Paleoproterozoic basin development and sedimentation in the Lake Superior region, North America. Sedimentary Geology 141–142, 319–341.
Paytan, A., McLaughlin, K. (2007) The oceanic phosphorus cycle. Chemical Reviews 107, 563-576.
Planavsky, N., Rouxel, O., Bekker, A., Shapiro, R., Fralick, P., Knudsen, A. (2009) Iron-oxidizing microbial ecosystems thrived in late Paleoproterozoic redox-stratified oceans. Earth Planetary Science Letters 286, 230–242.
Poulton, S.W., Fralick, P.W., Canfield, D.E. (2004) The transition to a sulphidic ocean, ~1.84 billion years ago. Nature 431, 173–177.
Poulton, S.W., Fralick, P.W., Canfield, D.E. (2010) Spatial variability in oceanic redox structure 1.8 billion years ago. Nature Geoscience 3, 486–490.
Pourmand, A., Dauphas, N., Ireland T.J. (2012) A novel extraction chromatography and MC-ICP-MS technique for rapid analysis of REE, Sc and Y: Revising C1-chondrite and Post-Archean Australian Shale. Chemical Geology 291, 38–54.
Pufahl, P., Fralick, P. (2000) Field Trip 4: Depositional Environments of the Paleoproterozoic Gunflint Formation. In: P. Fralick (Ed.) Institute on Lake Superior Geology Proceedings, 46th Annual Meeting, Thunder Bay, Ontario. Part 2 - Field Trip Guidebook. Institute on Lake Superior Geology, Minnesota, 98–141.
Pufahl, P.K., Hiatt, E.E., Kyser, T.K. (2010). Does the Paleoproterozoic Animikie Basin record the sulfidic ocean transition? Geology 38, 659–662.
Raiswell, R., Berner, R.A. (1986) Pyrite and organic matter in Phanerozoic normal marine shales. Geochimica et Cosmochimica Acta 50, 1967–1976.
Rasmussen, B., Fletcher, I.R., Bekker, A., Muhling, J.R., Gregory, C.J., Thorne, A.M. (2012) Deposition of 1.88-billion-year-old iron formations as a consequence of rapid crustal growth. Nature 484, 498–501.
Schopf, J.W., Klein, C. (1992) The Proterozoic Biosphere. A Multidisciplinary Study. Cambridge University Press, Cambridge.
Schulz, K.J., Cannon, W.F. (2007) The Penokean orogeny in the Lake Superior region. Precambrian Research 157, 4–25.
Shield, G., Veizer, J. (2002) Precambrian marine carbonate isotope database: Version 1.1. Geochemistry, Geophysics, Geosystems 3, 1–12.
Simonson, B.M. (1985) Sedimentological constrains on the origins of Precambrian iron-formations. Geological Society of America Bulletin 98, 244–252.
Tyler S.A., Barghoorn, E.S. (1954) Occurrence of Structurally Preserved Plants in Precambrian Rocks of the Canadian Shield. Science 30, 608–609.
Vandenbroucke, M. (2003) Kerogen: from Types to Models of Chemical Structure. Oil & Gas Science and Technology – Rev. IFP 58, 243–269.
Van Wyck, N., Johnson, C.M. (1997) Common lead, Sm-Nd, and U-Pb constraints on petrognesis, crustal architecture, and tectonic setting of the Penokean orogeny (Paleoproterozoic) in Wisconsin. Geological Society of America Bulletin 109, 799–808.
Wacey, D., McLoughlin, N., Kilburn, M.R., Saunders, M., Cliff, J.B., Kong, C., Barley, M.E., Brasier, M.D. (2013) Nanoscale analysis of pyritized microfossils reveals differential heterotrophic consumption in the ~1.9-Ga Gunflint chert. Proceedings of the National Academy of Science 110, 8020–8024.
Wacey, D., Saunders, M., Kong, C., Kilburn, R. (2016) A new occurrence of ambient inclusion trails from the ~1900-million-year-old Gunflint Formation, Ontario: nanocharacterization and testing of potential formation mechanisms. Geobiology 14, 440–456.
Williford, K.H., Ushikubo, T., Schopf, J.W., Lepot, K., Kitajima, K., Valley, J.W. (2013) Preservation and detection of microstructural and taxonomic correlations in the carbon isotopic compositions of individual Precambrian microfossils. Geochimica et Cosmochimica Acta 104, 165–182.
Winter, B.L., Knauth, L.P. (1992) Stable isotope geochemistry of early Proterozoic carbonate concretions in the Animiki Group of the Lake Superior region: evidence for anaerobic bacterial processes. Precambrian Research 54, 131–151.
Figures and Tables
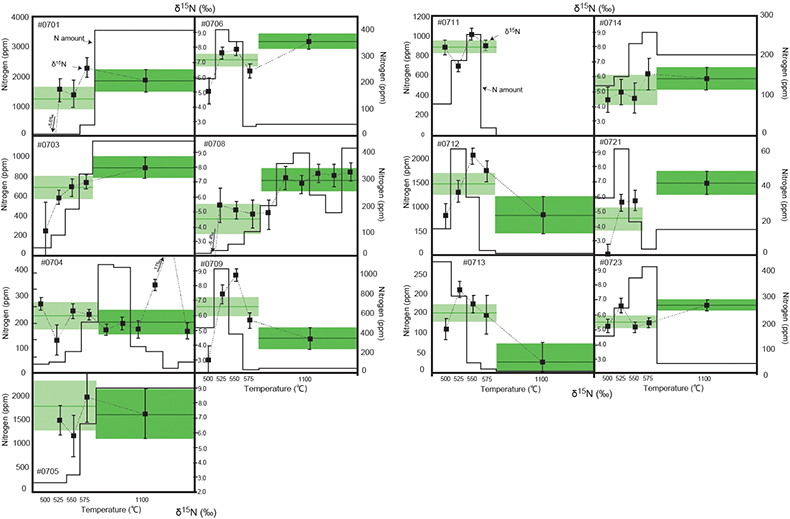
Figure 1 Results of the stepwise combustion analyses of 13 kerogen samples from the Gunflint Formation (samples #0704 and #0708 are cited from Ishida et al., 2012
Ishida, A., Hashizume, K., Kakegawa, T. (2012) Stepwise combustion analyses of distinct nitrogen isotopic compositions on Paleoproterozoic organic matter, Geochemical Journal 46, 249–253.
). Nitrogen concentrations (white bars) and d15N values (black squares) are shown. The average d15N values and errors of both the lower-temperature and the higher-temperature fractions are shown as light green and dark green bars, respectively. Original data are summarised in Table S-2.Table 1 Summary of light and trace element concentrations and the isotope compositions of bulk rock and kerogen samples.
section | sample ID | litho facies | bulk rock | kerogen | |||||||||||||
Total C | TOC | Ccarb | Spyr | Pr/Sm | Eu/Eu* | C | N | H | S | C/N | d13C | d34S (pyr) | d15N | ± | |||
wt. % | (PAAS normalised)1 | wt. % | atom | ‰ | |||||||||||||
Pass Lake Road | 0716 | Chert | 0.1 | 0.0 | 0.1 | 0.0 | 1.22 | 0.87 | n.a. | n.a. | n.a. | n.a. | - | n.a. | n.a. | n.a. | n.a. |
0718 | Carbonate | 6.6 | 0.0 | 6.6 | 0.0 | 0.61 | 2.22 | n.a. | n.a. | n.a. | n.a. | - | n.a. | n.a. | n.a. | n.a. | |
0719 | Silicified carbonate | 3.1 | 0.0 | 3.1 | 0.0 | 0.66 | 2.24 | n.a. | n.a. | n.a. | n.a. | - | n.a. | n.a. | n.a. | n.a. | |
0720 | Silicified carbonate | 3.7 | 0.1 | 3.6 | 0.0 | 0.67 | 2.18 | n.a. | n.a. | n.a. | n.a. | - | n.a. | n.a. | n.a. | n.a. | |
0721 | Taconite | 3.0 | 0.2 | 2.9 | 0.6 | 0.82 | 1.78 | 16.8 | 0.2 | 0.3 | 32.6 | 109.6 | -32.6 | 3.0 | 4.7 | 0.60 | |
0722 | Chert (BIF) | 0.2 | 0.0 | 0.2 | 0.0 | 0.88 | 1.73 | n.a. | n.a. | n.a. | n.a. | - | n.a. | n.a. | 6.8 | 1.00 | |
0723 | Carbonate (BIF) | 5.2 | 0.1 | 5.1 | 0.1 | 0.82 | 1.41 | n.a. | n.a. | n.a. | n.a. | - | -25.1 | n.a. | 5.4 | 0.40 | |
Blend River North | 0301 | Black shale | 0.3 | 0.3 | 0.0 | 0.0 | 1.76 | 1.25 | 70.7 | 1.2 | 3.5 | 5.6 | 67.4 | -31.6 | 2.1 | 2.9 | 0.70 |
0302 | Black shale | 0.8 | 0.3 | 0.5 | 0.2 | 0.49 | 1.48 | 53.1 | 0.6 | 2.4 | 18.2 | 96.2 | -31.6 | 4.7 | n.a. | n.a. | |
0303 | Ferruginous shale | 4.1 | 0.3 | 3.8 | 0.1 | 0.76 | 1.54 | 69.4 | 1.2 | 3.2 | 9.6 | 67.6 | -31.3 | 7.1 | n.a. | n.a. | |
0304 | Black shale | 1.1 | 0.9 | 0.1 | 0.0 | 1.43 | 1.19 | 73.1 | 1.1 | 3.2 | 3.2 | 75.5 | -32.1 | 8.6 | 5.9 | 0.70 | |
0305 | Carbonate | 9.1 | 0.8 | 8.3 | 0.4 | 0.69 | 1.59 | 49.8 | 0.9 | 2.0 | 18.8 | 64.7 | -31.9 | 8.2 | 6.5 | 0.70 | |
0306 | Ferruginous carbonate | 10.4 | 0.3 | 10.2 | 0.1 | 0.78 | 1.32 | 60.5 | 1.1 | 2.9 | 13.3 | 63.9 | -29.8 | n.a. | n.a. | n.a. | |
0307 | Silicified carbonate | 5.0 | 0.1 | 4.9 | 0.0 | 0.78 | 1.54 | n.a. | n.a. | n.a. | n.a. | - | n.a. | n.a. | n.a. | n.a. | |
0308 | Carbonate/shale | 4.0 | 0.0 | 3.9 | 0.0 | 0.83 | 1.18 | 44.1 | 0.0 | 0.0 | 15.2 | - | n.a. | n.a. | n.a. | n.a. | |
0309 | Ferruginous carbonate | 9.9 | 0.2 | 9.7 | 0.0 | 0.83 | 1.27 | 76.0 | 1.4 | 3.5 | 5.7 | 65.6 | n.a. | n.a. | n.a. | n.a. | |
0310 | Chert | 3.2 | 0.1 | 3.1 | 0.0 | 0.83 | 1.29 | n.a. | n.a. | n.a. | n.a. | - | n.a. | n.a. | n.a. | n.a. | |
0311 | Chert | 1.9 | 0.0 | 1.8 | 0.0 | 0.68 | 0.72 | 60.3 | 0.7 | 2.2 | 8.5 | 94.9 | n.a. | n.a. | n.a. | n.a. | |
Current River | 0701 | Carbonate-rich arkosic sandstone | 4.7 | 0.5 | 4.2 | 0.0 | 0.86 | 1.28 | 67.3 | 0.9 | 2.2 | 5.6 | 91.2 | -33.0 | 26.9 | 5.6 | 0.80 |
0702 | Arkosic sandstone | 0.8 | 0.2 | 0.6 | 0.0 | 1.87 | 1.11 | 56.6 | 0.6 | 3.2 | 2.5 | 101.7 | -32.2 | 8.3 | 6.7 | 0.70 | |
0703 | Sandy carbonate | 4.2 | 0.2 | 4.0 | 0.0 | 0.99 | 1.24 | 82.7 | 1.1 | 3.7 | 2.8 | 88.5 | -32.3 | 10.9 | 7.1 | 0.50 | |
0704 | Sandy carbonate | 5.9 | 0.2 | 5.7 | 0.0 | 0.98 | 1.18 | 82.9 | 1.0 | 3.8 | 3.2 | 98.4 | -32.5 | 11.2 | 4.9 | 1.00 | |
0705 | Sandy carbonate | 4.4 | 0.1 | 4.3 | 0.0 | 1.23 | 1.42 | 52.6 | 0.7 | 2.2 | 14.8 | 86.8 | -31.6 | 9.7 | 6.0 | 0.70 | |
0706 | Sandy carbonate | 5.4 | 0.3 | 5.1 | 0.2 | 1.03 | 1.52 | 47.8 | 0.6 | 2.0 | 19.6 | 87.6 | -32.6 | 15.1 | 6.9 | 0.60 | |
0707 | Sandy carbonate | 2.9 | 0.2 | 2.8 | 0.0 | 0.92 | 1.18 | 66.1 | 0.8 | 2.8 | 10.2 | 98.3 | -31.9 | 5.8 | 5.9 | 0.80 | |
0708 | Sandy carbonate | 7.1 | 0.3 | 6.7 | 0.0 | 0.76 | 1.76 | 85.7 | 1.0 | 4.1 | 1.6 | 98.8 | -32.9 | 16.0 | 6.9 | 0.50 | |
0709 | Laminated carbonate with detrital quartz | 1.8 | 0.2 | 1.6 | 0.1 | 1.03 | 1.31 | 59.9 | 0.8 | 2.5 | 14.2 | 86.7 | -32.4 | 14.8 | 7.3 | 0.60 | |
0710 | Laminated carbonate with detrital quartz | 0.3 | 0.1 | 0.2 | 0.0 | 1.18 | 1.48 | 72.1 | 1.0 | 4.4 | 5.9 | 80.3 | -32.0 | - | 3.2 | 0.60 | |
0711 | Massive carbonate | 9.0 | 0.1 | 8.9 | 0.1 | 0.93 | 1.63 | 54.3 | 0.8 | 2.9 | 14.6 | 80.9 | -32.6 | 13.4 | 8.0 | 0.40 | |
0712 | Laminated carbonate with detrital quartz | 0.7 | 0.3 | 0.4 | 0.1 | 1.16 | 1.63 | 66.0 | 1.0 | 3.4 | 9.4 | 78.3 | -32.5 | 11.3 | 7.2 | 0.30 | |
0713 | Carbonate shale (phosphorous) | 6.2 | 0.2 | 6.0 | 0.1 | 1.02 | 1.68 | 43.2 | 0.6 | 1.8 | 20.7 | 90.1 | -32.4 | 9.2 | 6.4 | 0.60 | |
0714 | Black shale | 4.5 | 0.9 | 3.7 | 0.1 | 1.42 | 1.22 | 68.6 | 1.0 | 3.0 | 4.8 | 78.2 | -33.6 | 9.3 | 4.8 | 0.90 | |
0715 | Black shale | 0.2 | 0.3 | 0.0 | 0.0 | 1.20 | 1.28 | 8.2 | 0.2 | 1.2 | 1.6 | 61.3 | -32.2 | 11.5 | 5.9 | 0.70 | |
Telly Fox | 0724 | Carbonate | 9.7 | 0.3 | 9.3 | 0.2 | 0.93 | 1.53 | 37.8 | 0.6 | 1.7 | 25.4 | 77.5 | -31.4 | -1.1 | n.a. | n.a. |
0725 | Silicified shale | 0.2 | 0.2 | 0.0 | 0.4 | 0.63 | 1.23 | n.a. | n.a. | n.a. | n.a. | - | -31.5 | 20.7 | 5.5 | 0.70 | |
0726 | Carbonate/shale | 0.3 | 0.4 | 0.0 | 0.2 | 0.71 | 1.40 | 60.1 | 0.9 | 2.9 | 12.5 | 78.6 | -31.6 | 10.8 | 4.5 | 0.30 | |
0729 | Silicified carbonate | 0.3 | 0.1 | 0.3 | 0.1 | 0.24 | 0.72 | n.a. | n.a. | n.a. | n.a. | - | -28.9 | n.a. | 4.3 | 0.30 | |
0730 | Silicified carbonate | 0.3 | 0.1 | 0.2 | 0.0 | 0.20 | 0.99 | n.a. | n.a. | n.a. | n.a. | - | n.a. | n.a. | n.a. | n.a. | |
Kakabeka Fall | 0601 | Shale (BIF) | 7.3 | 0.8 | 6.6 | 0.2 | 0.92 | 1.67 | 64.8 | 1.2 | 2.8 | 9.9 | 62.5 | -31.3 | 5.9 | 6.3 | 0.80 |
0602 | Chert (BIF) | 0.3 | 0.1 | 0.2 | 0.0 | 0.41 | 0.87 | 57.5 | 1.0 | 2.5 | 8.5 | 68.2 | -29.7 | n.a. | n.a. | n.a. | |
0603 | Shale (BIF) | 5.5 | 0.4 | 5.1 | 0.1 | 0.84 | 1.46 | 60.6 | 1.0 | 2.0 | 12.2 | 70.8 | -30.9 | 6.1 | n.a. | n.a. | |
0604 | Ferruginous chert | 2.7 | 0.1 | 2.6 | 0.1 | 1.16 | 1.37 | 46.4 | 0.9 | 1.6 | 15.6 | 60.3 | -27.8 | n.a. | n.a. | n.a. | |
0605 | Ferruginous chert | 0.0 | 0.0 | 0.0 | 0.0 | 0.75 | 0.72 | n.a. | n.a. | n.a. | n.a. | - | n.a. | n.a. | n.a. | n.a. |
Analytical error of d13C and d34S are within 0.2 ‰.
Underlined values: below instumental reproducibility (0.1 %), n.a.: not analysed because of low amount of organic matter.
1 Details are described in Tables S-1 and S-4.
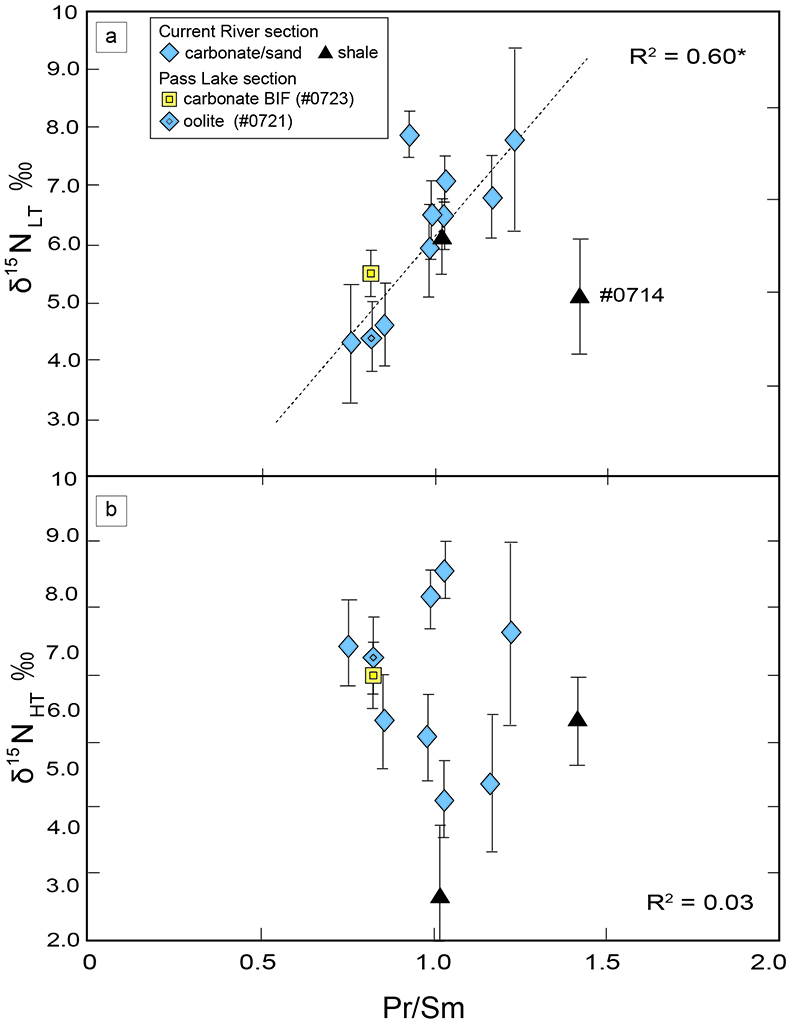
Figure 2 Correlation diagrams between the Pr/Sm ratios and (a) the d15NLT values and (b) the d15NHT values, respectively. A positive correlation is observed between the Pr/Sm ratios and the d15NLT values (R2 = 0.60, excluding #0714), whereas no correlation is observed between the d15NHT values.

Figure 3 Correlation diagrams between the Pr/Sm ratios and (a) the Eu/Eu* ratios, (b) TiO2, and (c) Zr concentrations. The Eu/Eu* is defined as Eu/0.5(Gd+Sm). Grey square areas indicate the range of modern ocean values. Dashed and solid arrows indicate the directions of hydrothermal fluid and crustal contributions, respectively (Bolhar et al., 2005
Bolhar, R., Van Krenendonk, M., Kamber, B.S. (2005) A trace element study of siderite-jasper banded iron formation in the 3.45 Ga Warrawoona Group, Pilbara Craton-Formation from hydrothermal fluids and shallow seawater. Precambrian Research 137, 93–114.
). Positive correlations exist between the Pr/Sm and TiO2 and Zr concentrations.Back to article
Supplementary Figures and Tables
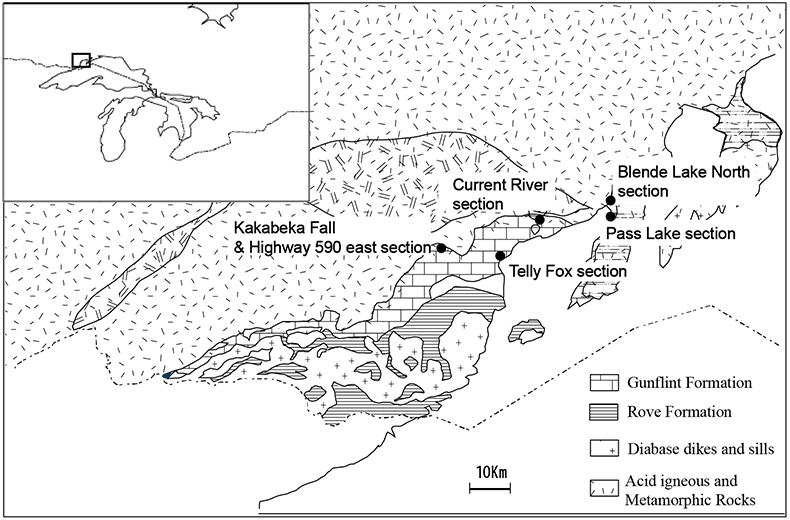
Figure S-1 Geological map of the Thunder Bay region (modified after Geological map of Thunder Bay district “Map 2065 ATIKOKAN-LAKEHEAD SHEET Geological Compilation Series”). Geological surveys were conducted in five sections: Pass Lake section; Blende Lake North section; Current River section; Terry Fox section; Kakabeka Falls and Highway 590 east section.
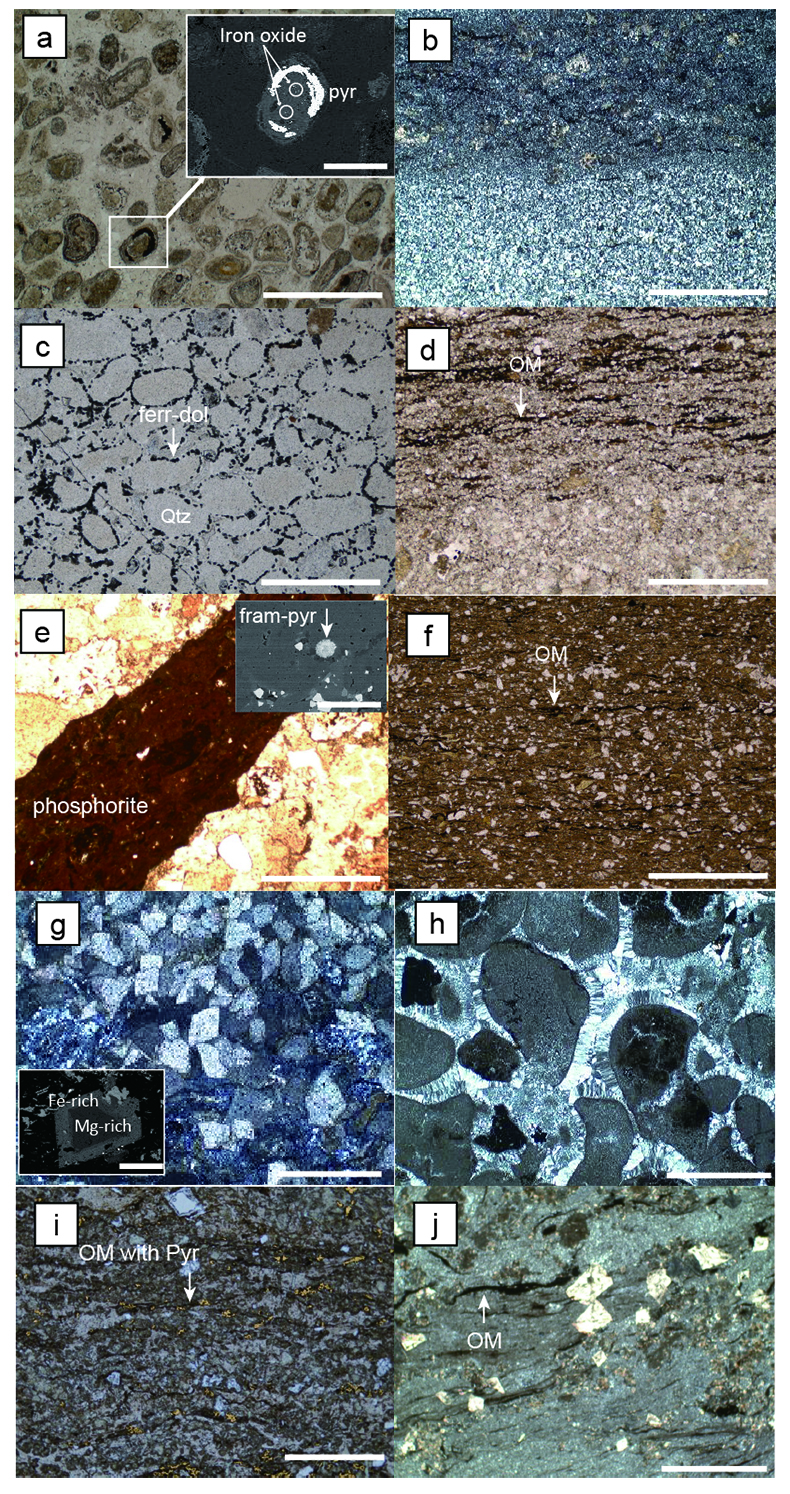
Figure S-2 Thin section images of representative samples. Scale bars in each photo are 1 millimetre unless otherwise noted. (a) Sample #0721: Oolite from Pass Lake section. Fe-rich dolomite covers lithic core concentrically in a quartz matrix. Inserted image: Back-scattered electron (BSE) image of ooid grain. Pyrite is contained in the rim of an ooid grain. Ooid grains contain tiny haematite crystals in the carbonate rims. Scale bar is 200 µm. (b) Sample #0722: BIF iron layer from Pass Lake section. The upper iron-rich layer consists of haematite in a microcrystalline quartz matrix. The lower chert layer consists of microcrystalline quartz. (c) Sample #0704: Sandstone from the Current River section. Sandy grains consist of microcrystalline quartz. Each diameter is approximately 50 to 100 µm. Tiny ferrodolomitic carbonate grains surround the grains. (d) Sample #0709: Organic matter (OM) is observed with detrital lithic fragments, such as quartz and clay minerals. The matrix is carbonate mud. (e) Sample #0713: Phosphorite pebble from the Current River section. The matrix consists of ferrodolomite and detrital quartz. The phosphorite pebbles consist of an apatite matrix, fragments of quartz, clay minerals, OM, and pyrite. Inserted image: Framboidal pyrite found around the phosphorite (white arrow). Both euhedral and framboidal pyrite crystals are observed. Arsenic is present in the framboidal pyrite as a minor component (less than 2 wt. %), but not in the euhedral pyrite. Scale bar is 50 µm. (f) Sample #0715: Finely laminated sedimentary layers with OM. The matrix consists of quartz and chlorite. Carbonate minerals and pyrite are scarce. (g) Sample #0726: Ferrodolomitic layer in BIF from the Terry Fox section. Euhedral dolomite crystals are present in a microcrystalline quartz matrix. Inserted image: BSE image of single ferrodolomite crystal. Outer rim of crystal is richer in iron than the crystal interior. Scale bar is 100 µm. (h) Sample #0726: Chert layer in a BIF sample. Microcrystalline quartz grains with fibrous cement are observed in a quartz matrix. (i) Sample #0725: Black chert from the Terry Fox section (reflected light). OM is present in a chert matrix. Pyrite crystals are observed in the OM layer. (j) Sample #0601: Iron layer from the Kakabeka Falls section. Matrix consists of iron-rich silicate. OM exists as a laminated structure. Minor siderites were observed with no relation to the stratigraphic layer.
Table S-1 Summary of major and trace element concentrations of bulk rock samples. Eu/Eu* is defined as Eu/0.5(Gd+Sm), Pr/Pr* is defined as Pr/0.5(Ce+Nd), and Ce/Ce* is defined as Ce/0.5(La+Pr). Values are normalised by PAAS reported by Pourmand et al. (2012).
sample ID | SiO2 | Al2O3 | Fe2O3 | MnO | MgO | CaO | Na2O | K2O | TiO2 | P2O5 |
wt. % | ||||||||||
0716 | 97.74 | 0.24 | 0.85 | 0.009 | 0.02 | 0.15 | 0.08 | 0.12 | 0.009 | 0.02 |
0718 | 43.08 | 0.19 | 6.18 | 1.341 | 7.98 | 15.83 | 0.11 | 0.05 | 0.005 | 0.01 |
0719 | 74.27 | 0.13 | 2.88 | 0.537 | 3.91 | 7.57 | 0.09 | 0.04 | 0.001 | 0.01 |
0720 | 68.08 | 0.13 | 5.16 | 0.715 | 4.34 | 8.71 | 0.08 | 0.07 | 0.005 | 0.01 |
0721 | 73.66 | 0.11 | 4.98 | 0.766 | 3.06 | 6.87 | 0.01 | 0.01 | 0.005 | 0.01 |
0722 | 97.15 | 0.31 | 0.69 | 0.017 | 0.01 | 1.07 | 0.05 | 0.04 | 0.001 | 0.02 |
0723 | 54.19 | 0.11 | 21.39 | 1.388 | 3.55 | 2.93 | 0.01 | 0.06 | 0.009 | 0.53 |
0301 | 63.37 | 14.67 | 8.31 | 0.082 | 1.89 | 0.18 | 0.11 | 5.74 | 1.167 | 0.09 |
0302 | 48.44 | 15.94 | 15.22 | 0.23 | 2.1 | 0.99 | 0.08 | 8.14 | 2.523 | 0.34 |
0303 | 45.29 | 5.22 | 26.32 | 1.17 | 3.42 | 1.93 | 0.02 | 0.5 | 0.255 | 0.1 |
0304 | 55.12 | 14.02 | 15.48 | 0.212 | 2.66 | 0.57 | 0.07 | 3.6 | 0.659 | 0.06 |
0305 | 16.74 | 1.27 | 28.04 | 2.83 | 6.82 | 12.75 | 0.13 | 0.06 | 0.012 | 1.23 |
0306 | 2.80 | 0.94 | 43.48 | 3.567 | 6.96 | 6.75 | 0.24 | 0.06 | 0.006 | 0.33 |
0307 | 51.43 | 0.04 | 24.87 | 1.602 | 2.45 | 2.58 | 0.02 | 0.01 | 0.003 | 0.75 |
0308 | 62.17 | 0.22 | 17.06 | 1.31 | 2.54 | 2.68 | 0.2 | 0.04 | 0.007 | 0.15 |
0309 | 5.04 | 1.43 | 45.22 | 3.325 | 6.88 | 5.49 | 0.01 | 0.01 | 0.003 | 0.34 |
0310 | 70.31 | 0.08 | 16.22 | 0.9 | 1.74 | 1.1 | 0.01 | 0.01 | 0.003 | 0.16 |
0311 | 82.50 | 0.1 | 9.69 | 0.594 | 0.98 | 0.56 | 0.22 | 0.04 | 0.007 | 0.07 |
0701 | 50.14 | 2.22 | 25.99 | 1.067 | 2.93 | 1.41 | 0.02 | 0.02 | 0.091 | 0.13 |
0702 | 71.07 | 5.23 | 14.79 | 0.107 | 2.66 | 1.19 | 0.04 | 0.06 | 0.222 | 0.04 |
0703 | 52.52 | 2.73 | 21.16 | 0.613 | 4.07 | 4.36 | 0.08 | 0.03 | 0.132 | 0.03 |
0704 | 41.28 | 1.47 | 27.22 | 0.836 | 4.29 | 4.85 | 0.12 | 0.04 | 0.05 | 0.07 |
0705 | 44.56 | 3.06 | 30.28 | 0.668 | 3.55 | 1.29 | 0.03 | 0.03 | 0.124 | 0.13 |
0706 | 45.52 | 2.04 | 13.18 | 0.438 | 5.39 | 12.47 | 0.01 | 0.07 | 0.07 | 0.09 |
0707 | 62.39 | 2.93 | 16.18 | 0.463 | 3.19 | 4.05 | 0.02 | 0.01 | 0.225 | 0.03 |
0708 | 26.51 | 2.98 | 29.33 | 0.87 | 6.96 | 8.26 | 0.09 | 0.06 | 0.083 | 0.09 |
0709 | 65.35 | 4.7 | 19.08 | 0.232 | 2.74 | 0.74 | 0.12 | 0.19 | 0.2 | 0.11 |
0710 | 74.91 | 5.75 | 10.75 | 0.03 | 1.83 | 0.47 | 0.1 | 1.22 | 0.355 | 0.07 |
0711 | 15.22 | 2.16 | 15.78 | 0.747 | 9.15 | 22.39 | 0.12 | 0.3 | 0.113 | 0.02 |
0712 | 75.36 | 5.53 | 10.47 | 0.071 | 1.7 | 0.66 | 0.07 | 1.73 | 0.29 | 0.05 |
0713 | 39.03 | 1.46 | 18.8 | 0.792 | 5.68 | 10.88 | 0.09 | 0.12 | 0.055 | 0.45 |
0714 | 50.18 | 4.99 | 23.59 | 0.458 | 3.53 | 2.95 | 0.03 | 0.62 | 0.164 | 0.06 |
0715 | 69.70 | 11.04 | 8.86 | 0.01 | 1.72 | 0.14 | 0.01 | 5.22 | 0.641 | 0.08 |
0724 | 18.30 | 0.29 | 11.3 | 1.524 | 10.85 | 22.79 | 0.03 | 0.01 | 0.009 | 0.54 |
0725 | 76.26 | 5.49 | 12.97 | 0.023 | 2.67 | 0.18 | 0.06 | 0.07 | 0.139 | 0.08 |
0726 | 44.25 | 1.91 | 6.22 | 0.484 | 8.91 | 14.81 | 0.06 | 0.55 | 0.065 | 0.03 |
0729 | 96.74 | 0.16 | 1.29 | 0.024 | 0.1 | 1.08 | 0.45 | 0.06 | 0.007 | 0.02 |
0730 | 96.60 | 0.13 | 0.68 | 0.017 | 0.01 | 0.69 | 0.14 | 0.03 | 0.005 | 0.03 |
0601 | 27.53 | 2.95 | 39.9 | 0.46 | 5.3 | 1.43 | 0.14 | 0.14 | 0.053 | 0.33 |
0602 | 96.59 | 0.34 | 1.79 | 0.019 | 0.08 | 0.03 | 0.13 | 0.08 | 0.009 | 0.02 |
0603 | 47.39 | 0.64 | 30.08 | 0.534 | 2.58 | 1.58 | 0.13 | 0.11 | 0.026 | 0.36 |
0604 | 72.91 | 0.31 | 16.58 | 0.352 | 1.12 | 0.78 | 0.04 | 0.06 | 0.01 | 0.17 |
0605 | 84.92 | 0.09 | 13.26 | 0.014 | 0.06 | 0.01 | 0.08 | 0.01 | 0.005 | 0.02 |
sample ID | La | Ce | Pr | Nd | Sm | Eu | Gd | Tb | Dy | Y | Ho | Er | Tm | Yb | Lu | SREE | Pr/Sm | Eu/Eu* | Ce/Ce* | Pr/Pr* |
ppm | (PAAS normalised) | |||||||||||||||||||
0716 | 1 | 3.2 | 0.18 | 0.6 | 0.1 | 0.05 | 0.1* | 0.1* | 0.1* | 2* | 0.1* | 0.1* | 0.05* | 0.1* | 0.04* | 5.13 | 1.22 | 0.87 | 1.81 | 0.68 |
0718 | 8.5 | 18.3 | 2.15 | 9.3 | 2.4 | 1.04 | 2.7 | 0.4 | 2.5 | 18 | 0.5 | 1.2 | 0.14 | 0.7 | 0.08 | 67.91 | 0.61 | 2.22 | 1.03 | 0.93 |
0719 | 5.7 | 11.5 | 1.27 | 5.1 | 1.3 | 0.55 | 1.5 | 0.2 | 1.2 | 7 | 0.2 | 0.6 | 0.08 | 0.4 | 0.05 | 36.65 | 0.66 | 2.24 | 1.03 | 0.94 |
0720 | 5.1 | 10 | 1.19 | 4.7 | 1.2 | 0.51 | 1.3 | 0.2 | 1.5 | 9 | 0.3 | 0.9 | 0.12 | 0.6 | 0.08 | 36.7 | 0.67 | 2.18 | 0.98 | 0.98 |
0721 | 3.1 | 6.1 | 0.85 | 2.7 | 0.7 | 0.31 | 0.9 | 0.2 | 1 | 7 | 0.2 | 0.7 | 0.1 | 0.5 | 0.07 | 24.43 | 0.82 | 1.78 | 0.90 | 1.18 |
0722 | 1.9 | 4.1 | 0.39 | 1.5 | 0.3 | 0.14 | 0.4 | 0.1* | 0.3 | 2* | 0.1* | 0.2 | 0.05* | 0.1* | 0.04* | 9.23 | 0.88 | 1.73 | 1.15 | 0.89 |
0723 | 4.9 | 11.9 | 1.09 | 4.1 | 0.9 | 0.28 | 1.1 | 0.2 | 0.9 | 7 | 0.2 | 0.5 | 0.07 | 0.3 | 0.04* | 33.44 | 0.82 | 1.41 | 1.24 | 0.88 |
0301 | 47.5 | 80.7 | 6.49 | 17.2 | 2.5 | 0.6 | 2 | 0.4 | 2.5 | 16 | 0.5 | 1.7 | 0.27 | 1.9 | 0.27 | 180.53 | 1.76 | 1.25 | 1.07 | 0.93 |
0302 | 16.9 | 50.9 | 6.47 | 31.4 | 8.9 | 2.37 | 8.4 | 1.2 | 6.5 | 33 | 1.3 | 3.6 | 0.55 | 3.5 | 0.5 | 175.49 | 0.49 | 1.48 | 1.13 | 0.90 |
0303 | 12.1 | 26.6 | 2.79 | 11.2 | 2.5 | 0.67 | 2.3 | 0.3 | 1.8 | 11 | 0.3 | 1 | 0.14 | 0.9 | 0.13 | 73.73 | 0.76 | 1.54 | 1.10 | 0.91 |
0304 | 26.6 | 42.7 | 3.37 | 9.8 | 1.6 | 0.39 | 1.4 | 0.3 | 2 | 14 | 0.4 | 1.4 | 0.23 | 1.5 | 0.22 | 105.91 | 1.43 | 1.19 | 1.04 | 0.89 |
0305 | 21.8 | 62.5 | 8.43 | 36.6 | 8.3 | 2.36 | 8.1 | 1.1 | 5.7 | 36 | 1 | 2.5 | 0.29 | 1.5 | 0.2 | 196.38 | 0.69 | 1.59 | 1.07 | 0.98 |
0306 | 7.9 | 17.2 | 1.62 | 6.1 | 1.4 | 0.4 | 1.5 | 0.3 | 1.6 | 11 | 0.3 | 0.9 | 0.13 | 0.7 | 0.1 | 51.15 | 0.78 | 1.32 | 1.16 | 0.89 |
0307 | 3.3 | 7.7 | 0.69 | 2.6 | 0.6 | 0.18 | 0.6 | 0.1* | 0.5 | 5 | 0.1* | 0.3 | 0.05* | 0.1* | 0.04* | 21.47 | 0.78 | 1.54 | 1.23 | 0.87 |
0308 | 2.5 | 5.7 | 0.49 | 1.7 | 0.4 | 0.11 | 0.4 | 0.1* | 0.4 | 3 | 0.1* | 0.3 | 0.05* | 0.3 | 0.04* | 15.3 | 0.83 | 1.18 | 1.24 | 0.88 |
0309 | 10.5 | 24.3 | 2.32 | 8.6 | 1.9 | 0.46 | 1.9 | 0.3 | 2 | 15 | 0.4 | 1.2 | 0.16 | 1 | 0.14 | 70.18 | 0.83 | 1.27 | 1.19 | 0.90 |
0310 | 2.3 | 5.6 | 0.49 | 1.8 | 0.4 | 0.12 | 0.4 | 0.1* | 0.3 | 3 | 0.1* | 0.2 | 0.05* | 0.1* | 0.04* | 14.61 | 0.83 | 1.29 | 1.27 | 0.86 |
0311 | 1 | 2.5 | 0.2 | 0.7 | 0.2 | 0.05 | 0.1* | 0.1* | 0.1* | 2* | 0.1* | 0.1* | 0.05* | 0.1* | 0.04* | 4.65 | 0.68 | 0.72 | 1.34 | 0.84 |
0701 | 2.9 | 8 | 0.76 | 2.9 | 0.6 | 0.15 | 0.5 | 0.1* | 0.4 | 3 | 0.1* | 0.3 | 0.05* | 0.2 | 0.04* | 19.71 | 0.86 | 1.28 | 1.30 | 0.89 |
0702 | 15.1 | 27.8 | 1.65 | 4.1 | 0.6 | 0.13 | 0.3 | 0.1* | 0.4 | 3 | 0.1* | 0.3 | 0.05* | 0.3 | 0.05 | 53.73 | 1.87 | 1.11 | 1.26 | 0.77 |
0703 | 3.4 | 8.5 | 0.73 | 2.4 | 0.5 | 0.13 | 0.4 | 0.1* | 0.4 | 3 | 0.1* | 0.3 | 0.05* | 0.3 | 0.04* | 20.06 | 0.99 | 1.24 | 1.30 | 0.90 |
0704 | 2.7 | 6.5 | 0.58 | 1.9 | 0.4 | 0.11 | 0.3 | 0.1* | 0.3 | 2* | 0.1* | 0.2 | 0.05* | 0.1* | 0.04* | 12.99 | 0.98 | 1.18 | 1.25 | 0.92 |
0705 | 8.1 | 17.8 | 1.45 | 4.6 | 0.8 | 0.2 | 0.7 | 0.1* | 0.4 | 3 | 0.1* | 0.2 | 0.05* | 0.2 | 0.04* | 37.45 | 1.23 | 1.42 | 1.24 | 0.88 |
0706 | 7.8 | 16.5 | 1.52 | 5.1 | 1 | 0.25 | 0.8 | 0.1* | 0.6 | 4 | 0.1* | 0.3 | 0.05* | 0.3 | 0.04* | 38.17 | 1.03 | 1.52 | 1.15 | 0.93 |
0707 | 3.4 | 7.8 | 0.54 | 2 | 0.4 | 0.11 | 0.4 | 0.1* | 0.5 | 4 | 0.1* | 0.3 | 0.05* | 0.3 | 0.05 | 19.8 | 0.92 | 1.18 | 1.36 | 0.75 |
0708 | 4.1 | 10.6 | 1.12 | 4.4 | 1 | 0.29 | 1.1 | 0.1* | 0.7 | 5 | 0.1* | 0.3 | 0.05* | 0.2 | 0.04* | 28.81 | 0.76 | 1.76 | 1.19 | 0.93 |
0709 | 5.9 | 14.2 | 1.37 | 4.6 | 0.9 | 0.2 | 0.7 | 0.1* | 0.4 | 2* | 0.1* | 0.3 | 0.05* | 0.2 | 0.04* | 28.77 | 1.03 | 1.31 | 1.20 | 0.95 |
0710 | 6.8 | 14 | 1.22 | 3.8 | 0.7 | 0.19 | 0.5 | 0.1* | 0.5 | 3 | 0.1* | 0.7 | 0.05* | 0.3 | 0.05 | 31.76 | 1.18 | 1.48 | 1.16 | 0.92 |
0711 | 10.1 | 21.2 | 1.92 | 6.6 | 1.4 | 0.42 | 1.3 | 0.2 | 1.3 | 12 | 0.3 | 0.7 | 0.09 | 0.5 | 0.07 | 58.1 | 0.93 | 1.63 | 1.16 | 0.91 |
0712 | 5.6 | 11.6 | 1.03 | 3.2 | 0.6 | 0.19 | 0.5 | 0.1* | 0.5 | 4 | 0.1* | 0.3 | 0.05* | 0.3 | 0.04* | 27.82 | 1.16 | 1.63 | 1.16 | 0.93 |
0713 | 17.4 | 38.1 | 3.16 | 10.5 | 2.1 | 0.65 | 2 | 0.3 | 1.6 | 13 | 0.3 | 0.8 | 0.11 | 0.6 | 0.08 | 90.7 | 1.02 | 1.68 | 1.23 | 0.87 |
0714 | 18.2 | 30.4 | 2.51 | 7.3 | 1.2 | 0.23 | 0.8 | 0.1* | 0.4 | 2* | 0.1* | 0.3 | 0.05* | 0.2 | 0.04* | 61.54 | 1.42 | 1.22 | 1.05 | 0.92 |
0715 | 12.7 | 22.6 | 2.13 | 6.7 | 1.2 | 0.3 | 0.9 | 0.2 | 0.9 | 7 | 0.2 | 0.7 | 0.12 | 0.8 | 0.13 | 56.58 | 1.20 | 1.28 | 1.03 | 0.96 |
0724 | 8.1 | 17.7 | 1.51 | 5.2 | 1.1 | 0.34 | 1.4 | 0.2 | 1.4 | 12 | 0.3 | 0.8 | 0.1 | 0.6 | 0.07 | 50.82 | 0.93 | 1.53 | 1.21 | 0.88 |
0725 | 0.8 | 2.4 | 0.28 | 1.3 | 0.3 | 0.1 | 0.4 | 0.1* | 0.6 | 2* | 0.1* | 0.4 | 0.06 | 0.3 | 0.05 | 6.99 | 0.63 | 1.23 | 1.19 | 0.89 |
0726 | 3.8 | 8 | 0.84 | 3.3 | 0.8 | 0.26 | 0.9 | 0.2 | 1 | 7 | 0.2 | 0.6 | 0.08 | 0.4 | 0.06 | 27.44 | 0.71 | 1.40 | 1.08 | 0.92 |
0729 | 0.4 | 0.6* | 0.07 | 0.5 | 0.2 | 0.05 | 0.2 | 0.1* | 0.2 | 2* | 0.1* | 0.1* | 0.05* | 0.2 | 0.04* | 1.82 | 0.24 | 0.72 | 0.86 | 0.68 |
0730 | 0.3* | 0.6* | 0.09 | 0.7 | 0.3 | 0.08 | 0.3 | 0.1* | 0.2 | 2* | 0.1* | 0.1* | 0.05* | 0.2 | 0.04* | 1.87 | 0.20 | 0.99 | 0.87 | 0.69 |
0601 | 7.8 | 17.5 | 1.76 | 6.3 | 1.3 | 0.41 | 1.5 | 0.2 | 1.4 | 10 | 0.3 | 0.8 | 0.1 | 0.6 | 0.09 | 50.06 | 0.92 | 1.67 | 1.14 | 0.94 |
0602 | 0.3* | 0.6* | 0.06 | 0.2* | 0.1 | 0.05 | 0.1* | 0.1* | 0.1 | 2* | 0.1* | 0.1* | 0.05* | 0.1* | 0.04* | 0.31 | 0.41 | 0.87 | 1.08 | 0.97 |
0603 | 11.3 | 30.2 | 2.71 | 10.3 | 2.2 | 0.45 | 2.5 | 0.1* | 0.7 | 15 | 0.1* | 0.4 | 0.06 | 0.4 | 0.12 | 76.34 | 0.84 | 1.46 | 1.31 | 0.86 |
0604 | 4 | 11.6 | 1.03 | 3.2 | 0.6 | 0.16 | 0.6 | 0.1* | 0.7 | 4 | 0.1* | 0.4 | 0.06 | 0.4 | 0.05 | 26.8 | 1.16 | 1.37 | 1.37 | 0.93 |
0605 | 1.3 | 3.9 | 0.22 | 0.9 | 0.2 | 0.05 | 0.1* | 0.1* | 0.3 | 2* | 0.1* | 0.2 | 0.05* | 0.2 | 0.04* | 7.27 | 0.75 | 0.72 | 1.74 | 0.63 |
sample ID | Sc | As | Hf | Th | U | Zr | Cr | Co | Cd | Cu | Ni | Zn | Ag | Pb | V | Ba | Sr | Ga | Ge | Rb | Mo |
ppm | |||||||||||||||||||||
0716 | 1* | 5 | 0.2* | 0.3 | 0.1* | 12 | 20* | 1* | 0.5 | 5 | 1* | 1* | 0.3* | 5* | 6 | 31 | 5 | 1* | 4 | 3 | 2* |
0718 | 1* | 5 | 0.2* | 0.1* | 0.3 | 4 | 20* | 1* | 0.6 | 4 | 3 | 2 | 0.9 | 5* | 32 | 15 | 12 | 1* | 1* | 2* | 2* |
0719 | 1* | 5 | 0.2* | 0.1* | 0.4 | 9 | 20* | 1* | 0.5 | 9 | 3 | 1* | 0.6 | 5* | 28 | 20 | 10 | 1* | 2 | 2* | 2* |
0720 | 1* | 5 | 0.2* | 0.1* | 0.1* | 5 | 20* | 1* | 0.6 | 7 | 4 | 49 | 0.5 | 14 | 16 | 15 | 12 | 1* | 1* | 2* | 2* |
0721 | 1* | 43 | 0.2* | 0.1* | 0.4 | 4 | 20* | 9 | 0.5 | 11 | 5 | 10 | 0.5 | 6 | 11 | 24 | 8 | 1* | 2 | 2* | 2* |
0722 | 1* | 5 | 0.2* | 0.1* | 0.3 | 4 | 20* | 1* | 0.5 | 8 | 3 | 3 | 0.3* | 5* | 5 | 114 | 11 | 1* | 1* | 2* | 2* |
0723 | 1* | 5 | 0.2* | 0.1* | 0.5 | 4 | 20* | 3 | 1.7 | 6 | 7 | 4 | 0.7 | 7 | 6 | 21 | 17 | 1* | 1* | 2* | 2* |
0301 | 19 | 5 | 4.4 | 9.8 | 2.4 | 169 | 150 | 8 | 0.7 | 127 | 29 | 18 | 0.3* | 8 | 142 | 466 | 33 | 23 | 4 | 190 | 2* |
0302 | 23 | 6 | 5.3 | 7.2 | 3.3 | 175 | 70 | 28 | 1.1 | 208 | 64 | 19 | 0.5 | 10 | 340 | 1620 | 18 | 17 | 3 | 11 | 27 |
0303 | 1* | 14 | 0.8 | 2.7 | 1.7 | 33 | 40 | 6 | 0.5 | 3 | 1* | 1* | 0.3* | 5* | 56 | 64 | 11 | 13 | 3 | 18 | 2* |
0304 | 15 | 5 | 3 | 7.8 | 3.1 | 124 | 70 | 18 | 1.3 | 22 | 44 | 23 | 0.4 | 5* | 131 | 333 | 20 | 23 | 4 | 124 | 4 |
0305 | 2 | 16 | 0.2* | 0.3 | 3 | 4 | 20* | 4 | 1.6 | 6 | 15 | 6 | 1.3 | 13 | 72 | 19 | 65 | 4 | 1* | 2* | 2* |
0306 | 1* | 9 | 0.2* | 0.1* | 2.3 | 4 | 20* | 4 | 2.1 | 11 | 13 | 8 | 1.9 | 12 | 76 | 15 | 25 | 3 | 1* | 2* | 2* |
0307 | 1* | 5 | 0.2* | 0.1* | 0.2 | 4 | 20* | 2 | 1.6 | 3 | 2 | 4 | 0.7 | 8 | 5 | 10 | 16 | 1* | 1* | 2* | 2* |
0308 | 1* | 5 | 0.2* | 0.1* | 0.2 | 4 | 20* | 2 | 1.4 | 6 | 5 | 4 | 0.7 | 5* | 5 | 22 | 14 | 1* | 2 | 2* | 2* |
0309 | 2 | 5 | 0.2* | 0.2 | 0.9 | 4 | 20* | 4 | 2.2 | 5 | 9 | 6 | 1.5 | 12 | 28 | 11 | 25 | 3 | 1* | 2* | 2* |
0310 | 1* | 5 | 0.2* | 0.1* | 0.1* | 4 | 20* | 2 | 1.3 | 5 | 3 | 4 | 0.5 | 6 | 5 | 13 | 17 | 1* | 2 | 2* | 2* |
0311 | 1* | 5 | 0.2* | 0.1* | 0.1* | 4 | 20* | 3 | 0.8 | 9 | 4 | 3 | 0.3* | 5* | 5 | 14 | 5 | 1* | 1* | 2* | 2* |
0701 | 3 | 5 | 0.6 | 1.9 | 0.2 | 37 | 20* | 6 | 1.8 | 1* | 9 | 7 | 0.9 | 7 | 24 | 21 | 11 | 5 | 2 | 2* | 2* |
0702 | 4 | 5 | 1.6 | 3.6 | 0.5 | 74 | 40 | 9 | 1.2 | 9 | 15 | 52 | 0.3* | 5* | 47 | 25 | 7 | 12 | 6 | 5 | 2* |
0703 | 3 | 5 | 0.8 | 2.4 | 0.5 | 40 | 20* | 6 | 1.7 | 6 | 9 | 10 | 0.4 | 5* | 24 | 18 | 13 | 7 | 3 | 2* | 2* |
0704 | 1* | 5 | 0.4 | 1 | 0.3 | 19 | 20* | 6 | 1.8 | 4 | 8 | 6 | 0.8 | 9 | 10 | 14 | 14 | 4 | 2 | 2* | 2* |
0705 | 4 | 5 | 1 | 2.8 | 0.4 | 53 | 20* | 12 | 1.8 | 3 | 11 | 10 | 0.5 | 8 | 29 | 23 | 9 | 8 | 3 | 2* | 2* |
0706 | 3 | 15 | 0.5 | 1.4 | 0.8 | 20 | 20* | 21 | 1.1 | 17 | 13 | 8 | 0.5 | 12 | 25 | 18 | 45 | 4 | 2 | 2* | 2* |
0707 | 4 | 5 | 1.4 | 3.3 | 0.6 | 59 | 40 | 6 | 1.4 | 4 | 11 | 10 | 0.4 | 5 | 29 | 29 | 21 | 7 | 4 | 2* | 2* |
0708 | 6 | 5 | 0.4 | 1.9 | 0.4 | 24 | 20* | 7 | 1.9 | 5 | 12 | 10 | 0.6 | 6 | 27 | 11 | 14 | 6 | 3 | 2* | 2* |
0709 | 4 | 5 | 1.3 | 4.1 | 0.9 | 60 | 30 | 10 | 1.6 | 18 | 15 | 13 | 0.4 | 5* | 34 | 42 | 9 | 9 | 3 | 5 | 2* |
0710 | 3 | 5 | 2.1 | 5.3 | 1 | 100 | 40 | 9 | 1 | 21 | 19 | 14 | 0.3* | 5* | 42 | 131 | 13 | 12 | 5 | 22 | 2* |
0711 | 6 | 5 | 0.8 | 1.9 | 0.8 | 36 | 20* | 1* | 1.1 | 4 | 10 | 7 | 0.6 | 7 | 31 | 37 | 51 | 6 | 1* | 6 | 2* |
0712 | 3 | 5 | 2 | 3.5 | 1.4 | 78 | 50 | 6 | 0.9 | 13 | 17 | 13 | 0.3* | 5* | 54 | 167 | 14 | 13 | 5 | 35 | 2* |
0713 | 3 | 22 | 0.9 | 1.5 | 1.7 | 37 | 20* | 19 | 1.3 | 9 | 15 | 5 | 0.8 | 11 | 18 | 19 | 24 | 5 | 2 | 2* | 2* |
0714 | 14 | 5 | 0.7 | 2.4 | 1.2 | 33 | 20* | 11 | 1.7 | 16 | 21 | 11 | 0.7 | 7 | 84 | 74 | 16 | 11 | 4 | 14 | 2* |
0715 | 11 | 5 | 3.3 | 9.7 | 1.7 | 133 | 80 | 6 | 0.7 | 21 | 28 | 18 | 0.3* | 5* | 87 | 493 | 28 | 13 | 4 | 122 | 2* |
0724 | 1 | 16 | 0.2* | 0.2 | 1.3 | 4 | 20* | 1* | 1.2 | 5 | 8 | 2 | 0.7 | 6 | 21 | 15 | 42 | 1* | 1* | 2* | 2* |
0725 | 4 | 38 | 0.8 | 2 | 0.8 | 35 | 20* | 20 | 1 | 14 | 49 | 17 | 0.5 | 16 | 45 | 18 | 13 | 13 | 4 | 2* | 2* |
0726 | 4 | 5 | 0.4 | 1 | 1.2 | 23 | 20* | 1* | 0.5 | 9 | 10 | 5 | 0.3* | 5* | 46 | 62 | 56 | 3 | 1* | 11 | 2* |
0729 | 1* | 14 | 0.2* | 0.1* | 0.6 | 4 | 20* | 3 | 0.5 | 8 | 4 | 21 | 0.3* | 5* | 6 | 16 | 6 | 1* | 2 | 2* | 2* |
0730 | 1* | 5 | 0.3 | 0.1* | 0.3 | 4 | 20* | 1* | 0.5 | 6 | 2 | 1* | 0.3* | 5* | 5 | 8 | 3 | 1* | 2 | 2* | 2* |
0601 | 4 | 12 | 0.3 | 0.8 | 1.5 | 11 | 20* | 4 | 2.1 | 7 | 18 | 19 | 0.5 | 15 | 60 | 35 | 23 | 5 | 2 | 3 | 2* |
0602 | 1* | 5 | 0.2* | 0.2 | 0.1* | 5 | 20* | 3 | 0.5 | 13 | 3 | 47 | 0.3* | 9 | 5 | 76 | 14 | 1* | 6 | 3 | 2* |
0603 | 1* | 9 | 0.2* | 0.6 | 0.3 | 11 | 20* | 4 | 1.9 | 9 | 9 | 24 | 0.4 | 12 | 13 | 29 | 28 | 1* | 2 | 2* | 2* |
0604 | 1* | 14 | 2.7 | 0.3 | 0.3 | 99 | 20* | 3 | 1.3 | 11 | 7 | 27 | 0.3* | 6 | 5 | 15 | 12 | 2 | 5 | 2* | 2* |
0605 | 1* | 2 | 0.2* | 0.1* | 0.1* | 4 | 20* | 3 | 1.1 | 7 | 4 | 2 | 0.3* | 5* | 9 | 5 | 2 | 1* | 3 | 2* | 2* |
* : below detection limit
Back to article | Download in Excel
Figure S-3 Correlation diagrams of geochemical data from the examined samples. Data are sorted based on lithology: blue rhombs = carbonate, red circle = chert, black triangle = shale, and square = BIF (yellow: chert/carbonate, black: shale containing iron layer). (a) Correlation diagram of total organic carbon content (TOC) versus pyrite sulphur content (Spyr). A positive correlation exists, except for two samples that contain higher amounts of sulphur (samples #0721 and #0725). (b) Correlation diagram of TOC versus d13C values for organic matter. No correlation is observed. Correlation diagrams of (c) kerogen C/N ratios vs. d15N values and (d) d13C vs. d15N values.

Figure S-4 Correlation diagram of N/C and H/C ratios of kerogens. Data are sorted by lithology; legends are the same as Figure S-3. Grey squares are data from Mesoproterozoic to Palaeoproterozoic kerogen samples cited from Schopf and Klein (1992).
Table S-2 Summary of the stepwise combustion analyses performed on kerogen (sample IDs #0701, #0703, #0705, #0706, #0709, #0711, #0712, #0713, #0714, #0721, and #0723).
Temp | C | H | N | C/N | d15N | ± |
°C | ppm | (atomic) | ‰ | |||
0701 (0.047 mg) | ||||||
500 | 82705 | 69 | 81 | 1193 | -5.6 | 0.7 |
525 | 85022 | 121 | 84 | 1180 | 5.1 | 0.8 |
550 | 125467 | 221 | 72 | 2040 | 4.9 | 0.9 |
575 | 164260 | 212 | 389 | 493 | 6.6 | 0.7 |
1100 | 348763 | 436 | 3547 | 115 | 5.8 | 0.8 |
total | 806217 | 1059 | 4172 | |||
average | 225 | 5.6 | 0.8 | |||
0703 (0.226 mg) | ||||||
475 | 79345 | 2544 | 54 | 1724 | 0.5 | 1.8 |
500 | 92155 | 1235 | 82 | 1312 | 3.8 | 0.4 |
525 | 151538 | 1392 | 215 | 822 | 5.9 | 0.6 |
550 | 188591 | 1150 | 466 | 472 | 6.6 | 0.4 |
575 | 218032 | 939 | 823 | 309 | 7.0 | 0.7 |
1100 | 135681 | 576 | 1141 | 139 | 7.9 | 0.5 |
total | 865342 | 7835 | 2780 | |||
average | 363 | 7.1 | 0.5 | |||
0705 (0.286 mg) | ||||||
450 | pre-combustion | |||||
475 | 115150 | 182 | 61 | 2200 | 5.9 | 1.2 |
500 | 141665 | 245 | lost | |||
525 | 46160 | 359 | 200 | 269 | 6.9 | 1.0 |
550 | 10286 | 559 | 388 | 31 | 5.8 | 1.4 |
575 | 5026 | 844 | 1445 | 4 | 8.4 | 1.7 |
1100 | 34316 | 2720 | 2177 | 18 | 7.3 | 1.6 |
total | 352604 | 4908 | - | |||
average | 902 | 5.4 | 1.4 | |||
0706 (0.555 mg) | ||||||
475 | 40784 | 22 | 59 | 803 | 0.1 | 0.8 |
500 | 91937 | 655 | 217 | 495 | 5.0 | 0.4 |
525 | 110533 | 829 | 401 | 322 | 7.6 | 0.4 |
550 | 89845 | 573 | 356 | 295 | 7.9 | 0.4 |
575 | 25852 | 147 | 37 | 804 | 6.4 | 0.5 |
1100 | 22105 | 474 | 42 | 617 | 8.4 | 0.5 |
total | 381056 | 2701 | 1112 | |||
average | 400 | 6.9 | 0.4 | |||
0709 (0.067 mg) | ||||||
500 | 180948 | 197 | 480 | 440 | 2.9 | 0.9 |
525 | 172407 | 137 | 1058 | 190 | 7.4 | 0.6 |
550 | 89034 | 50 | 409 | 254 | 8.7 | 0.4 |
575 | 30518 | 54 | 42 | 850 | 5.6 | 0.5 |
1100 | 53034 | 154 | 59 | 1045 | 4.4 | 0.7 |
total | 525940 | 593 | 2049 | |||
average | 299 | 7.3 | 0.6 | |||
0711 (0.412 mg) | ||||||
450 | pre-combustion | |||||
475 | 60541 | 93 | 126 | 803 | 7.2 | 0.5 |
500 | 99552 | 477 | 317 | 495 | 7.8 | 0.3 |
525 | 122193 | 545 | 756 | 322 | 6.7 | 0.4 |
550 | 125328 | 578 | 1018 | 295 | 8.8 | 0.3 |
575 | 13805 | 265 | 75 | 804 | 8.1 | 0.4 |
1100 | lost | |||||
total | 421419 | 1958 | 2291 | |||
average | 215 | 8.0 | 0.4 | |||
0712 (0.072 mg) | ||||||
500 | 192440 | 1022 | 544 | 412 | 4.6 | 0.8 |
525 | 263813 | 374 | 2211 | 139 | 6.2 | 0.7 |
550 | 139411 | 250 | 1212 | 134 | 8.7 | 0.5 |
575 | 15570 | 70 | 54 | 339 | 7.7 | 0.7 |
1100 | 40299 | 229 | 21 | 2254 | 4.7 | 1.2 |
total | 651534 | 1944 | 4041 | |||
average | 188 | 7.2 | 0.3 | |||
0713 (0.065 mg) | ||||||
500 | 157778 | 365 | 280 | 656 | 5.0 | 0.7 |
525 | 116425 | 210 | 196 | 691 | 7.6 | 0.5 |
550 | 24157 | 42 | 25 | 1108 | 6.6 | 0.6 |
575 | 6824 | 17 | 8 | 944 | 5.9 | 1.3 |
1100 | 29652 | 98 | 24 | 1457 | 2.7 | 1.3 |
total | 334837 | 731 | 535 | |||
average | 731 | 6.4 | 0.6 | |||
0714 (0.611 mg) | ||||||
450 | pre-combustion | |||||
475 | 96101 | 762 | 115 | 973 | 2.9 | 0.7 |
500 | 85257 | 837 | 122 | 814 | 4.4 | 0.8 |
525 | 103927 | 626 | 145 | 834 | 4.9 | 0.8 |
550 | 135435 | 770 | 231 | 683 | 4.5 | 1.0 |
575 | 117687 | 504 | 258 | 533 | 6.1 | 1.1 |
1100 | 24930 | 144 | 202 | 144 | 5.8 | 0.8 |
total | 563338 | 3644 | 1074 | |||
average | 612 | 4.8 | 0.9 | |||
0721 (0.153 mg) | ||||||
500 | 54442 | 17 | 33 | 1923 | 2.1 | 0.6 |
525 | 57008 | 15 | 62 | 1079 | 5.5 | 0.6 |
550 | 29440 | 6 | 19 | 1785 | 5.6 | 0.7 |
575 | 5187 | 6 | 3 | 1856 | lost | |
1100 | 32579 | 24 | 15 | 2568 | 6.8 | 0.8 |
total | 178657 | 67 | 132 | |||
average | 1579 | 4.7 | 0.6 | |||
0723 (0.412 mg) | ||||||
450 | pre-combustion | |||||
475 | 28654 | 68 | 94 | 357 | 4.6 | 0.3 |
500 | 29798 | 76 | 138 | 252 | 5.2 | 0.5 |
525 | 34056 | 68 | 246 | 161 | 6.6 | 0.5 |
550 | 38036 | 77 | 360 | 123 | 5.1 | 0.3 |
575 | 38036 | 43 | 402 | 110 | 5.4 | 0.3 |
1100 | 45206 | 166 | 30 | 1739 | 6.6 | 0.4 |
total | 213785 | 498 | 1271 | |||
average | 196 | 5.4 | 0.4 |

Figure S-5 An alternative version of Figure 1. The lower- and the higher-temperature fractions are divided depending on the shift of d15N values at approximately 575 °C. The nitrogen concentrations (white bars) and d15N values (black squares) are shown.
Table S-3 Summary of the d15NLT and d15NHT values of the 13 samples analysed with the stepwise combustion method. ‘d15N (‰): divided by temperature’ refers to the lower- and higher-temperature fractions divided based on the temperature: lower temperature = 500–575 °C, higher temperature = higher than 575 to 1100 °C. ‘d15N (‰): divided by d15N values’ means the data are divided and calculated into two fractions according to the shift in d15N values (refer to Fig. S-5). The average d15N values were calculated based on the weighted average of the released amount of nitrogen.
Sample ID | Litho facies | d15N (‰): divided by temperature | d15N (‰): divided by d15N values | ||||||||
d15NLT | ± | d15NHT | ± | temperature range | d15NLT | ± | temperature range | d15NHT | ± | ||
0701 | Carbonate sandstone | 4.6 | 0.7 | 5.8 | 0.8 | 500-550 °C | 5.0 | 0.8 | 575-1100 °C | 5.9 | 0.8 |
0703 | Carbonate sandstone | 6.5 | 0.6 | 7.9 | 0.5 | 500-575 °C | 6.5 | 0.6 | 1100 °C | 7.9 | 0.5 |
0704 | Carbonate sandstone | 5.9 | 0.8 | 5.5 | 0.8 | 500-575 °C | 5.9 | 0.8 | 600-650 °C | 5.2 | 0.8 |
0705 | Carbonate sandstone | 7.8 | 1.6 | 7.3 | 1.6 | 500-575 °C | 7.8 | 1.6 | 1100 °C | 7.3 | 1.6 |
0706 | Carbonate sandstone | 7.1 | 0.4 | 8.4 | 0.5 | 500-550 °C | 7.1 | 0.4 | 575-1100 °C | 7.4 | 0.5 |
0708 | Carbonate sandstone | 4.3 | 1.0 | 7.1 | 0.8 | 500-600 °C | 5.0 | 1.0 | 625-1100 °C | 7.3 | 0.8 |
0709 | Carbonate sandstone | 6.5 | 0.6 | 4.4 | 0.7 | 500-575 °C | 6.5 | 0.6 | 1100 °C | 4.9 | 0.7 |
0711 | Carbonate sandstone | 7.9 | 0.4 | - | - | 500-550 °C | 7.9 | 0.4 | - | - | - |
0712 | Carbonate sandstone | 6.8 | 0.7 | 4.7 | 1.2 | 500-550 °C | 6.8 | 0.7 | 575-1100 °C | 6.9 | 0.8 |
0713 | Shale (phosphorous) | 6.1 | 0.6 | 2.7 | 1.3 | 500-525 °C | 6.1 | 0.6 | 550-1100 °C | 4.9 | 1.0 |
0714 | Black shale | 5.1 | 1.0 | 5.8 | 0.8 | 500-550 °C | 4.6 | 0.9 | 575-1100 °C | 6.0 | 0.9 |
0721 | Taconite | 4.4 | 0.6 | 6.8 | 0.8 | 500-550 °C | 4.4 | 0.6 | 1100 °C | 6.8 | 0.8 |
0723 | Carbonate BIF | 5.5 | 0.4 | 6.6 | 0.4 | 500-575 °C | 5.5 | 0.4 | 1100 °C | 6.6 | 0.4 |
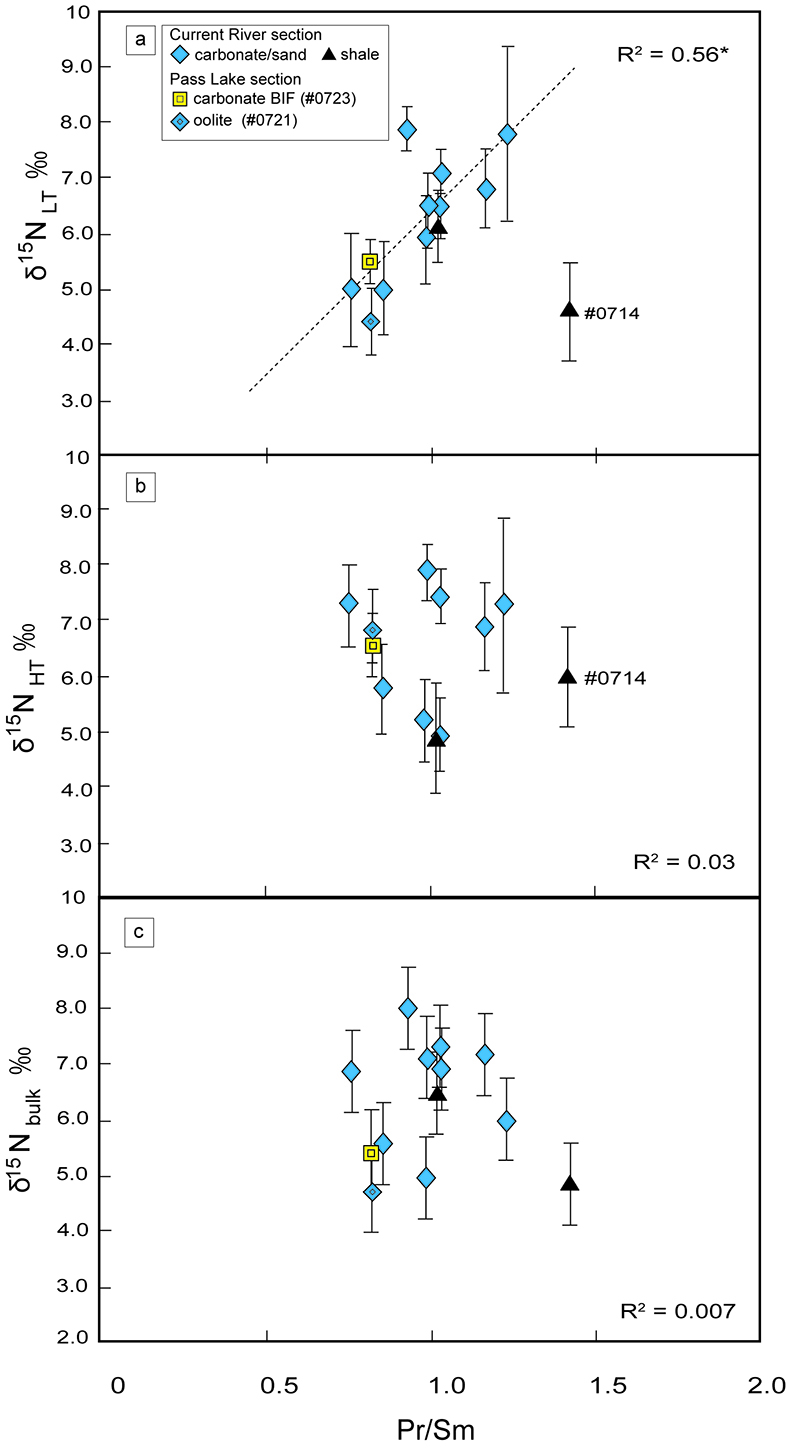
Figure S-6 The correlations between the (a) d15NLT values, and (b) the d15NHT values, and the Pr/Sm ratios. Both d15NLT and d15NHT are divided based on the temperatures of the shift of the d15N values that were observed on each sample. The results of calculations are summarised in the Table S-3. The same trends as in Figure 2 were observed: a positive correlation is observed between the Pr/Sm ratios and d15NLT values (R2 = 0.59, excluding #0714), whereas no correlation is observed between the Pr/Sm ratios and d15NHT values. (c) There is no correlation between the d15Nbulk values and the Pr/Sm ratios.
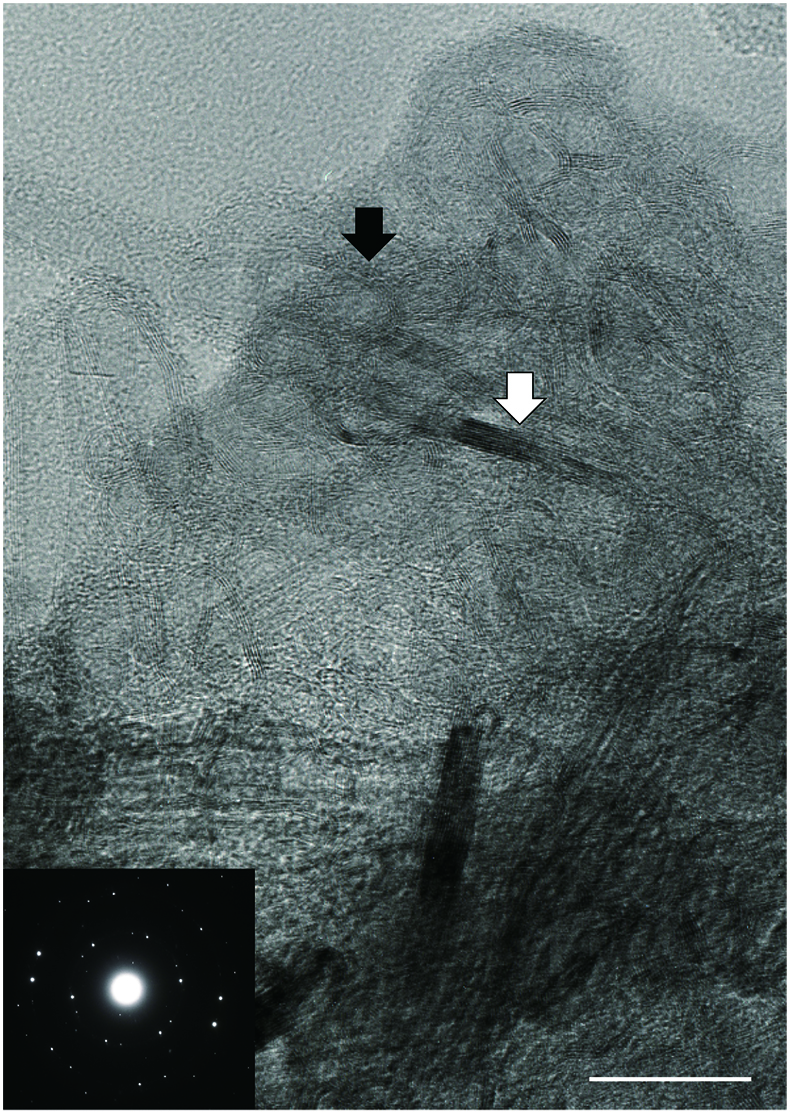
Figure S-7 Transmitted electron microscopic image of the kerogen extracted from sample #0708. There are at least two types of carbon structures: a highly graphitised portion (white arrow) and folded structures (black arrow). This difference in structure might be responsible for the differences in the nitrogen isotopes. The scale bar is 200 nanometres. Insert: Selected area electron diffraction pattern of the highly graphitised area.
Table S-4 Summary of REE concentrations of the examined samples normalised by the PAAS values.
sample ID | La | Ce | Pr | Nd | Sm | Eu | Gd | Tb | Dy | Y | Ho | Er | Tm | Yb | Lu |
(PAAS normalised) | |||||||||||||||
0716 | 0.022 | 0.036 | 0.018 | 0.016 | 0.015 | 0.041 | 0.017* | 0.112* | 0.019* | 0.073* | 0.095* | 0.033* | 0.111* | 0.033* | 0.091* |
0718 | 0.191 | 0.207 | 0.212 | 0.249 | 0.349 | 0.856 | 0.447 | 0.449 | 0.469 | 0.659 | 0.475 | 0.390 | 0.310 | 0.232 | 0.182 |
0719 | 0.128 | 0.130 | 0.125 | 0.137 | 0.189 | 0.453 | 0.248 | 0.224 | 0.225 | 0.256 | 0.190 | 0.195 | 0.177 | 0.133 | 0.114 |
0720 | 0.114 | 0.113 | 0.117 | 0.126 | 0.174 | 0.420 | 0.215 | 0.224 | 0.282 | 0.330 | 0.285 | 0.293 | 0.266 | 0.199 | 0.182 |
0721 | 0.070 | 0.069 | 0.084 | 0.072 | 0.102 | 0.255 | 0.149 | 0.224 | 0.188 | 0.256 | 0.190 | 0.228 | 0.222 | 0.166 | 0.160 |
0722 | 0.043 | 0.046 | 0.038 | 0.040 | 0.044 | 0.115 | 0.066 | 0.112* | 0.056 | 0.073* | 0.095* | 0.065 | 0.111* | 0.033* | 0.091* |
0723 | 0.110 | 0.135 | 0.107 | 0.110 | 0.131 | 0.230 | 0.182 | 0.224 | 0.169 | 0.256 | 0.190 | 0.163 | 0.155 | 0.100 | 0.091* |
0301 | 1.066 | 0.914 | 0.639 | 0.461 | 0.363 | 0.494 | 0.331 | 0.449 | 0.469 | 0.586 | 0.475 | 0.553 | 0.599 | 0.631 | 0.616 |
0302 | 0.379 | 0.577 | 0.637 | 0.841 | 1.293 | 1.951 | 1.390 | 1.346 | 1.221 | 1.208 | 1.235 | 1.171 | 1.220 | 1.162 | 1.140 |
0303 | 0.272 | 0.301 | 0.275 | 0.300 | 0.363 | 0.551 | 0.381 | 0.337 | 0.338 | 0.403 | 0.285 | 0.325 | 0.310 | 0.299 | 0.296 |
0304 | 0.597 | 0.484 | 0.332 | 0.263 | 0.232 | 0.321 | 0.232 | 0.337 | 0.376 | 0.513 | 0.380 | 0.455 | 0.510 | 0.498 | 0.502 |
0305 | 0.489 | 0.708 | 0.831 | 0.981 | 1.206 | 1.942 | 1.340 | 1.234 | 1.070 | 1.318 | 0.950 | 0.813 | 0.643 | 0.498 | 0.456 |
0306 | 0.177 | 0.195 | 0.160 | 0.163 | 0.203 | 0.329 | 0.248 | 0.337 | 0.300 | 0.403 | 0.285 | 0.293 | 0.288 | 0.232 | 0.228 |
0307 | 0.074 | 0.087 | 0.068 | 0.070 | 0.087 | 0.148 | 0.099 | 0.112* | 0.094 | 0.183 | 0.095* | 0.098 | 0.111* | 0.033* | 0.091* |
0308 | 0.056 | 0.065 | 0.048 | 0.046 | 0.058 | 0.091 | 0.066 | 0.112* | 0.075 | 0.110 | 0.095* | 0.098 | 0.111* | 0.100 | 0.091* |
0309 | 0.236 | 0.275 | 0.229 | 0.230 | 0.276 | 0.379 | 0.314 | 0.337 | 0.376 | 0.549 | 0.380 | 0.390 | 0.355 | 0.332 | 0.319 |
0310 | 0.052 | 0.063 | 0.048 | 0.048 | 0.058 | 0.099 | 0.066 | 0.112* | 0.056 | 0.110 | 0.095* | 0.065 | 0.111* | 0.033* | 0.091* |
0311 | 0.022 | 0.028 | 0.020 | 0.019 | 0.029 | 0.041 | 0.017* | 0.112* | 0.019* | 0.073* | 0.095* | 0.033* | 0.111* | 0.033* | 0.091* |
0701 | 0.065 | 0.091 | 0.075 | 0.078 | 0.087 | 0.123 | 0.083 | 0.112* | 0.075 | 0.110 | 0.095* | 0.098 | 0.111* | 0.066 | 0.091* |
0702 | 0.339 | 0.315 | 0.163 | 0.110 | 0.087 | 0.107 | 0.050 | 0.112* | 0.075 | 0.110 | 0.095* | 0.098 | 0.111* | 0.100 | 0.114 |
0703 | 0.076 | 0.096 | 0.072 | 0.064 | 0.073 | 0.107 | 0.066 | 0.112* | 0.075 | 0.110 | 0.095* | 0.098 | 0.111* | 0.100 | 0.091* |
0704 | 0.061 | 0.074 | 0.057 | 0.051 | 0.058 | 0.091 | 0.050 | 0.112* | 0.056 | 0.073* | 0.095* | 0.065 | 0.111* | 0.033* | 0.091* |
0705 | 0.182 | 0.202 | 0.143 | 0.123 | 0.116 | 0.165 | 0.116 | 0.112* | 0.075 | 0.110 | 0.095* | 0.065 | 0.111* | 0.066 | 0.091* |
0706 | 0.175 | 0.187 | 0.150 | 0.137 | 0.145 | 0.206 | 0.132 | 0.112* | 0.113 | 0.146 | 0.095* | 0.098 | 0.111* | 0.100 | 0.091* |
0707 | 0.076 | 0.088 | 0.053 | 0.054 | 0.058 | 0.091 | 0.066 | 0.112* | 0.094 | 0.146 | 0.095* | 0.098 | 0.111* | 0.100 | 0.114 |
0708 | 0.092 | 0.120 | 0.110 | 0.118 | 0.145 | 0.239 | 0.182 | 0.112* | 0.131 | 0.183 | 0.095* | 0.098 | 0.111* | 0.066 | 0.091* |
0709 | 0.132 | 0.161 | 0.135 | 0.123 | 0.131 | 0.165 | 0.116 | 0.112* | 0.075 | 0.073* | 0.095* | 0.098 | 0.111* | 0.066 | 0.091* |
0710 | 0.153 | 0.159 | 0.120 | 0.102 | 0.102 | 0.156 | 0.083 | 0.112* | 0.094 | 0.110 | 0.095* | 0.228 | 0.111* | 0.100 | 0.114 |
0711 | 0.227 | 0.240 | 0.189 | 0.177 | 0.203 | 0.346 | 0.215 | 0.224 | 0.244 | 0.439 | 0.285 | 0.228 | 0.200 | 0.166 | 0.160 |
0712 | 0.126 | 0.131 | 0.101 | 0.086 | 0.087 | 0.156 | 0.083 | 0.112* | 0.094 | 0.146 | 0.095* | 0.098 | 0.111* | 0.100 | 0.091* |
0713 | 0.390 | 0.432 | 0.311 | 0.281 | 0.305 | 0.535 | 0.331 | 0.337 | 0.300 | 0.476 | 0.285 | 0.260 | 0.244 | 0.199 | 0.182 |
0714 | 0.408 | 0.344 | 0.247 | 0.196 | 0.174 | 0.189 | 0.132 | 0.112* | 0.075 | 0.073* | 0.095* | 0.098 | 0.111* | 0.066 | 0.091* |
0715 | 0.285 | 0.256 | 0.210 | 0.180 | 0.174 | 0.247 | 0.149 | 0.224 | 0.169 | 0.256 | 0.190 | 0.228 | 0.266 | 0.266 | 0.296 |
0724 | 0.182 | 0.201 | 0.149 | 0.139 | 0.160 | 0.280 | 0.232 | 0.224 | 0.263 | 0.439 | 0.285 | 0.260 | 0.222 | 0.199 | 0.160 |
0725 | 0.018 | 0.027 | 0.028 | 0.035 | 0.044 | 0.082 | 0.066 | 0.112* | 0.113 | 0.073* | 0.095* | 0.130 | 0.133 | 0.100 | 0.114 |
0726 | 0.085 | 0.091 | 0.083 | 0.088 | 0.116 | 0.214 | 0.149 | 0.224 | 0.188 | 0.256 | 0.190 | 0.195 | 0.177 | 0.133 | 0.137 |
0729 | 0.009 | 0.007* | 0.007* | 0.013 | 0.029 | 0.041 | 0.033 | 0.112* | 0.038 | 0.073* | 0.095* | 0.033* | 0.111* | 0.066 | 0.091* |
0730 | 0.007* | 0.007* | 0.009 | 0.019 | 0.044 | 0.066 | 0.050 | 0.112* | 0.038 | 0.073* | 0.095* | 0.033* | 0.111* | 0.066 | 0.091* |
0601 | 0.175 | 0.198 | 0.173 | 0.169 | 0.189 | 0.337 | 0.248 | 0.224 | 0.263 | 0.366 | 0.285 | 0.260 | 0.222 | 0.199 | 0.205 |
0602 | 0.007* | 0.007* | 0.006 | 0.005* | 0.015 | 0.041 | 0.017* | 0.112* | 0.019* | 0.073* | 0.095* | 0.033* | 0.111* | 0.033* | 0.091* |
0603 | 0.254 | 0.342 | 0.267 | 0.276 | 0.320 | 0.370 | 0.414 | 0.112* | 0.131 | 0.549 | 0.095* | 0.130 | 0.133 | 0.133 | 0.274 |
0604 | 0.090 | 0.131 | 0.101 | 0.086 | 0.087 | 0.132 | 0.099 | 0.112* | 0.131 | 0.146 | 0.095* | 0.130 | 0.133 | 0.133 | 0.114 |
0605 | 0.029 | 0.044 | 0.022 | 0.024 | 0.029 | 0.041 | 0.017* | 0.112* | 0.019* | 0.073* | 0.095* | 0.065 | 0.111* | 0.066 | 0.091* |
PAAS values | La | Ce | Pr | Nd | Sm | Eu | Gd | Tb | Dy | Y | Ho | Er | Tm | Yb | Lu |
44.56 | 88.25 | 10.15 | 37.32 | 6.884 | 1.215 | 6.043 | 0.8914 | 5.325 | 27.31 | 1.053 | 3.075 | 0.451 | 3.012 | 0.4386 |
* below detection limit
PAAS values were referred from Pourmand et al. (2012)
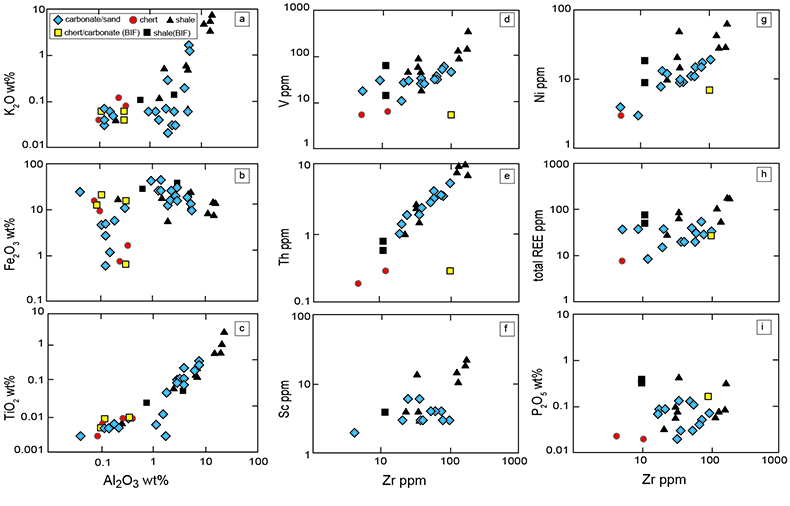
Figure S-8 Correlation diagrams of Al2O3 versus K2O, Fe2O3, and TiO2 in bulk rock and Zr versus V, Th, Sc, Ni, total amount of rare earth elements (total REEs), and P2O5 concentrations. K2O (a) and TiO2 (c) show positive correlations with Al2O3, whereas Fe2O3 (b) does not exhibit a correlation with Al2O3. V (d), Th (e), Sc (f), and Ni (g) have positive correlations with Zr. Total REEs and P2O5 have weak positive correlations. Data below the detection limit were omitted. Data are sorted by lithology; legends are the same as Figure S-3.

Figure S-9 Diagrams of the rare earth elements and Y in each surveyed section: (a) Pass Lake section, (b) Blende Lake North section, (c) Current River section, (d) Terry Fox section, and (e) Kakabeka Falls section.
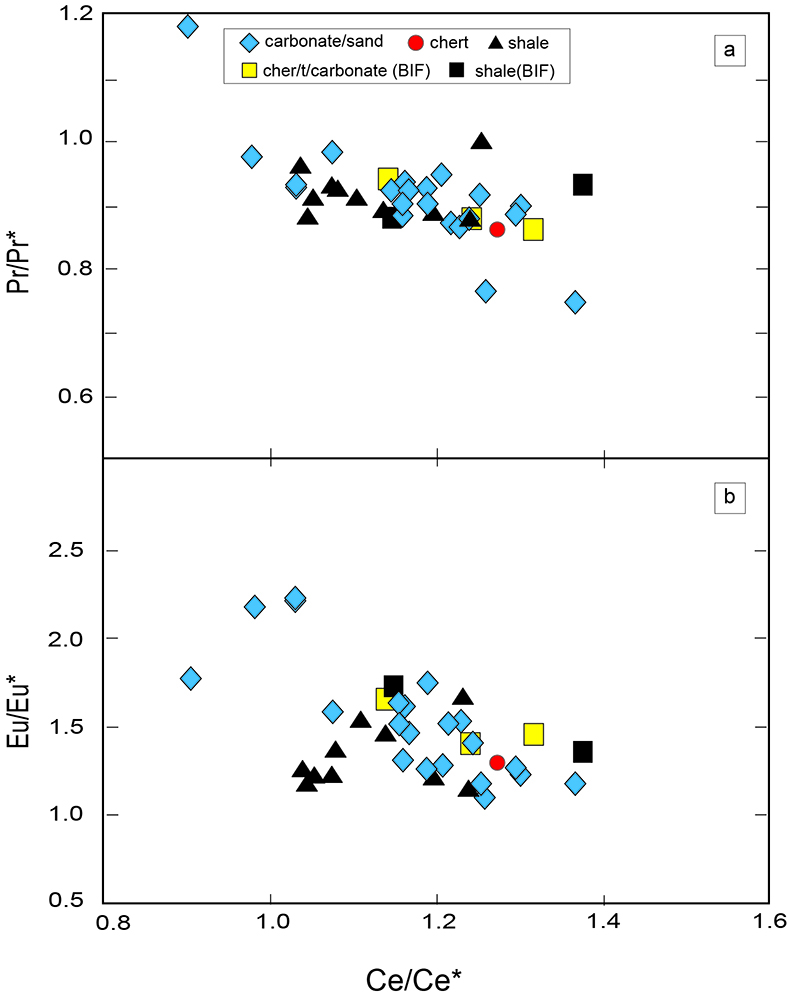
Figure S-10 Correlation diagrams of (a) Pr/Pr* and Ce/Ce* ratios and (b) Ce/Ce* and Eu/Eu* ratios. Pr/Pr* is defined as Pr/0.5(Ce+Nd), and Ce/Ce* is defined as Ce/0.5(La+Pr). A negative correlation exists between them. Data are sorted by lithology; legends are the same as Figure S-3.
Table S-5 Correlation coefficient values of the Pr/Sm ratios against the major and trace elements concentrations. Elements which have positive correlations with the Pr/Sm ratios are displayed in bold.
Major elements | Trace elements | REEs | d15N values | ||||
SiO2 | 0.23 | Sc | 0.67 | La | 0.62 | d15NLT | 0.56 |
Al2O3 | 0.68 | As | -0.23 | Ce | 0.49 | d15NHT | 0.03 |
Fe2O3 | -0.10 | Hf | 0.74 | Pr | 0.25 | d15Nbulk | <0.01 |
MnO | -0.43 | Ta | 0.67 | Nd | 0.04 | ||
MgO | -0.36 | Th | 0.69 | Sm | -0.12 | ||
CaO | -0.36 | U | 0.29 | Eu | -0.23 | ||
Na2O | -0.13 | Zr | 0.76 | Gd | -0.23 | ||
K2O | 0.56 | Cr | 0.64 | Tb | -0.15 | ||
TiO2 | 0.68 | Co | 0.37 | Dy | -0.15 | ||
P2O5 | -0.23 | Cd | 0.01 | Y | -0.16 | ||
Cu | 0.56 | Ho | -0.11 | ||||
Ni | 0.42 | Er | -0.01 | ||||
Zn | 0.48 | Tm | 0.11 | ||||
Ag | -0.36 | Yb | 0.23 | ||||
Pb | -0.27 | Lu | 0.27 | ||||
V | 0.53 | ||||||
Ba | 0.55 | ||||||
Sr | -0.10 | ||||||
Ga | 0.69 | ||||||
Ge | 0.71 | ||||||
Rb | 0.59 | ||||||
Mo | 0.23 |
The components which have positive correlation coefficiencies are displayed in bold.
Back to article | Download in Excel