The heterogeneous nature of Fe delivery from melting icebergs
Affiliations | Corresponding Author | Cite as | Funding informationKeywords: icebergs, iron, Fe, Svalbard, Arctic
-
Share this article
Article views:8,353Cumulative count of HTML views and PDF downloads.
- Download Citation
-
Rights & Permissions
Abstract
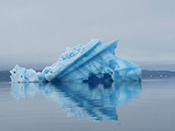
Figures and Tables
![]() Figure 1 Surface fjord sample locations in Kongsfjorden. |
![]() Figure 2 Median FeAsc (wt. %) with 25/75th (boxes) and 10/90th (whiskers) percentiles (outliers also shown) for iceberg embedded (n = 34) and iceberg surface (n = 20) sediment. |
![]() Figure 3 DFe and TdFe (both nM, plotted as log10, TdFe shown minus DFe) for 28 discrete iceberg samples showed no clear relationship. |
![]() Table 1 Comparing data for Kongsfjorden from this and prior work suggests a critical difference in both FeAsc (wt. %) and in the scaling of FeAsc to iceberg sediment load (g L-1 of ice melt). *The suggested 0.5 g L-1 sediment loading is used for data from Raiswell et al. (2016). **For our study, measured sediment loadings were used for each sample. As sediment-rich ice was specifically targeted, the calculated mean/median should be over-estimates. |
![]() Figure 4 FeAsc (µmol L-1 melted ice) increased with sediment load (g L-1 melted ice), but it is unclear if the relationship remains linear at high (>50 g L-1) loadings. |
Figure 1 | Figure 2 | Figure 3 | Table 1 | Figure 4 |
Supplementary Figures and Tables
![]() Table S-1 Analysis of Certified Reference Materials for Fe concentration (± standard deviation of at least 6 measurements). |
![]() Table S-2 FeAsc concentration reported for various sediment samples collected around Kongsfjorden. For iceberg embedded sediment with sediment loadings >0.1 g L-1, FeAsc is reported both as wt. % and per volume of meltwater. |
![]() Table S-3 Dissolved (<0.2 µm) Fe and total dissolvable Fe for 15 surface water samples collected in Kongsfjorden (July 2015). |
![]() Table S-4 Dissolved (<0.2 µm) Fe and total dissolvable Fe for 28 randomly collected Kongsfjorden iceberg samples (July 2015). |
Table S-1 | Table S-2 | Table S-3 | Table S-4 |
top
Introduction
Icebergs contain higher Fe concentrations than seawater, both in the dissolved (<0.2 µm) (Martin et al., 1990
Martin, J.H., Gordon, R.M., Fitzwater, S.E. (1990) Iron in Antarctic waters. Nature 345, 156–158.
; De Baar et al., 1995De Baar, H.J.W., De Jong, J.T.M., Bakker, D.C.E., Loscher, B.M., Veth, C., Bathmann, U., Smetacek, V. (1995) Importance of iron for plankton blooms and carbon dioxide drawdown in the Southern Ocean. Nature 373, 412–415.
; Loscher et al., 1997Loscher, B.M., De Baar, H.J.W., De Jong, J.T.M., Veth, C., Dehairs, F. (1997) The distribution of Fe in the Antarctic Circumpolar Current. Deep Sea Research Part II: Topical Studies in Oceanography 44, 143–187.
) and particulate (>0.2 µm) phases (Hart, 1934Hart, T.J. (1934) Discovery Reports. Discovery Reports 8, 1–268.
; Lin et al., 2011Lin, H., Rauschenberg, S., Hexel, C.R., Shaw, T.J., Twining, B.S. (2011) Free-drifting icebergs as sources of iron to the Weddell Sea. Deep Sea Research Part II: Topical Studies in Oceanography 58, 1392–1406.
; Shaw et al., 2011Shaw, T.J., Raiswell, R., Hexel, C.R., Vu, H.P., Moore, W.S., Dudgeon, R., Smith Jr., K.L. (2011) Input, composition, and potential impact of terrigenous material from free-drifting icebergs in the Weddell Sea. Deep Sea Research Part II: Topical Studies in Oceanography 58, 1376–1383.
). Icebergs should thus constitute a source of the micronutrient Fe to offshore polar waters (Raiswell et al., 2008Raiswell, R., Benning, L.G., Tranter, M., Tulaczyk, S. (2008) Bioavailable iron in the Southern Ocean: the significance of the iceberg conveyor belt. Geochemical Transactions 9, doi:10.1186/1467-4866-9-7.
). As the rate of iceberg calving in polar seas oscillates on glacial to inter-glacial timescales (Bond et al., 1992Bond, G., Heinrich, H., Broecker, W.S., Labeyrie, L., McManus, J.F., Andrews, J.T., Huon, S., Jantschik, R., Clasen, S., Simet, C., Tedesco, K., Klas, M., Bonani, G., Ivy, S. (1992) Evidence for massive discharges of icebergs into the North Atlantic ocean during the last glacial period, Nature 360, 245–249.
), and recent climate change has increased the discharge of calved ice volume from both the Antarctic (Paolo et al., 2015Paolo, F.S., Fricker, H.A., Padman, L. (2015) Volume loss from Antarctic ice shelves is accelerating. Science 348, 327–331.
) and Greenlandic (Bamber et al., 2012Bamber, J., van den Broeke, M., Ettema, J., Lenaerts, J., Rignot, E. (2012) Recent large increases in freshwater fluxes from Greenland into the North Atlantic. Geophysical Research Letters 39, L19501, doi:10.1029/2012gl052552.
) ice sheets, Fe delivery from icebergs may also change. Particularly in the Southern Ocean, where DFe deficiency extensively limits primary production (Martin et al., 1990Martin, J.H., Gordon, R.M., Fitzwater, S.E. (1990) Iron in Antarctic waters. Nature 345, 156–158.
, 1991Martin, J.H., Gordon, R.M., Fitzwater, S.E. (1991)The case for iron. Limnology and Oceanography 36, 1793–1802.
; Moore et al., 2013Moore, C.M., Mills, M.M., Arrigo, K.R., Berman-Frank, I., Bopp, L., Boyd, P.W., Galbraith, E.D., Geider, R.J., Guieu, C., Jaccard, S.L., Jickells, T.D., La Roche, J., Lenton, T.M., Mahowald, N.M., Maranon, E., Marinov, I., Moore, J.K., Nakatsuka, T., Oschlies, A., Saito, M.A., Thingstad, T.F., Tsuda, A., Ulloa, O. (2013) Processes and patterns of oceanic nutrient limitation. Nature Geoscience 6, 701–710.
), and icebergs cause chemical and biological enrichment of surrounding waters (Smith Jr. et al., 2007Smith Jr., K.L., Robison, B.H., Helly, J.J., Kaufmann, R.S., Ruhl, H.A., Shaw, T.J., Twining, B.S., Vernet, M. (2007) Free-drifting icebergs: Hot spots of chemical and biological enrichment in the Weddell Sea. Science 317, 478–482.
; Schwarz and Schodlok, 2009Schwarz, J.N., Schodlok, M.P. (2009) Impact of drifting icebergs on surface phytoplankton biomass in the Southern Ocean: Ocean colour remote sensing and in situ iceberg tracking. Deep Sea Research Part I: Oceanographic Research Papers 56, 1727–1741.
; Smith et al., 2011Smith, K.L., Sherman, A.D., Shaw, T.J., Murray, A.E., Vernet, M., Cefarelli, A.O. (2011) Carbon export associated with free-drifting icebergs in the Southern Ocean. Deep Sea Research Part II: Topical Studies in Oceanography 58, 1485–1496.
), a change in iceberg Fe supply could significantly affect marine primary productivity. Yet there remain large uncertainties concerning the magnitude of iceberg Fe supply and its effect(s) on marine ecosystems. For example, calculated phytoplankton Fe utilisation is considerably less than present estimates of iceberg Fe supply to the Weddell Sea (Boyd et al., 2012Boyd, P.W., Arrigo, K.R., Strzepek, R., Van Dijken, G.L. (2012) Mapping phytoplankton iron utilization: Insights into Southern Ocean supply mechanisms. Journal Geophysical Research: Oceans 117, doi:10.1029/2011JC007726.
). The reason for this is unclear, yet it demonstrates the difficulty in isolating the contribution of icebergs to the marine Fe cycle.Observations of DFe concentrations in iceberg meltwater are sparse, but the available data does suggest a heterogeneous distribution, with DFe ranging 4–600 nM in Antarctic (Lin et al., 2011
Lin, H., Rauschenberg, S., Hexel, C.R., Shaw, T.J., Twining, B.S. (2011) Free-drifting icebergs as sources of iron to the Weddell Sea. Deep Sea Research Part II: Topical Studies in Oceanography 58, 1392–1406.
) and 3–300 nM in Greenlandic (Hopwood et al., 2016Hopwood, M.J., Connelly, D.P., Arendt, K.E., Juul-Pedersen, T., Stinchcombe, M., Meire, L., Esposito, M., Krishna, R. (2016) Seasonal changes in Fe along a glaciated Greenlandic fjord. Frontiers in Earth Sciences 4, doi:10.3389/feart.2016.00015.
) iceberg melt. The distribution of particulate Fe (which includes FeAsc) is also expected to be heterogeneous due to the presence of embedded sediment-rich layers that account for only a small fraction of total iceberg volume (Lin et al., 2011Lin, H., Rauschenberg, S., Hexel, C.R., Shaw, T.J., Twining, B.S. (2011) Free-drifting icebergs as sources of iron to the Weddell Sea. Deep Sea Research Part II: Topical Studies in Oceanography 58, 1392–1406.
; Raiswell, 2011Raiswell, R. (2011) Iceberg-hosted nanoparticulate Fe in the Southern Ocean: Mineralogy, origin, dissolution kinetics and source of bioavailable Fe. Deep Sea Research Part II: Topical Studies in Oceanography 58, 1364–1375.
; Raiswell et al., 2016Raiswell, R., Hawkings, J.R., Benning, L.G., Baker, A.R., Death, R., Albani, S., Mahowald, N., Krom, M.D., Poulton, S.W., Wadham, J., Tranter, M. (2016) Potentially bioavailable iron delivery by iceberg-hosted sediments and atmospheric dust to the polar oceans. Biogeosciences 13, 3887–3900.
). Whilst TdFe data for icebergs is sparse, iceberg FeAsc content has previously been estimated in multiple catchments worldwide (Raiswell et al., 2016Raiswell, R., Hawkings, J.R., Benning, L.G., Baker, A.R., Death, R., Albani, S., Mahowald, N., Krom, M.D., Poulton, S.W., Wadham, J., Tranter, M. (2016) Potentially bioavailable iron delivery by iceberg-hosted sediments and atmospheric dust to the polar oceans. Biogeosciences 13, 3887–3900.
) producing a mean global content of 2.7–17 µM. However, FeAsc content and offshore iceberg FeAsc fluxes are normally calculated using a mean sediment loading (0.5 g L-1 is widely used as outlined in Raiswell et al. (2016)Raiswell, R., Hawkings, J.R., Benning, L.G., Baker, A.R., Death, R., Albani, S., Mahowald, N., Krom, M.D., Poulton, S.W., Wadham, J., Tranter, M. (2016) Potentially bioavailable iron delivery by iceberg-hosted sediments and atmospheric dust to the polar oceans. Biogeosciences 13, 3887–3900.
) with considerable uncertainty generally acknowledged in this value. Here we combine the analysis of DFe, TdFe, FeAsc and iceberg sediment load in order to provide a well constrained assessment of iceberg-Fe content within a single catchment.top
Methods
A FeAsc dataset was compiled for icebergs in Kongsfjorden with visible embedded or surface sediment sampled from small boats in July 2015 and August 2016. Sediment from pro-glacial streambeds in the catchment, embedded sediment from Kongsvegen glacier surface, and embedded sediment ~100 m inside an ice crevasse (on Midtre Lovénbreen glacier) was also collected (Fig. 1) for comparative purposes. FeAsc leaches were conducted on wet sediment as per Raiswell et al. (2010)
Raiswell, R., Vu, H.P., Brinza, L., Benning, L.G. (2010) The determination of labile Fe in ferrihydrite by ascorbic acid extraction: Methodology, dissolution kinetics and loss of solubility with age and de-watering. Chemical Geology 278, 70–79.
, with leached Fe determined by measuring absorbance (λ = 562 nm) before and after the addition of ferrozine (as detailed in Supplementary Information Methods).Separately, ice samples (1–2 kg) were randomly collected from small boats (July 2015). The meltwater was acidified to pH < 2. After storage for 12 months, DFe and TdFe were measured by inductively coupled plasma mass spectroscopy (further details in Supplementary Information Methods).
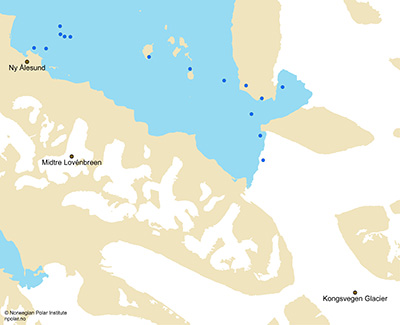
Figure 1 Surface fjord sample locations in Kongsfjorden.
top
Results
The FeAsc concentration is reported for 116 different sediment samples (Table S-2) including 58 iceberg samples collected from ice with visible embedded sediment. FeAsc ranged from 0.0042 to 0.12 wt. % in iceberg embedded sediment (Fig. 2). Ice sediment content ranged from <0.1 to 234 g L-1 of meltwater, close to the 0.2–200 g L-1 range previously reported in Svalbard by Dowdeswell and Dowdeswell (1989)
Dowdeswell, J.A., Dowdeswell, E.K. (1989) Debris in Icebergs and Rates of Glaci-Marine Sedimentation: Observations from Spitsbergen and a Simple Model. The Journal of Geology 97, 221–231.
. Combining FeAsc (wt. %) and sediment load (g L-1 of melted ice) produced a median FeAsc ice content of 2.5 µM. Given that our sampling strategy was to target sediment-rich ice, this should thereby be an over-estimate of median iceberg FeAsc content (L-1 of melted ice) in Kongsfjorden.
Figure 2 Median FeAsc (wt. %) with 25/75th (boxes) and 10/90th (whiskers) percentiles (outliers also shown) for iceberg embedded (n = 34) and iceberg surface (n = 20) sediment.
The DFe and TdFe concentrations are reported in parallel for 28 randomly collected iceberg samples (Table S-4). TdFe ranged from 46 nM to 57 µM (mean 3.6 µM, median 144 nM) and the range of 0.63 nM – 536 nM for DFe was similarly broad (mean 37 nM, median 6.5 nM). There was no significant correlation between particulate Fe (TdFe minus DFe) and DFe in these samples (Fig. 3), suggesting that DFe was not specifically associated with sediment laden ice. For comparison, DFe in surface fjord waters averaged 18 ± 17 nM (15 surface stations, Fig. 1) and TdFe ranged widely from 1.1 to 52 µM (mean 8.1 µM, median 3.7 µM) (Table S-3).
top
Discussion
As has been demonstrated in this study and elsewhere (e.g., Markussen et al., 2016
Markussen, T.N., Elberling, B., Winter, C., Andersen, T.J. (2016) Flocculated meltwater particles control Arctic land-sea fluxes of labile iron. Scientific Reports 6, 24033.
), surface waters in stratified, glaciated fjords can exhibit extremely high TdFe concentrations due to the presence of glacially derived particle plumes. TdFe concentrations in surface waters of Kongsfjorden (mean 8.1 µM, median 3.7 µM) exceeded those in icebergs (3.6 µM and 144 nM, respectively). In the Arctic, a large fraction of iceberg melt occurs in these inshore, high TdFe waters before icebergs are able to deliver Fe to the offshore environment. Accounting for this near-shore loss in flux calculations for iceberg derived Fe supply to the open ocean is difficult. In two Greenlandic catchments, Ilulissat Fjord and Sermilik Fjord, overall in-fjord iceberg volume losses were estimated to be >50 % (Enderlin et al., 2016Enderlin, E.M., Hamilton, G.S., Straneo, F., Sutherland, D.A. (2016) Iceberg meltwater fluxes dominate the freshwater budget in Greenland’s iceberg-congested glacial fjords. Geophysical Research Letters 43, 11287–11294.
), tentatively supporting the 50 % inshore iceberg volume loss used to estimate offshore FeAsc fluxes by Raiswell et al. (2016)Raiswell, R., Hawkings, J.R., Benning, L.G., Baker, A.R., Death, R., Albani, S., Mahowald, N., Krom, M.D., Poulton, S.W., Wadham, J., Tranter, M. (2016) Potentially bioavailable iron delivery by iceberg-hosted sediments and atmospheric dust to the polar oceans. Biogeosciences 13, 3887–3900.
. However this assumes that changes in total iceberg Fe content are directly proportional to changes in total ice volume.All measured Fe phases (DFe, TdFe and FeAsc) in Kongsfjorden were very heterogeneously distributed within the ice. For TdFe and FeAsc (but not DFe, Fig. 3), this can specifically be attributed to the heterogeneous distribution of ice embedded sediment. In the Arctic, iceberg-borne sediment is known to be lost from icebergs faster than ice volume (Mugford and Dowdeswell, 2010
Mugford, R.I., Dowdeswell, J.A. (2010) Modeling iceberg-rafted sedimentation in high-latitude fjord environments. Journal of Geophysical Research: Earth Surface 115, doi:10.1029/2009JF001564.
) due to its association with basal ice. Thus we expect that the mean TdFe content per volume of an iceberg should decline with time after calving. A model for Kangerdlugssuaq Fjord (Greenland) shows that whilst icebergs lose 20–30 % ice volume within this fjord, the corresponding in-fjord sediment loss is 70–85 % (Mugford and Dowdeswell, 2010Mugford, R.I., Dowdeswell, J.A. (2010) Modeling iceberg-rafted sedimentation in high-latitude fjord environments. Journal of Geophysical Research: Earth Surface 115, doi:10.1029/2009JF001564.
). Only a relatively small iceberg volume loss (<20 %) is thereby likely required for the majority of TdFe content to be lost from icebergs. In Kongsfjorden, where summer melting of calved ice is quite rapid due to relatively warm surface seawater (4–5 °C throughout July-August 2016), the post-calving age of an iceberg is therefore likely a critical factor in determining its TdFe content. Sediment loss should also affect mean FeAsc content in the same way, however FeAsc losses may be offset from TdFe losses if significant processing of surface sediment occurs on the timescale of iceberg Fe delivery (Raiswell et al., 2016Raiswell, R., Hawkings, J.R., Benning, L.G., Baker, A.R., Death, R., Albani, S., Mahowald, N., Krom, M.D., Poulton, S.W., Wadham, J., Tranter, M. (2016) Potentially bioavailable iron delivery by iceberg-hosted sediments and atmospheric dust to the polar oceans. Biogeosciences 13, 3887–3900.
).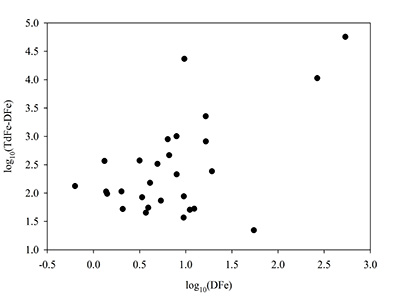
Figure 3 DFe and TdFe (both nM, plotted as log10, TdFe shown minus DFe) for 28 discrete iceberg samples showed no clear relationship.
In Kongsfjorden, Raiswell et al. (2016)
Raiswell, R., Hawkings, J.R., Benning, L.G., Baker, A.R., Death, R., Albani, S., Mahowald, N., Krom, M.D., Poulton, S.W., Wadham, J., Tranter, M. (2016) Potentially bioavailable iron delivery by iceberg-hosted sediments and atmospheric dust to the polar oceans. Biogeosciences 13, 3887–3900.
reported a FeAsc range of 0.016–0.37 wt. % (n = 14), with a mean of 0.14 wt. % and median of 0.092 wt. %; equivalent to 1.4–33 µM, 12 µM and 8.2 µM, respectively when using the suggested mean sediment loading of 0.5 g L-1. Comparing our data both as wt. % and as a µM concentration (calculated using measured sediment loading for each sample, range 0.1–234 g L-1, Table S-2), our FeAsc (wt. %) is consistently lower. Yet our mean FeAsc per volume is much higher (51 µM), because our measured sediment loadings were often greater than the assumed mean of 0.5 g L-1. These differences generally highlight the very high spatial variability in iceberg sediment load and thus TdFe and FeAsc content even within a single fjord.Table 1 Comparing data for Kongsfjorden from this and prior work suggests a critical difference in both FeAsc (wt. %) and in the scaling of FeAsc to iceberg sediment load (g L-1 of ice melt). *The suggested 0.5 g L-1 sediment loading is used for data from Raiswell et al. (2016)
Raiswell, R., Hawkings, J.R., Benning, L.G., Baker, A.R., Death, R., Albani, S., Mahowald, N., Krom, M.D., Poulton, S.W., Wadham, J., Tranter, M. (2016) Potentially bioavailable iron delivery by iceberg-hosted sediments and atmospheric dust to the polar oceans. Biogeosciences 13, 3887–3900.
. **For our study, measured sediment loadings were used for each sample. As sediment-rich ice was specifically targeted, the calculated mean/median should be over-estimates.
| a This study |
b Raiswell et al. (2016) Raiswell, R., Hawkings, J.R., Benning, L.G., Baker, A.R., Death, R., Albani, S., Mahowald, N., Krom, M.D., Poulton, S.W., Wadham, J., Tranter, M. (2016) Potentially bioavailable iron delivery by iceberg-hosted sediments and atmospheric dust to the polar oceans. Biogeosciences 13, 3887–3900. | a/b % | |
FeAsc / wt. % | Mean | 0,021 | 0,14 | 16 |
Median | 0,015 | 0,092 | 17 | |
FeAsc / µM (per litre of ice melt)* | Mean | <59 ** | 12 | 480 |
| Median | <2.5 ** | 8,2 | 31 |
Some methodological differences between this study and previous work could be important for the difference in FeAsc (wt. %) (Table 1). In our study, the sediment was not sieved to remove anomalous large particles. Yet a relatively large sub-sample mass was used with good reproducibility demonstrated. For glacial flour particles of <1 mm, it has previously been demonstrated that the change in FeAsc (wt. %) with particle size is not pronounced (Hopwood et al., 2014
Hopwood, M.J., Statham, P.J., Tranter, M., Wadham, J.L. (2014) Glacial flours as a potential source of Fe(II) and Fe(III) to polar waters. Biogeochemistry 118, 443–452.
; Raiswell et al., 2016Raiswell, R., Hawkings, J.R., Benning, L.G., Baker, A.R., Death, R., Albani, S., Mahowald, N., Krom, M.D., Poulton, S.W., Wadham, J., Tranter, M. (2016) Potentially bioavailable iron delivery by iceberg-hosted sediments and atmospheric dust to the polar oceans. Biogeosciences 13, 3887–3900.
), but this may not be the case for larger particles. Moreover, in this study sediment was processed in Svalbard with no prolonged storage between collection and analysis. Whilst dried sediment exhibits a rapid decline in FeAsc wt. % (Raiswell et al., 2010Raiswell, R., Vu, H.P., Brinza, L., Benning, L.G. (2010) The determination of labile Fe in ferrihydrite by ascorbic acid extraction: Methodology, dissolution kinetics and loss of solubility with age and de-watering. Chemical Geology 278, 70–79.
), it is not clear how storage of ice or wet sediments affects FeAsc.Furthermore, there are the critical issues of heterogeneity and of the non-linearity between iceberg sediment and iceberg volume losses. In-fjord iceberg volume loss should correspond to a disproportionately high loss of iceberg embedded sediment (Mugford and Dowdeswell, 2010
Mugford, R.I., Dowdeswell, J.A. (2010) Modeling iceberg-rafted sedimentation in high-latitude fjord environments. Journal of Geophysical Research: Earth Surface 115, doi:10.1029/2009JF001564.
), and thereby also FeAsc and TdFe. There are no quantitative measures of iceberg age or volume loss for our dataset and the residence time of ice in Kongsfjorden is strongly affected by meteorological conditions and thus subject to high short-term variability. Nonetheless, a difference in the post-calving age of ice sampled between different datasets could correspond to large shifts in iceberg FeAsc and TdFe content. Increased iceberg age would be expected to correspond to lower mean sediment load, and thereby lower TdFe and FeAsc per volume. A reduction in basal sediment load could also explain a difference in FeAsc (wt. %) content if FeAsc (wt. %) is enriched in basal ice compared to non-basal ice. FeAsc (µmol L-1) is correlated with sediment load (Fig. 4), but assessing whether changes in sediment load affect FeAsc (wt. %) is complicated by the lack of any parameter to account for the post-calving age of ice and by the highly variable bedrock composition across Kongsfjorden (see for example Hjelle, 1993Hjelle, A. (1993) The geology of Svalbard: Oslo. Polarhåndbok nr. 6. Norsk Polarinstitutt, Oslo.
).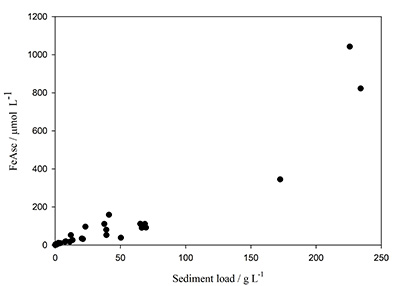
Figure 4 FeAsc (µmol L-1 melted ice) increased with sediment load (g L-1 melted ice), but it is unclear if the relationship remains linear at high (>50 g L-1) loadings.
top
Conclusions
Whilst median DFe (6.5 nM) and TdFe (144 nM) concentrations in Kongsfjorden were within the range of concentrations reported elsewhere globally, the median FeAsc concentration (2.5 µM) measured was considerably lower than that reported previously in Kongsfjorden, and compared to present estimates of the global mean, despite the very high sediment loadings observed (<0.1 – 234 g L-1). Generally in the Arctic, a sharp decline in the mean FeAsc and TdFe per volume of meltwater from icebergs with time after calving would be expected due to the preferential loss of iceberg basal ice, as modelled by Mugford and Dowdeswell (2010)
Mugford, R.I., Dowdeswell, J.A. (2010) Modeling iceberg-rafted sedimentation in high-latitude fjord environments. Journal of Geophysical Research: Earth Surface 115, doi:10.1029/2009JF001564.
. Iceberg derived fluxes of TdFe and FeAsc are thereby biased towards delivery in near-shore waters and offshore fluxes are likely much less than if TdFe and FeAsc were homogeneously distributed throughout icebergs.top
Glossary
‘Fe’ refers to all iron phases.
‘DFe’, dissolved Fe, refers to all Fe phases <0.2 µm.
‘FeAsc’ is the ferrihydrite content of sediment, defined by Raiswell et al. (2010)
Raiswell, R., Vu, H.P., Brinza, L., Benning, L.G. (2010) The determination of labile Fe in ferrihydrite by ascorbic acid extraction: Methodology, dissolution kinetics and loss of solubility with age and de-watering. Chemical Geology 278, 70–79.
.‘TdFe’ is all Fe soluble at pH < 2, inclusive of DFe and should also include any FeAsc present in unfiltered meltwater.
top
Acknowledgements
Financial aid from the European Commission (OCEAN-CERTAIN, FP7- ENV-2013-6.1-1; no: 603773) is gratefully acknowledged. 2016 fieldwork was conducted during the CNR Dirigibilite Italia hosted project ‘pH in Svalbard’.
Editor: Liane G. Benning
top
References
Bamber, J., van den Broeke, M., Ettema, J., Lenaerts, J., Rignot, E. (2012) Recent large increases in freshwater fluxes from Greenland into the North Atlantic. Geophysical Research Letters 39, L19501, doi:10.1029/2012gl052552.

As the rate of iceberg calving in polar seas oscillates on glacial to inter-glacial timescales (Bond et al., 1992), and recent climate change has increased the discharge of calved ice volume from both the Antarctic (Paolo et al., 2015) and Greenlandic (Bamber et al., 2012) ice sheets, Fe delivery from icebergs may also change.
View in article
Bond, G., Heinrich, H., Broecker, W.S., Labeyrie, L., McManus, J.F., Andrews, J.T., Huon, S., Jantschik, R., Clasen, S., Simet, C., Tedesco, K., Klas, M., Bonani, G., Ivy, S. (1992) Evidence for massive discharges of icebergs into the North Atlantic ocean during the last glacial period, Nature 360, 245–249.

As the rate of iceberg calving in polar seas oscillates on glacial to inter-glacial timescales (Bond et al., 1992), and recent climate change has increased the discharge of calved ice volume from both the Antarctic (Paolo et al., 2015) and Greenlandic (Bamber et al., 2012) ice sheets, Fe delivery from icebergs may also change.
View in article
Boyd, P.W., Arrigo, K.R., Strzepek, R., Van Dijken, G.L. (2012) Mapping phytoplankton iron utilization: Insights into Southern Ocean supply mechanisms. Journal Geophysical Research: Oceans 117, doi:10.1029/2011JC007726.

For example, calculated phytoplankton Fe utilisation is considerably less than present estimates of iceberg Fe supply to the Weddell Sea (Boyd et al., 2012).
View in article
De Baar, H.J.W., De Jong, J.T.M., Bakker, D.C.E., Loscher, B.M., Veth, C., Bathmann, U., Smetacek, V. (1995) Importance of iron for plankton blooms and carbon dioxide drawdown in the Southern Ocean. Nature 373, 412–415.

Icebergs contain higher Fe concentrations than seawater, both in the dissolved (<0.2 µm) (Martin et al., 1990; De Baar et al., 1995; Loscher et al., 1997) and particulate (>0.2 µm) phases (Hart, 1934; Lin et al., 2011; Shaw et al., 2011).
View in article
Dowdeswell, J.A., Dowdeswell, E.K. (1989) Debris in Icebergs and Rates of Glaci-Marine Sedimentation: Observations from Spitsbergen and a Simple Model. The Journal of Geology 97, 221–231.

Ice sediment content ranged from <0.1 to 234 g L-1 of meltwater, close to the 0.2–200 g L-1 range previously reported in Svalbard by Dowdeswell and Dowdeswell (1989).
View in article
Enderlin, E.M., Hamilton, G.S., Straneo, F., Sutherland, D.A. (2016) Iceberg meltwater fluxes dominate the freshwater budget in Greenland’s iceberg-congested glacial fjords. Geophysical Research Letters 43, 11287–11294.

In two Greenlandic catchments, Ilulissat Fjord and Sermilik Fjord, overall in-fjord iceberg volume losses were estimated to be >50 % (Enderlin et al., 2016), tentatively supporting the 50 % inshore iceberg volume loss used to estimate offshore FeAsc fluxes by Raiswell et al. (2016).
View in article
Hart, T.J. (1934) Discovery Reports. Discovery Reports 8, 1–268.

Icebergs contain higher Fe concentrations than seawater, both in the dissolved (<0.2 µm) (Martin et al., 1990; De Baar et al., 1995; Loscher et al., 1997) and particulate (>0.2 µm) phases (Hart, 1934; Lin et al., 2011; Shaw et al., 2011).
View in article
Hjelle, A. (1993) The geology of Svalbard: Oslo. Polarhåndbok nr. 6. Norsk Polarinstitutt, Oslo.

FeAsc (µmol L-1) is correlated with sediment load (Fig. 4), but assessing whether changes in sediment load affect FeAsc (wt. %) is complicated by the lack of any parameter to account for the post-calving age of ice and by the highly variable bedrock composition across Kongsfjorden (see for example Hjelle, 1993).
View in article
Hopwood, M.J., Connelly, D.P., Arendt, K.E., Juul-Pedersen, T., Stinchcombe, M., Meire, L., Esposito, M., Krishna, R. (2016) Seasonal changes in Fe along a glaciated Greenlandic fjord. Frontiers in Earth Sciences 4, doi:10.3389/feart.2016.00015.

Observations of DFe concentrations in iceberg meltwater are sparse, but the available data does suggest a heterogeneous distribution, with DFe ranging 4–600 nM in Antarctic (Lin et al., 2011) and 3–300 nM in Greenlandic (Hopwood et al., 2016) iceberg melt.
View in article
Hopwood, M.J., Statham, P.J., Tranter, M., Wadham, J.L. (2014) Glacial flours as a potential source of Fe(II) and Fe(III) to polar waters. Biogeochemistry 118, 443–452.

For glacial flour particles of <1 mm, it has previously been demonstrated that the change in FeAsc (wt. %) with particle size is not pronounced (Hopwood et al., 2014; Raiswell et al., 2016), but this may not be the case for larger particles.
View in article
Lin, H., Rauschenberg, S., Hexel, C.R., Shaw, T.J., Twining, B.S. (2011) Free-drifting icebergs as sources of iron to the Weddell Sea. Deep Sea Research Part II: Topical Studies in Oceanography 58, 1392–1406.

Icebergs contain higher Fe concentrations than seawater, both in the dissolved (<0.2 µm) (Martin et al., 1990; De Baar et al., 1995; Loscher et al., 1997) and particulate (>0.2 µm) phases (Hart, 1934; Lin et al., 2011; Shaw et al., 2011).
View in article
Observations of DFe concentrations in iceberg meltwater are sparse, but the available data does suggest a heterogeneous distribution, with DFe ranging 4–600 nM in Antarctic (Lin et al., 2011) and 3–300 nM in Greenlandic (Hopwood et al., 2016) iceberg melt.
View in article
The distribution of particulate Fe (which includes FeAsc) is also expected to be heterogeneous due to the presence of embedded sediment-rich layers that account for only a small fraction of total iceberg volume (Lin et al., 2011; Raiswell, 2011; Raiswell et al., 2016).
View in article
Loscher, B.M., De Baar, H.J.W., De Jong, J.T.M., Veth, C., Dehairs, F. (1997) The distribution of Fe in the Antarctic Circumpolar Current. Deep Sea Research Part II: Topical Studies in Oceanography 44, 143–187.

Icebergs contain higher Fe concentrations than seawater, both in the dissolved (<0.2 µm) (Martin et al., 1990; De Baar et al., 1995; Loscher et al., 1997) and particulate (>0.2 µm) phases (Hart, 1934; Lin et al., 2011; Shaw et al., 2011).
View in article
Markussen, T.N., Elberling, B., Winter, C., Andersen, T.J. (2016) Flocculated meltwater particles control Arctic land-sea fluxes of labile iron. Scientific Reports 6, 24033.

As has been demonstrated in this study and elsewhere (e.g., Markussen et al., 2016), surface waters in stratified, glaciated fjords can exhibit extremely high TdFe concentrations due to the presence of glacially derived particle plumes.
View in article
Martin, J.H., Gordon, R.M., Fitzwater, S.E. (1990) Iron in Antarctic waters. Nature 345, 156–158.

Icebergs contain higher Fe concentrations than seawater, both in the dissolved (<0.2 µm) (Martin et al., 1990; De Baar et al., 1995; Loscher et al., 1997) and particulate (>0.2 µm) phases (Hart, 1934; Lin et al., 2011; Shaw et al., 2011).
View in article
Particularly in the Southern Ocean, where DFe deficiency extensively limits primary production (Martin et al., 1990, 1991; Moore et al., 2013), and icebergs cause chemical and biological enrichment of surrounding waters (Smith Jr. et al., 2007; Schwarz and Schodlok, 2009; Smith et al., 2011), a change in iceberg Fe supply could significantly affect marine primary productivity.
View in article
Martin, J.H., Gordon, R.M., Fitzwater, S.E. (1991)The case for iron. Limnology and Oceanography 36, 1793–1802.

Particularly in the Southern Ocean, where DFe deficiency extensively limits primary production (Martin et al., 1990, 1991; Moore et al., 2013), and icebergs cause chemical and biological enrichment of surrounding waters (Smith Jr. et al., 2007; Schwarz and Schodlok, 2009; Smith et al., 2011), a change in iceberg Fe supply could significantly affect marine primary productivity.
View in article
Moore, C.M., Mills, M.M., Arrigo, K.R., Berman-Frank, I., Bopp, L., Boyd, P.W., Galbraith, E.D., Geider, R.J., Guieu, C., Jaccard, S.L., Jickells, T.D., La Roche, J., Lenton, T.M., Mahowald, N.M., Maranon, E., Marinov, I., Moore, J.K., Nakatsuka, T., Oschlies, A., Saito, M.A., Thingstad, T.F., Tsuda, A., Ulloa, O. (2013) Processes and patterns of oceanic nutrient limitation. Nature Geoscience 6, 701–710.

Particularly in the Southern Ocean, where DFe deficiency extensively limits primary production (Martin et al., 1990, 1991; Moore et al., 2013), and icebergs cause chemical and biological enrichment of surrounding waters (Smith Jr. et al., 2007; Schwarz and Schodlok, 2009; Smith et al., 2011), a change in iceberg Fe supply could significantly affect marine primary productivity.
View in article
Mugford, R.I., Dowdeswell, J.A. (2010) Modeling iceberg-rafted sedimentation in high-latitude fjord environments. Journal of Geophysical Research: Earth Surface 115, doi:10.1029/2009JF001564.

In the Arctic, iceberg-borne sediment is known to be lost from icebergs faster than ice volume (Mugford and Dowdeswell, 2010) due to its association with basal ice.
View in article
A model for Kangerdlugssuaq Fjord (Greenland) shows that whilst icebergs lose 20–30 % ice volume within this fjord, the corresponding in-fjord sediment loss is 70–85 % (Mugford and Dowdeswell, 2010).
View in article
In-fjord iceberg volume loss should correspond to a disproportionately high loss of iceberg embedded sediment (Mugford and Dowdeswell, 2010), and thereby also FeAsc and TdFe.
View in article
Generally in the Arctic, a sharp decline in the mean FeAsc and TdFe per volume of meltwater from icebergs with time after calving would be expected due to the preferential loss of iceberg basal ice, as modelled by Mugford and Dowdeswell (2010).
View in article
Paolo, F.S., Fricker, H.A., Padman, L. (2015) Volume loss from Antarctic ice shelves is accelerating. Science 348, 327–331.

As the rate of iceberg calving in polar seas oscillates on glacial to inter-glacial timescales (Bond et al., 1992), and recent climate change has increased the discharge of calved ice volume from both the Antarctic (Paolo et al., 2015) and Greenlandic (Bamber et al., 2012) ice sheets, Fe delivery from icebergs may also change.
View in article
Raiswell, R. (2011) Iceberg-hosted nanoparticulate Fe in the Southern Ocean: Mineralogy, origin, dissolution kinetics and source of bioavailable Fe. Deep Sea Research Part II: Topical Studies in Oceanography 58, 1364–1375.

The distribution of particulate Fe (which includes FeAsc) is also expected to be heterogeneous due to the presence of embedded sediment-rich layers that account for only a small fraction of total iceberg volume (Lin et al., 2011; Raiswell, 2011; Raiswell et al., 2016).
View in article
Raiswell, R., Benning, L.G., Tranter, M., Tulaczyk, S. (2008) Bioavailable iron in the Southern Ocean: the significance of the iceberg conveyor belt. Geochemical Transactions 9, doi:10.1186/1467-4866-9-7.

Icebergs should thus constitute a source of the micronutrient Fe to offshore polar waters (Raiswell et al., 2008).
View in article
Raiswell, R., Vu, H.P., Brinza, L., Benning, L.G. (2010) The determination of labile Fe in ferrihydrite by ascorbic acid extraction: Methodology, dissolution kinetics and loss of solubility with age and de-watering. Chemical Geology 278, 70–79.

FeAsc leaches were conducted on wet sediment as per Raiswell et al. (2010), with leached Fe determined by measuring absorbance (λ = 562 nm) before and after the addition of ferrozine (as detailed in Supplementary Information Methods).
View in article
Whilst dried sediment exhibits a rapid decline in FeAsc wt. % (Raiswell et al., 2010), it is not clear how storage of ice or wet sediments affects FeAsc.
View in article
‘FeAsc’ is the ferrihydrite content of sediment, defined by Raiswell et al. (2010).
View in article
Raiswell, R., Hawkings, J.R., Benning, L.G., Baker, A.R., Death, R., Albani, S., Mahowald, N., Krom, M.D., Poulton, S.W., Wadham, J., Tranter, M. (2016) Potentially bioavailable iron delivery by iceberg-hosted sediments and atmospheric dust to the polar oceans. Biogeosciences 13, 3887–3900.

The distribution of particulate Fe (which includes FeAsc) is also expected to be heterogeneous due to the presence of embedded sediment-rich layers that account for only a small fraction of total iceberg volume (Lin et al., 2011; Raiswell, 2011; Raiswell et al., 2016).
View in article
Whilst TdFe data for icebergs is sparse, iceberg FeAsc content has previously been estimated in multiple catchments worldwide (Raiswell et al., 2016) producing a mean global content of 2.7–17 µM.
View in article
However, FeAsc content and offshore iceberg FeAsc fluxes are normally calculated using a mean sediment loading (0.5 g L-1 is widely used as outlined in Raiswell et al. (2016)) with considerable uncertainty generally acknowledged in this value.
View in article
In two Greenlandic catchments, Ilulissat Fjord and Sermilik Fjord, overall in-fjord iceberg volume losses were estimated to be >50 % (Enderlin et al., 2016), tentatively supporting the 50 % inshore iceberg volume loss used to estimate offshore FeAsc fluxes by Raiswell et al. (2016).
View in article
Sediment loss should also affect mean FeAsc content in the same way, however FeAsc losses may be offset from TdFe losses if significant processing of surface sediment occurs on the timescale of iceberg Fe delivery (Raiswell et al., 2016).
View in article
In Kongsfjorden, Raiswell et al. (2016) reported a FeAsc range of 0.016–0.37 wt. % (n = 14), with a mean of 0.14 wt. % and median of 0.092 wt. %; equivalent to 1.4–33 µM, 12 µM and 8.2 µM, respectively when using the suggested mean sediment loading of 0.5 g L-1.
View in article
Table 1 [...] *The suggested 0.5 g L-1 sediment loading is used for data from Raiswell et al. (2016).
View in article
For glacial flour particles of <1 mm, it has previously been demonstrated that the change in FeAsc (wt. %) with particle size is not pronounced (Hopwood et al., 2014; Raiswell et al., 2016), but this may not be the case for larger particles.
View in article
Schwarz, J.N., Schodlok, M.P. (2009) Impact of drifting icebergs on surface phytoplankton biomass in the Southern Ocean: Ocean colour remote sensing and in situ iceberg tracking. Deep Sea Research Part I: Oceanographic Research Papers 56, 1727–1741.

Particularly in the Southern Ocean, where DFe deficiency extensively limits primary production (Martin et al., 1990, 1991; Moore et al., 2013), and icebergs cause chemical and biological enrichment of surrounding waters (Smith Jr. et al., 2007; Schwarz and Schodlok, 2009; Smith et al., 2011), a change in iceberg Fe supply could significantly affect marine primary productivity.
View in article
Shaw, T.J., Raiswell, R., Hexel, C.R., Vu, H.P., Moore, W.S., Dudgeon, R., Smith Jr., K.L. (2011) Input, composition, and potential impact of terrigenous material from free-drifting icebergs in the Weddell Sea. Deep Sea Research Part II: Topical Studies in Oceanography 58, 1376–1383.

Icebergs contain higher Fe concentrations than seawater, both in the dissolved (<0.2 µm) (Martin et al., 1990; De Baar et al., 1995; Loscher et al., 1997) and particulate (>0.2 µm) phases (Hart, 1934; Lin et al., 2011; Shaw et al., 2011).
View in article
Smith, K.L., Sherman, A.D., Shaw, T.J., Murray, A.E., Vernet, M., Cefarelli, A.O. (2011) Carbon export associated with free-drifting icebergs in the Southern Ocean. Deep Sea Research Part II: Topical Studies in Oceanography 58, 1485–1496.

Particularly in the Southern Ocean, where DFe deficiency extensively limits primary production (Martin et al., 1990, 1991; Moore et al., 2013), and icebergs cause chemical and biological enrichment of surrounding waters (Smith Jr. et al., 2007; Schwarz and Schodlok, 2009; Smith et al., 2011), a change in iceberg Fe supply could significantly affect marine primary productivity.
View in article
Smith Jr., K.L., Robison, B.H., Helly, J.J., Kaufmann, R.S., Ruhl, H.A., Shaw, T.J., Twining, B.S., Vernet, M. (2007) Free-drifting icebergs: Hot spots of chemical and biological enrichment in the Weddell Sea. Science 317, 478–482.

Particularly in the Southern Ocean, where DFe deficiency extensively limits primary production (Martin et al., 1990, 1991; Moore et al., 2013), and icebergs cause chemical and biological enrichment of surrounding waters (Smith Jr. et al., 2007; Schwarz and Schodlok, 2009; Smith et al., 2011), a change in iceberg Fe supply could significantly affect marine primary productivity.
View in article
top
Supplementary Information
Supplementary Methods
Ice sample collection
All ice samples were collected from calved ice masses within Kongsfjorden east of 11.89° E. Sampled icebergs had a maximum length (visible above the waterline) of between 0.4 and 20 m, and a maximum height above the waterline of between 10 cm and 4 m. For FeAsc, icebergs with visible sediment layers (darkened layers approximately 0.5 to 5 cm thick) were targeted whereas sample collection for DFe/TdFe was random.
FeAsc
To measure FeAsc, 1–2 kg ice pieces were returned to the laboratory in insulated plastic boxes. As a precaution against contamination, ice was rinsed with de-ionised water (Milli-Q, Millipore, conductivity 18.2 MΩ cm-1). Ice was then melted in low density polyethylene (LDPE) bags with the first (1–2 hr later) meltwater discarded. After melting ice overnight, the total volume was recorded and then excess water was removed slowly (<5 mL min-1) by filtration (0.45 µm, polyvinylidene fluoride, Millipore) to concentrate the sediment. All plastic vials and filtration equipment for sediment collection/handling was pre-cleaned (1 M HCl, 3 de-ionised water rinses) prior to use. FeAsc was determined as per Raiswell et al. (2010) but using larger (~100 mg) sediment samples without sieving. Leached Fe was determined by measuring absorbance (λ = 562 nm) before, and after, the addition of ferrozine (Stookey, 1970) using a 1 cm cell with a USB4000 Fiber-optic Spectrometer and a LS-1 tungsten halogen light source (Ocean Optics). Fe standards were made by spiking DFe from an acidified (pH 2) 1 mM Fe stock solution into aliquots of ascorbic leaching solution producing a linear response (R2 > 0.99, derived molar adsorption coefficient 24,500 M-1 cm-1) over the absorbance range 0–0.6. Sample absorbance was then corrected for background absorbance and dilution by reagents.
A sub-sample of sediment was retained (except for sediment loadings <0.1 g L-1 where this was not possible) and air dried to constant mass in order to calculate FeAsc content g-1 (of sediment) and L-1 (of meltwater). FeAsc measurements were duplicated for 11 randomly selected samples producing a relative standard deviation of 4.5 %. The propagated standard deviation for FeAsc L-1 of meltwater (obtained from combining sediment load with FeAsc wt. %) was approximately 15 %.
DFe and TdFe
125 mL LDPE bottles (Nalgene) were pre-cleaned in a 3 stage process with 3 de-ionised water rinses after each stage (detergent, 1.2 M HCl, 1.2 M HNO3). After melting ice in LDPE bags, as above, 125 mL meltwater was retained without filtration for TdFe, and 125 mL was syringe filtered for DFe analysis (0.20 µm, polyvinylidene fluoride, Millipore). TdFe and DFe samples were acidified (with HCl, UPA, ROMIL) to pH < 2.0 and subsequently stored for 12 months to ensure complete recovery of soluble Fe (Edwards and Sedwick, 2001). DFe and TdFe were analysed by ICP-MS (ELEMENT XR, ThermoFisherScientific) after dilution with 1 M HNO3 (distilled in house using a DST-1000, Savillex, from SPA grade HNO3, ROMIL) and calibrated by standard addition with a linear peak response from 0–1000 nM Fe (R2 > 0.99). The analytical blank (DFe and TdFe) was always <0.6 nM Fe. To verify that the dilution technique yielded reproducible and accurate DFe results, NASS-7 and CASS-6 Certified Reference Materials (CRMs, National Research Council Canada) were analysed for Fe. Both CRMs yielded reproducible Fe concentrations within the certified ranges (Table S-1).
Table S-1 Analysis of Certified Reference Materials for Fe concentration (± standard deviation of at least 6 measurements).
Certified Reference Material | Fe (± SD) / nM | Certified Fe concentration / nM |
NASS-7 | 6.21 (± 0.77) | 6.29 (± 0.47) |
CASS-6 | 26.6 (± 0.71) | 27.9 (± 2.1) |
A linear plot of all paired DFe and TdFe minus DFe (n = 28) yielded R2 = 0.78. However, R2 declined to 0.01 when the 3 highest TdFe data were removed. A Spearman Rank Order Correlation suggested there was no significant relationship between DFe and TdFe minus DFe (P value 0.21). A linear plot of all FeAsc (mol L-1) data and corresponding ice sediment load (g L-1) yielded R2 = 0.88. A Spearman Rank Order Correlation Coefficient of 0.95 (P value 2 × 10-7, n = 58) demonstrates that the relationship is significant and remains significant if the 3 highest sediment loadings are removed (Coefficient 0.94, P value 2 × 10-7, n = 55) - regardless of whether the sediment loads reported as <0.1 g L-1 are treated as 0.1 g L-1, 0.01 g L-1, or excluded. Statistics were performed in SigmaPlot 13.
Water column
In July 2015, 15 samples of fjord surface water (depth <0.2 m) were collected by hand upstream of a small boat (locations shown Fig. 1). As per ice samples for DFe and TdFe, 125 mL was retained without filtration and 125 mL was syringe filtered for DFe analysis (0.20 µm, polyvinylidene fluoride, Millipore). Analysis was conducted via ICP-MS after storage for 12 months at pH < 2, and dilution with 1 M HNO3 (as per melted ice, above). The temperature and salinity of surface fjord water were recorded using a LF 325 conductivity meter (WTW) which was calibrated before use with a KCl solution.
In July–August 2016 conductivity, temperature, depth profiles (CTDs) were acquired at 20 stations in the area of the fjord where ice was observed and collected (east of 11.89° E and proceeding to within approximately 400 m of each marine terminating glacier in Kongsfjorden, Fig. 1). Seawater temperature (recorded at 1 m depth) was consistently 4.0–5.0 °C. A broader range of surface temperatures were observed in July 2015 (2.6–8.8 °C at <0.2 m), but are not directly comparable because of the depth difference.
Supplementary Material
Table S-2 FeAsc concentration reported for various sediment samples collected around Kongsfjorden. For iceberg embedded sediment with sediment loadings >0.1 g L-1, FeAsc is reported both as wt. % and per volume of meltwater.
Sample number | Origin | FeAsc wt. % | *FeAsc µmol / L | *Sediment loading g / L |
1 | Embedded sediment , glacier crevasse (Midtre Lovénbreen) | 0.014 | ||
2 | Embedded sediment , glacier crevasse (Midtre Lovénbreen) | 0.0057 | ||
3 | Embedded sediment , glacier crevasse (Midtre Lovénbreen) | 0.17 | ||
4 | Embedded sediment , glacier crevasse (Midtre Lovénbreen) | 0.023 | ||
5 | Embedded sediment , glacier crevasse (Midtre Lovénbreen) | 0.0038 | ||
6 | Embedded sediment , glacier crevasse (Midtre Lovénbreen) | 0.053 | ||
7 | Glacier surface sediment (Kongsvegen) | 0.016 | ||
8 | Glacier surface sediment (Kongsvegen) | 0.016 | ||
9 | Glacier surface sediment (Kongsvegen) | 0.012 | ||
10 | Glacier surface sediment (Kongsvegen) | 0.010 | ||
11 | Glacier surface sediment (Kongsvegen) | 0.016 | ||
12 | Glacier surface sediment (Kongsvegen) | 0.012 | ||
13 | Glacier surface sediment (Kongsvegen) | 0.014 | ||
14 | Iceberg embedded sediment | 0.009 | 110 | 69 |
15 | Iceberg embedded sediment | 0.0093 | 34 | 20 |
16 | Iceberg embedded sediment | 0.0076 | 90 | 66 |
17 | Iceberg embedded sediment | 0.0074 | 52 | 39 |
18 | Iceberg embedded sediment | 0.0085 | 17 | 11 |
19 | Iceberg embedded sediment | 0.016 | 2.5 | 0.91 |
20 | Iceberg embedded sediment | 0.0089 | 1.2 | 0.76 |
21 | Iceberg embedded sediment | 0.085 | 3.9 | 0.25 |
22 | Iceberg embedded sediment | 0.014 | 9.6 | 3.9 |
23 | Iceberg embedded sediment | 0.020 | 820 | 230 |
24 | Iceberg embedded sediment | 0.034 | 3.9 | 0.65 |
25 | Iceberg embedded sediment | 0.011 | 26 | 13 |
26 | Iceberg embedded sediment | 0.026 | 1000 | 230 |
27 | Iceberg embedded sediment | 0.023 | 96 | 23 |
28 | Iceberg embedded sediment | 0.0042 | 38 | 50 |
29 | Iceberg embedded sediment | 0.022 | 160 | 41 |
30 | Iceberg embedded sediment | 0.016 | 110 | 38 |
31 | Iceberg embedded sediment | 0.0082 | 31 | 21 |
32 | Iceberg embedded sediment | 0.024 | 52 | 12 |
33 | Iceberg embedded sediment | 0.0095 | 110 | 65 |
34 | Iceberg embedded sediment | 0.0073 | 91 | 70 |
35 | Iceberg embedded sediment | 0.015 | 5.0 | 1.8 |
36 | Iceberg embedded sediment | 0.013 | 17 | 7.5 |
37 | Iceberg embedded sediment | 0.011 | 340 | 170 |
38 | Iceberg embedded sediment | 0.027 | 7.8 | 1.6 |
39 | Iceberg embedded sediment | 0.026 | 1.2 | 0.25 |
40 | Iceberg embedded sediment | 0.025 | 11 | 2.4 |
41 | Iceberg embedded sediment | 0.12 | 3.0 | 0.14 |
42 | Iceberg embedded sediment | 0.028 | 2.5 | 0.51 |
43 | Iceberg embedded sediment | 0.013 | 19 | 8.1 |
44 | Iceberg embedded sediment | 0.011 | 80 | 39 |
45 | Iceberg embedded sediment | 0.020 | 3.6 | 0.99 |
46 | Iceberg embedded sediment | 0.026 | 1.2 | 0.26 |
47 | Iceberg embedded sediment | 0.012 | 2.1 | 1.0 |
48 | Iceberg embedded sediment | n/d | 0.26 | < 0.1 |
49 | Iceberg embedded sediment | n/d | 0.26 | < 0.1 |
50 | Iceberg embedded sediment | n/d | 0.87 | < 0.1 |
51 | Iceberg embedded sediment | n/d | 0.65 | < 0.1 |
52 | Iceberg embedded sediment | n/d | 0.27 | < 0.1 |
53 | Iceberg embedded sediment | n/d | 0.50 | < 0.1 |
54 | Iceberg embedded sediment | n/d | 0.28 | < 0.1 |
55 | Iceberg embedded sediment | n/d | 0.63 | < 0.1 |
56 | Iceberg embedded sediment | n/d | 0.40 | < 0.1 |
57 | Iceberg embedded sediment | n/d | 0.69 | < 0.1 |
58 | Iceberg embedded sediment | n/d | 0.37 | < 0.1 |
59 | Iceberg embedded sediment | n/d | 0.43 | < 0.1 |
60 | Iceberg embedded sediment | n/d | 0.68 | < 0.1 |
61 | Iceberg embedded sediment | n/d | 0.87 | < 0.1 |
62 | Iceberg embedded sediment | n/d | 0.27 | < 0.1 |
63 | Iceberg embedded sediment | n/d | 0.39 | < 0.1 |
64 | Iceberg embedded sediment | n/d | 1.8 | < 0.1 |
65 | Iceberg embedded sediment | n/d | 0.40 | < 0.1 |
66 | Iceberg embedded sediment | n/d | 0.77 | < 0.1 |
67 | Iceberg embedded sediment | n/d | 0.35 | < 0.1 |
68 | Iceberg embedded sediment | n/d | 0.29 | < 0.1 |
69 | Iceberg embedded sediment | n/d | 0.32 | < 0.1 |
70 | Iceberg embedded sediment | n/d | 0.20 | < 0.1 |
71 | Iceberg embedded sediment | n/d | 0.15 | < 0.1 |
72 | Iceberg surface sediment | 0.0065 | ||
73 | Iceberg surface sediment | 0.0068 | ||
74 | Iceberg surface sediment | 0.0091 | ||
75 | Iceberg surface sediment | 0.0072 | ||
76 | Iceberg surface sediment | 0.024 | ||
77 | Iceberg surface sediment | 0.0071 | ||
78 | Iceberg surface sediment | 0.032 | ||
79 | Iceberg surface sediment | 0.010 | ||
80 | Iceberg surface sediment | 0.012 | ||
81 | Iceberg surface sediment | 0.021 | ||
82 | Iceberg surface sediment | 0.0093 | ||
83 | Iceberg surface sediment | 0.0077 | ||
84 | Iceberg surface sediment | 0.059 | ||
85 | Iceberg surface sediment | 0.0066 | ||
86 | Iceberg surface sediment | 0.0080 | ||
87 | Iceberg surface sediment | 0.0080 | ||
88 | Iceberg surface sediment | 0.029 | ||
89 | Iceberg surface sediment | 0.032 | ||
90 | Iceberg surface sediment | 0.0087 | ||
91 | Iceberg surface sediment | 0.0098 | ||
92 | Pro-glacial stream, glacial flour | 0.0090 | ||
93 | Pro-glacial stream, glacial flour | 0.0083 | ||
94 | Pro-glacial stream, glacial flour | 0.0063 | ||
95 | Pro-glacial stream, glacial flour | 0.0079 | ||
96 | Pro-glacial stream, glacial flour | 0.0072 | ||
97 | Pro-glacial stream, glacial flour | 0.0070 | ||
98 | Pro-glacial stream, glacial flour | 0.0073 | ||
99 | Pro-glacial stream, glacial flour | 0.019 | ||
100 | Pro-glacial stream, glacial flour | 0.031 | ||
101 | Pro-glacial stream, glacial flour | 0.010 | ||
102 | Pro-glacial stream, glacial flour | 0.0078 | ||
103 | Pro-glacial stream, glacial flour | 0.010 | ||
104 | Pro-glacial stream, glacial flour | 0.0066 | ||
105 | Pro-glacial stream, glacial flour | 0.0089 | ||
106 | Pro-glacial stream, glacial flour | 0.0096 | ||
107 | Pro-glacial stream, glacial flour | 0.0041 | ||
108 | Pro-glacial stream, glacial flour | 0.0032 | ||
109 | Pro-glacial stream, glacial flour | 0.0057 | ||
110 | Pro-glacial stream, glacial flour | 0.0079 | ||
111 | Surface glacier (Kongsvegen) embedded sediment | 0.044 | ||
112 | Surface glacier (Kongsvegen) embedded sediment | 0.071 | ||
113 | Surface glacier (Kongsvegen) embedded sediment | 0.037 | ||
114 | Surface glacier (Kongsvegen) embedded sediment | 0.011 | ||
115 | Surface glacier (Kongsvegen) embedded sediment | 0.061 | ||
116 | Surface glacier (Kongsvegen) embedded sediment | 0.11 |
|
* Iceberg embedded sediment only
n/d not determined as dry mass insufficient (<0.1 g)
Table S-3 Dissolved (<0.2 µm) Fe and total dissolvable Fe for 15 surface water samples collected in Kongsfjorden (July 2015).
Name | DFe / nM | TdFe / nM | Temperature / °C | Latitude °N | Longitude °E | Salinity |
F1 | 28.5 | 7226 | 8.4 | 78.938 | 11.992 | 20.0 |
F2 | 9.5 | 1548 | 7.0 | 78.937 | 12.003 | 32.1 |
F3 | 19.6 | 1806 | 6.7 | 78.942 | 11.990 | 31.7 |
F4 | 6.6 | 12444 | 8.2 | 78.937 | 12.018 | 14.2 |
F5 | 19.7 | 1175 | 7.5 | 78.931 | 11.957 | 31.7 |
F6 | 47.1 | 5463 | 8.8 | 78.928 | 11.926 | 22.6 |
F7 | 15.5 | 52495 | 2.6 | 78.880 | 12.528 | 26.9 |
F8 | 10.3 | 4492 | 3.7 | 78.893 | 12.518 | 28.1 |
F9 | 4.6 | 3425 | 4.1 | 78.903 | 12.493 | 28.6 |
F10 | 4.5 | 5126 | 4.9 | 78.911 | 12.518 | 23.1 |
F11 | 60.1 | 15558 | 4.8 | 78.917 | 12.570 | 23.1 |
F12 | 6.8 | 3408 | 4.8 | 78.917 | 12.476 | 26.7 |
F13 | 27.8 | 3691 | 5.0 | 78.919 | 12.419 | 27.6 |
F14 | 6.9 | 2600 | 4.6 | 78.924 | 12.330 | 29.8 |
F15 | 5.6 | 1605 | 5.4 | 78.929 | 12.223 | 29.7 |
Table S-4 Dissolved (<0.2 µm) Fe and total dissolvable Fe for 28 randomly collected Kongsfjorden iceberg samples (July 2015).
Sample label | Origin | Catchment | DFe / nM | TdFe / nM | PFe (TdFe-DFe) / nM |
Ice 1 | Ny Alesund, Svalbard, Summer 2015 | Kongsfjorden | 19.2 | 261 | 242 |
Ice 2 | Ny Alesund, Svalbard, Summer 2015 | Kongsfjorden | 9.5 | 97 | 87 |
Ice 3 | Ny Alesund, Svalbard, Summer 2015 | Kongsfjorden | 1.3 | 369 | 367 |
Ice 4 | Ny Alesund, Svalbard, Summer 2015 | Kongsfjorden | 7.9 | 1013 | 1005 |
Ice 5 | Ny Alesund, Svalbard, Summer 2015 | Kongsfjorden | 4.9 | 331 | 326 |
Ice 6 | Ny Alesund, Svalbard, Summer 2015 | Kongsfjorden | 0.6 | 133 | 133 |
Ice 7 | Ny Alesund, Svalbard, Summer 2015 | Kongsfjorden | 3.2 | 377 | 374 |
Ice 8 | Ny Alesund, Svalbard, Summer 2015 | Kongsfjorden | 2.0 | 109 | 107 |
Ice 9 | Ny Alesund, Svalbard, Summer 2015 | Kongsfjorden | 2.1 | 54 | 52 |
Ice 10 | Ny Alesund, Svalbard, Summer 2015 | Kongsfjorden | 6.4 | 897 | 890 |
Ice 11 | Ny Alesund, Svalbard, Summer 2015 | Kongsfjorden | 16.5 | 2274 | 2257 |
Ice 12 | Ny Alesund, Svalbard, Summer 2015 | Kongsfjorden | 6.6 | 470 | 464 |
Ice 13 | Ny Alesund, Svalbard, Summer 2015 | Kongsfjorden | 9.7 | 23259 | 23250 |
Ice 14 | Ny Alesund, Svalbard, Summer 2015 | Kongsfjorden | 8.0 | 221 | 213 |
Ice 15 | Ny Alesund, Svalbard, Summer 2015 | Kongsfjorden | 3.7 | 49 | 45 |
Ice 16 | Ny Alesund, Svalbard, Summer 2015 | Kongsfjorden | 3.9 | 59 | 55 |
Ice 17 | Ny Alesund, Svalbard, Summer 2015 | Kongsfjorden | 11.1 | 62 | 51 |
Ice 18 | Ny Alesund, Svalbard, Summer 2015 | Kongsfjorden | 9.5 | 46 | 37 |
Ice 19 | Ny Alesund, Svalbard, Summer 2015 | Kongsfjorden | 12.4 | 65 | 53 |
Ice 20 | Ny Alesund, Svalbard, Summer 2015 | Kongsfjorden | 5.4 | 79 | 73 |
Ice 21 | Ny Alesund, Svalbard, Summer 2015 | Kongsfjorden | 536.3 | 57401 | 56864 |
Ice 22 | Ny Alesund, Svalbard, Summer 2015 | Kongsfjorden | 54.7 | 77 | 22 |
Ice 23 | Ny Alesund, Svalbard, Summer 2015 | Kongsfjorden | 4.1 | 156 | 151 |
Ice 24 | Ny Alesund, Svalbard, Summer 2015 | Kongsfjorden | 1.4 | 99 | 97 |
Ice 25 | Ny Alesund, Svalbard, Summer 2015 | Kongsfjorden | 1.4 | 107 | 106 |
Ice 26 | Ny Alesund, Svalbard, Summer 2015 | Kongsfjorden | 3.4 | 88 | 84 |
Ice 27 | Ny Alesund, Svalbard, Summer 2015 | Kongsfjorden | 16.5 | 830 | 814 |
Ice 28 | Ny Alesund, Svalbard, Summer 2015 | Kongsfjorden | 266.1 | 10876 | 10610 |
Supplementary Information References
Raiswell, R., Vu, H.P., Brinza, L., Benning, L.G. (2010) The determination of labile Fe in ferrihydrite by ascorbic acid extraction: Methodology, dissolution kinetics and loss of solubility with age and de-watering. Chemical Geology 278, 70–79.
Stookey, L.L. (1970) Ferrozine- a new spectrophotometric reagent for iron. Analytical Chemistry 42, 779–781.
Figures and Tables

Figure 1 Surface fjord sample locations in Kongsfjorden.
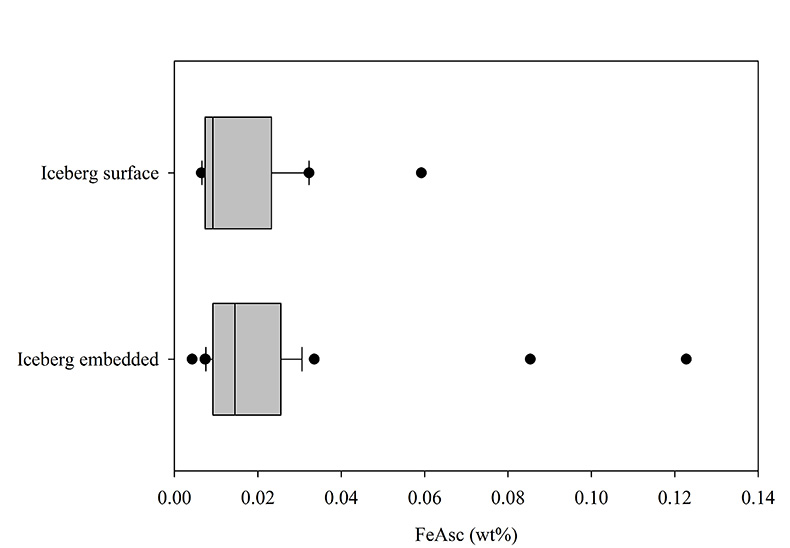
Figure 2 Median FeAsc (wt. %) with 25/75th (boxes) and 10/90th (whiskers) percentiles (outliers also shown) for iceberg embedded (n = 34) and iceberg surface (n = 20) sediment.
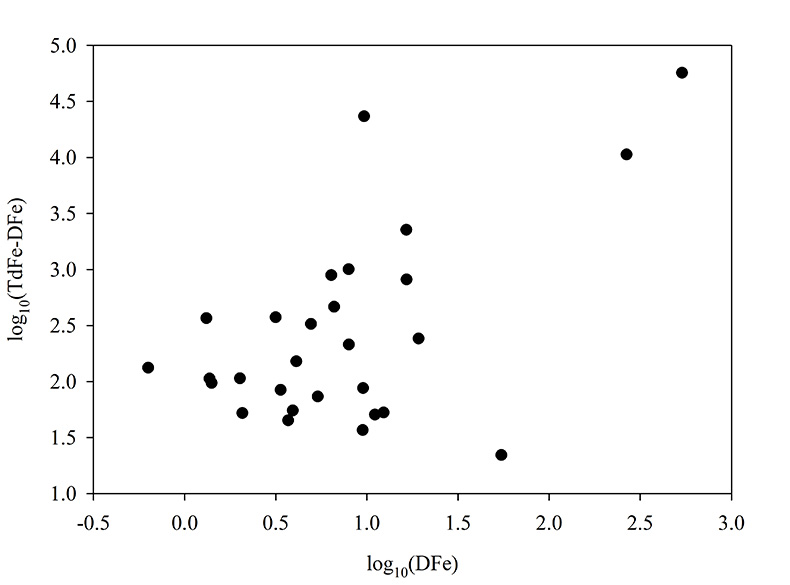
Figure 3 DFe and TdFe (both nM, plotted as log10, TdFe shown minus DFe) for 28 discrete iceberg samples showed no clear relationship.
Table 1 Comparing data for Kongsfjorden from this and prior work suggests a critical difference in both FeAsc (wt. %) and in the scaling of FeAsc to iceberg sediment load (g L-1 of ice melt).*The suggested 0.5 g L-1 sediment loading is used for data from Raiswell et al. (2016)
Raiswell, R., Hawkings, J.R., Benning, L.G., Baker, A.R., Death, R., Albani, S., Mahowald, N., Krom, M.D., Poulton, S.W., Wadham, J., Tranter, M. (2016) Potentially bioavailable iron delivery by iceberg-hosted sediments and atmospheric dust to the polar oceans. Biogeosciences 13, 3887–3900.
.** For our study, measured sediment loadings were used for each sample. As sediment-rich ice was specifically targeted, the calculated mean/median should be over-estimates.
| a This study |
b Raiswell et al. (2016) Raiswell, R., Hawkings, J.R., Benning, L.G., Baker, A.R., Death, R., Albani, S., Mahowald, N., Krom, M.D., Poulton, S.W., Wadham, J., Tranter, M. (2016) Potentially bioavailable iron delivery by iceberg-hosted sediments and atmospheric dust to the polar oceans. Biogeosciences 13, 3887–3900. | a/b % | |
FeAsc / wt. % | Mean | 0,021 | 0,14 | 16 |
Median | 0,015 | 0,092 | 17 | |
FeAsc / µM (per litre of ice melt)* | Mean | <59 ** | 12 | 480 |
| Median | <2.5 ** | 8,2 | 31 |
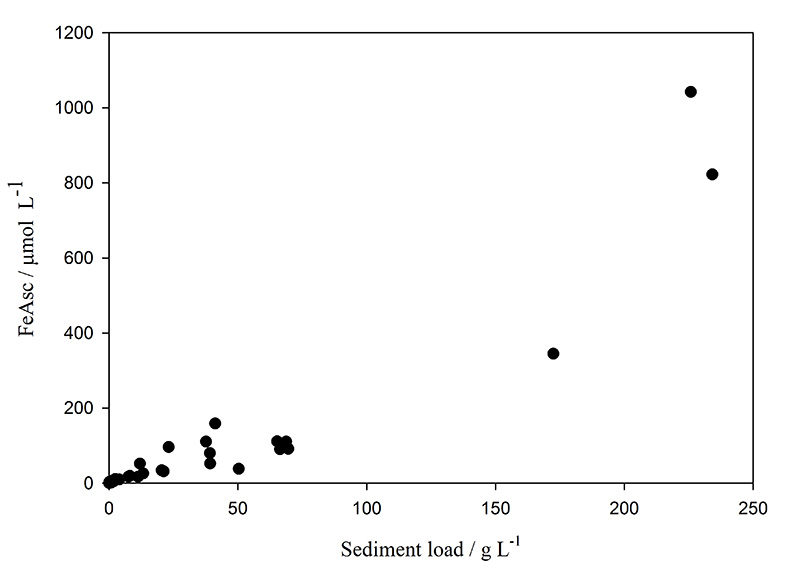
Figure 4 FeAsc (µmol L-1 melted ice) increased with sediment load (g L-1 melted ice), but it is unclear if the relationship remains linear at high (>50 g L-1) loadings.
Back to article
Supplementary Figures and Tables
Table S-1 Analysis of Certified Reference Materials for Fe concentration (± standard deviation of at least 6 measurements).
Certified Reference Material | Fe (± SD) / nM | Certified Fe concentration / nM |
NASS-7 | 6.21 (± 0.77) | 6.29 (± 0.47) |
CASS-6 | 26.6 (± 0.71) | 27.9 (± 2.1) |
Table S-2 FeAsc concentration reported for various sediment samples collected around Kongsfjorden. For iceberg embedded sediment with sediment loadings >0.1 g L-1, FeAsc is reported both as wt. % and per volume of meltwater.
Sample number | Origin | FeAsc wt. % | *FeAsc µmol / L | *Sediment loading g / L |
1 | Embedded sediment , glacier crevasse (Midtre Lovénbreen) | 0.014 | ||
2 | Embedded sediment , glacier crevasse (Midtre Lovénbreen) | 0.0057 | ||
3 | Embedded sediment , glacier crevasse (Midtre Lovénbreen) | 0.17 | ||
4 | Embedded sediment , glacier crevasse (Midtre Lovénbreen) | 0.023 | ||
5 | Embedded sediment , glacier crevasse (Midtre Lovénbreen) | 0.0038 | ||
6 | Embedded sediment , glacier crevasse (Midtre Lovénbreen) | 0.053 | ||
7 | Glacier surface sediment (Kongsvegen) | 0.016 | ||
8 | Glacier surface sediment (Kongsvegen) | 0.016 | ||
9 | Glacier surface sediment (Kongsvegen) | 0.012 | ||
10 | Glacier surface sediment (Kongsvegen) | 0.010 | ||
11 | Glacier surface sediment (Kongsvegen) | 0.016 | ||
12 | Glacier surface sediment (Kongsvegen) | 0.012 | ||
13 | Glacier surface sediment (Kongsvegen) | 0.014 | ||
14 | Iceberg embedded sediment | 0.009 | 110 | 69 |
15 | Iceberg embedded sediment | 0.0093 | 34 | 20 |
16 | Iceberg embedded sediment | 0.0076 | 90 | 66 |
17 | Iceberg embedded sediment | 0.0074 | 52 | 39 |
18 | Iceberg embedded sediment | 0.0085 | 17 | 11 |
19 | Iceberg embedded sediment | 0.016 | 2.5 | 0.91 |
20 | Iceberg embedded sediment | 0.0089 | 1.2 | 0.76 |
21 | Iceberg embedded sediment | 0.085 | 3.9 | 0.25 |
22 | Iceberg embedded sediment | 0.014 | 9.6 | 3.9 |
23 | Iceberg embedded sediment | 0.020 | 820 | 230 |
24 | Iceberg embedded sediment | 0.034 | 3.9 | 0.65 |
25 | Iceberg embedded sediment | 0.011 | 26 | 13 |
26 | Iceberg embedded sediment | 0.026 | 1000 | 230 |
27 | Iceberg embedded sediment | 0.023 | 96 | 23 |
28 | Iceberg embedded sediment | 0.0042 | 38 | 50 |
29 | Iceberg embedded sediment | 0.022 | 160 | 41 |
30 | Iceberg embedded sediment | 0.016 | 110 | 38 |
31 | Iceberg embedded sediment | 0.0082 | 31 | 21 |
32 | Iceberg embedded sediment | 0.024 | 52 | 12 |
33 | Iceberg embedded sediment | 0.0095 | 110 | 65 |
34 | Iceberg embedded sediment | 0.0073 | 91 | 70 |
35 | Iceberg embedded sediment | 0.015 | 5.0 | 1.8 |
36 | Iceberg embedded sediment | 0.013 | 17 | 7.5 |
37 | Iceberg embedded sediment | 0.011 | 340 | 170 |
38 | Iceberg embedded sediment | 0.027 | 7.8 | 1.6 |
39 | Iceberg embedded sediment | 0.026 | 1.2 | 0.25 |
40 | Iceberg embedded sediment | 0.025 | 11 | 2.4 |
41 | Iceberg embedded sediment | 0.12 | 3.0 | 0.14 |
42 | Iceberg embedded sediment | 0.028 | 2.5 | 0.51 |
43 | Iceberg embedded sediment | 0.013 | 19 | 8.1 |
44 | Iceberg embedded sediment | 0.011 | 80 | 39 |
45 | Iceberg embedded sediment | 0.020 | 3.6 | 0.99 |
46 | Iceberg embedded sediment | 0.026 | 1.2 | 0.26 |
47 | Iceberg embedded sediment | 0.012 | 2.1 | 1.0 |
48 | Iceberg embedded sediment | n/d | 0.26 | < 0.1 |
49 | Iceberg embedded sediment | n/d | 0.26 | < 0.1 |
50 | Iceberg embedded sediment | n/d | 0.87 | < 0.1 |
51 | Iceberg embedded sediment | n/d | 0.65 | < 0.1 |
52 | Iceberg embedded sediment | n/d | 0.27 | < 0.1 |
53 | Iceberg embedded sediment | n/d | 0.50 | < 0.1 |
54 | Iceberg embedded sediment | n/d | 0.28 | < 0.1 |
55 | Iceberg embedded sediment | n/d | 0.63 | < 0.1 |
56 | Iceberg embedded sediment | n/d | 0.40 | < 0.1 |
57 | Iceberg embedded sediment | n/d | 0.69 | < 0.1 |
58 | Iceberg embedded sediment | n/d | 0.37 | < 0.1 |
59 | Iceberg embedded sediment | n/d | 0.43 | < 0.1 |
60 | Iceberg embedded sediment | n/d | 0.68 | < 0.1 |
61 | Iceberg embedded sediment | n/d | 0.87 | < 0.1 |
62 | Iceberg embedded sediment | n/d | 0.27 | < 0.1 |
63 | Iceberg embedded sediment | n/d | 0.39 | < 0.1 |
64 | Iceberg embedded sediment | n/d | 1.8 | < 0.1 |
65 | Iceberg embedded sediment | n/d | 0.40 | < 0.1 |
66 | Iceberg embedded sediment | n/d | 0.77 | < 0.1 |
67 | Iceberg embedded sediment | n/d | 0.35 | < 0.1 |
68 | Iceberg embedded sediment | n/d | 0.29 | < 0.1 |
69 | Iceberg embedded sediment | n/d | 0.32 | < 0.1 |
70 | Iceberg embedded sediment | n/d | 0.20 | < 0.1 |
71 | Iceberg embedded sediment | n/d | 0.15 | < 0.1 |
72 | Iceberg surface sediment | 0.0065 | ||
73 | Iceberg surface sediment | 0.0068 | ||
74 | Iceberg surface sediment | 0.0091 | ||
75 | Iceberg surface sediment | 0.0072 | ||
76 | Iceberg surface sediment | 0.024 | ||
77 | Iceberg surface sediment | 0.0071 | ||
78 | Iceberg surface sediment | 0.032 | ||
79 | Iceberg surface sediment | 0.010 | ||
80 | Iceberg surface sediment | 0.012 | ||
81 | Iceberg surface sediment | 0.021 | ||
82 | Iceberg surface sediment | 0.0093 | ||
83 | Iceberg surface sediment | 0.0077 | ||
84 | Iceberg surface sediment | 0.059 | ||
85 | Iceberg surface sediment | 0.0066 | ||
86 | Iceberg surface sediment | 0.0080 | ||
87 | Iceberg surface sediment | 0.0080 | ||
88 | Iceberg surface sediment | 0.029 | ||
89 | Iceberg surface sediment | 0.032 | ||
90 | Iceberg surface sediment | 0.0087 | ||
91 | Iceberg surface sediment | 0.0098 | ||
92 | Pro-glacial stream, glacial flour | 0.0090 | ||
93 | Pro-glacial stream, glacial flour | 0.0083 | ||
94 | Pro-glacial stream, glacial flour | 0.0063 | ||
95 | Pro-glacial stream, glacial flour | 0.0079 | ||
96 | Pro-glacial stream, glacial flour | 0.0072 | ||
97 | Pro-glacial stream, glacial flour | 0.0070 | ||
98 | Pro-glacial stream, glacial flour | 0.0073 | ||
99 | Pro-glacial stream, glacial flour | 0.019 | ||
100 | Pro-glacial stream, glacial flour | 0.031 | ||
101 | Pro-glacial stream, glacial flour | 0.010 | ||
102 | Pro-glacial stream, glacial flour | 0.0078 | ||
103 | Pro-glacial stream, glacial flour | 0.010 | ||
104 | Pro-glacial stream, glacial flour | 0.0066 | ||
105 | Pro-glacial stream, glacial flour | 0.0089 | ||
106 | Pro-glacial stream, glacial flour | 0.0096 | ||
107 | Pro-glacial stream, glacial flour | 0.0041 | ||
108 | Pro-glacial stream, glacial flour | 0.0032 | ||
109 | Pro-glacial stream, glacial flour | 0.0057 | ||
110 | Pro-glacial stream, glacial flour | 0.0079 | ||
111 | Surface glacier (Kongsvegen) embedded sediment | 0.044 | ||
112 | Surface glacier (Kongsvegen) embedded sediment | 0.071 | ||
113 | Surface glacier (Kongsvegen) embedded sediment | 0.037 | ||
114 | Surface glacier (Kongsvegen) embedded sediment | 0.011 | ||
115 | Surface glacier (Kongsvegen) embedded sediment | 0.061 | ||
116 | Surface glacier (Kongsvegen) embedded sediment | 0.11 |
* Iceberg embedded sediment only
n/d not determined as dry mass insufficient (<0.1 g)
Table S-3 Dissolved (<0.2 µm) Fe and total dissolvable Fe for 15 surface water samples collected in Kongsfjorden (July 2015).
Name | DFe / nM | TdFe / nM | Temperature / °C | Latitude °N | Longitude °E | Salinity |
F1 | 28.5 | 7226 | 8.4 | 78.938 | 11.992 | 20.0 |
F2 | 9.5 | 1548 | 7.0 | 78.937 | 12.003 | 32.1 |
F3 | 19.6 | 1806 | 6.7 | 78.942 | 11.990 | 31.7 |
F4 | 6.6 | 12444 | 8.2 | 78.937 | 12.018 | 14.2 |
F5 | 19.7 | 1175 | 7.5 | 78.931 | 11.957 | 31.7 |
F6 | 47.1 | 5463 | 8.8 | 78.928 | 11.926 | 22.6 |
F7 | 15.5 | 52495 | 2.6 | 78.880 | 12.528 | 26.9 |
F8 | 10.3 | 4492 | 3.7 | 78.893 | 12.518 | 28.1 |
F9 | 4.6 | 3425 | 4.1 | 78.903 | 12.493 | 28.6 |
F10 | 4.5 | 5126 | 4.9 | 78.911 | 12.518 | 23.1 |
F11 | 60.1 | 15558 | 4.8 | 78.917 | 12.570 | 23.1 |
F12 | 6.8 | 3408 | 4.8 | 78.917 | 12.476 | 26.7 |
F13 | 27.8 | 3691 | 5.0 | 78.919 | 12.419 | 27.6 |
F14 | 6.9 | 2600 | 4.6 | 78.924 | 12.330 | 29.8 |
F15 | 5.6 | 1605 | 5.4 | 78.929 | 12.223 | 29.7 |
Table S-4 Dissolved (<0.2 µm) Fe and total dissolvable Fe for 28 randomly collected Kongsfjorden iceberg samples (July 2015).
Sample label | Origin | Catchment | DFe / nM | TdFe / nM | PFe (TdFe-DFe) / nM |
Ice 1 | Ny Alesund, Svalbard, Summer 2015 | Kongsfjorden | 19.2 | 261 | 242 |
Ice 2 | Ny Alesund, Svalbard, Summer 2015 | Kongsfjorden | 9.5 | 97 | 87 |
Ice 3 | Ny Alesund, Svalbard, Summer 2015 | Kongsfjorden | 1.3 | 369 | 367 |
Ice 4 | Ny Alesund, Svalbard, Summer 2015 | Kongsfjorden | 7.9 | 1013 | 1005 |
Ice 5 | Ny Alesund, Svalbard, Summer 2015 | Kongsfjorden | 4.9 | 331 | 326 |
Ice 6 | Ny Alesund, Svalbard, Summer 2015 | Kongsfjorden | 0.6 | 133 | 133 |
Ice 7 | Ny Alesund, Svalbard, Summer 2015 | Kongsfjorden | 3.2 | 377 | 374 |
Ice 8 | Ny Alesund, Svalbard, Summer 2015 | Kongsfjorden | 2.0 | 109 | 107 |
Ice 9 | Ny Alesund, Svalbard, Summer 2015 | Kongsfjorden | 2.1 | 54 | 52 |
Ice 10 | Ny Alesund, Svalbard, Summer 2015 | Kongsfjorden | 6.4 | 897 | 890 |
Ice 11 | Ny Alesund, Svalbard, Summer 2015 | Kongsfjorden | 16.5 | 2274 | 2257 |
Ice 12 | Ny Alesund, Svalbard, Summer 2015 | Kongsfjorden | 6.6 | 470 | 464 |
Ice 13 | Ny Alesund, Svalbard, Summer 2015 | Kongsfjorden | 9.7 | 23259 | 23250 |
Ice 14 | Ny Alesund, Svalbard, Summer 2015 | Kongsfjorden | 8.0 | 221 | 213 |
Ice 15 | Ny Alesund, Svalbard, Summer 2015 | Kongsfjorden | 3.7 | 49 | 45 |
Ice 16 | Ny Alesund, Svalbard, Summer 2015 | Kongsfjorden | 3.9 | 59 | 55 |
Ice 17 | Ny Alesund, Svalbard, Summer 2015 | Kongsfjorden | 11.1 | 62 | 51 |
Ice 18 | Ny Alesund, Svalbard, Summer 2015 | Kongsfjorden | 9.5 | 46 | 37 |
Ice 19 | Ny Alesund, Svalbard, Summer 2015 | Kongsfjorden | 12.4 | 65 | 53 |
Ice 20 | Ny Alesund, Svalbard, Summer 2015 | Kongsfjorden | 5.4 | 79 | 73 |
Ice 21 | Ny Alesund, Svalbard, Summer 2015 | Kongsfjorden | 536.3 | 57401 | 56864 |
Ice 22 | Ny Alesund, Svalbard, Summer 2015 | Kongsfjorden | 54.7 | 77 | 22 |
Ice 23 | Ny Alesund, Svalbard, Summer 2015 | Kongsfjorden | 4.1 | 156 | 151 |
Ice 24 | Ny Alesund, Svalbard, Summer 2015 | Kongsfjorden | 1.4 | 99 | 97 |
Ice 25 | Ny Alesund, Svalbard, Summer 2015 | Kongsfjorden | 1.4 | 107 | 106 |
Ice 26 | Ny Alesund, Svalbard, Summer 2015 | Kongsfjorden | 3.4 | 88 | 84 |
Ice 27 | Ny Alesund, Svalbard, Summer 2015 | Kongsfjorden | 16.5 | 830 | 814 |
Ice 28 | Ny Alesund, Svalbard, Summer 2015 | Kongsfjorden | 266.1 | 10876 | 10610 |