The effect of core segregation on the Cu and Zn isotope composition of the silicate Moon
Affiliations | Corresponding Author | Cite as | Funding information- Share this article
Article views:2,424Cumulative count of HTML views and PDF downloads.
- Download Citation
- Rights & Permissions
top
Abstract

Figures and Tables
![]() Figure 1 Half-mass condensation temperatures (Lodders, 2003) for a solar system composition gas versus isotope composition. Data reference for the isotopic compositions are detailed in the Supplementary Information. It should be noted, however, that the condensation temperatures displayed are for deposition from gas to solid in a nebula gas, not for evaporation from a liquid at the more oxidising conditions pertinent to Moon formation. | ![]() Figure 2 (a,b) Zn and Cu isotope fractionation factors between liquid metal-silicate and sulfide-silicate as a function of temperature. Both increasing temperature and Ni content of sulfide decrease the Zn and Cu isotope fractionation, with the result that low Ni sulfides all exhibit a temperature dependent excess of isotopically light Cu and Zn. The pale-red band is the 95 % confidence interval for the regression shown. | ![]() Figure 3 The Cu isotope evolution of the silicate Moon during lunar sulfide segregation. Assuming the Moon is derived from the precursor BSE, and the Moon’s core is predominantly FeS (equating to a bulk Moon of ~7500 ppm S), or just the outer core (3500 ppm S) and sulfide sequestration occurs by Rayleigh fractionation, the silicate Moon becomes progressively lighter in Cu. Horizontal lines represent values of the BSM estimated by lunar basalts (red) and the BSE (green) (Herzog et al., 2009). The pale blue band represents 2 standard deviations of the error on the mean of the fractionation regression. | ![]() Figure 4 Cu is hosted in lunar sulfides as a consequence of its significantly higher preference for the sulfide phase over silicate during LMO cooling and the consequent decreasing solubility of sulfide in the melt. Zn, however, is little affected implying the BSM Zn isotopic content is set by element volatility during Moon formation and LMO degassing. The BSM’s Cu isotopic composition reflects sulfide loss to the lunar core. |
Figure 1 | Figure 2 | Figure 3 | Figure 4 |
top
Introduction
The elemental composition of the bulk silicate Earth and Moon (BSE and BSM respectively) provides insight into the accretion processes and subsequent differentiation of the Earth-Moon system. Lunar rocks and the BSE express many similarities which support a genetic link between the Earth and Moon. These include the proportions of refractory elements and the stable isotope compositions of elements with half-mass condensation temperatures (T50) higher than 1100 K, such as Si (Fitoussi and Bourdon, 2012
Fitoussi, C., Bourdon, B. (2012) Silicon isotope evidence against an enstatite chondrite Earth. Science 335, 1477-1480.
), Cr (Mougel et al., 2018Mougel, B., Moynier, F., Göpel, C. (2018) Chromium isotopic homogeneity between the Moon, the Earth, and enstatite chondrites. Earth and Planetary Science Letters 481, 1-8.
), and W (Touboul et al., 2007Touboul, M., Kleine, T., Bourdon, B., Palme, H., Wieler, R. (2007) Late formation and prolonged differentiation of the Moon inferred from W isotopes in lunar metals. Nature 450, 1206-1209.
). The Moon does, however, display a notable elemental depletion in the moderately volatile elements, such as Zn, Rb, Ga, K, and Cu, coupled with an enrichment of their heavy isotopes with respect to the BSE (Fig. 1). Although this condensation sequence is for deposition from a reducing nebula gas to solid phases, evaporation from melt at the more oxidising conditions of lunar formation results in Zn and Cu exhibiting similar volatilities (Norris and Wood, 2017Norris, C.A., Wood, B.J. (2017) Earth’s volatile contents established by melting and vaporization. Nature 549, 507.
). making explanations of their lunar isotopic variability problematic.
Figure 1 Half-mass condensation temperatures (Lodders, 2003
Lodders, K. (2003) Solar System Abundances and Condensation Temperatures of The Elements. Astrophysical Journal 591, 1220-1247.
) for a solar system composition gas versus isotope composition. Data reference for the isotopic compositions are detailed in the Supplementary Information. It should be noted, however, that the condensation temperatures displayed are for deposition from gas to solid in a nebula gas, not for evaporation from a liquid at the more oxidising conditions pertinent to Moon formation.These features have been interpreted as resulting from a global scale evaporation event associated with either the giant impact (Wang and Jacobsen, 2016
Wang, K., Jacobsen, S.B. (2016) Potassium isotopic evidence for a high-energy giant impact origin of the Moon. Nature 538, 487-490.
), and/or the differentiation of the Lunar Magma Ocean (LMO) (Day and Moynier, 2014Day, J.M., Moynier, F. (2014) Evaporative fractionation of volatile stable isotopes and their bearing on the origin of the Moon. Philosophical Transactions of the Royal Society A 372, 20130259.
; Kato et al., 2015Kato, C., Moynier, F., Valdes, M.C., Dhaliwal, J.K., Day, J.M.D. (2015) Extensive volatile loss during formation and differentiation of the Moon. Nature Communications 6, 7617.
) and localised degassing of the mare basalt lavas (Sharp et al., 2010Sharp, Z., Shearer, C., Barnes, J. (2010) The chlorine isotope composition of the Moon. 41st Lunar and Planetary Science Conference, Abstract 2424.
). Cu and Zn, however, exhibit larger isotopic variations between mare basalts and the BSE (1.07 ± 0.34 ‰ for Zn, 0.43 ± 0.14 ‰ for Cu) than other volatile isotopes of comparable condensation temperatures (e.g., 0.18 ± 0.2 1 ‰ for Rb, 0.28 ± 0.25 ‰ for Ga, 0.40 ± 0.06 ‰ for K) (Fig. 1). Although element volatility during lunar formation and degassing of the LMO undoubtedly played a role in setting the Moon’s elemental and isotopic budget, the disparity between the isotopic and elemental abundances and element condensation temperatures remains enigmatic, suggesting that more than one mechanism may be responsible. We propose that this disparity arises from the segregation of sulfides to the lunar core, exacerbated by the cooling LMO and the consequent decrease in sulfide solubility in silicate melts.Zinc and copper exhibit both siderophile and chalcophile behaviours, evidenced both by metal-silicate-sulfide partitioning experiments (Siebert et al., 2011
Siebert, J., Corgne, A., Ryerson, F.J. (2011) Systematics of metal–silicate partitioning for many siderophile elements applied to Earth’s core formation. Geochimica et Cosmochimica Acta 75, 1451-1489.
; Mahan et al., 2017Mahan, B., Siebert, J., Pringle, E.A., Moynier, F. (2017) Elemental partitioning and isotopic fractionation of Zn between metal and silicate and geochemical estimation of the S content of the Earth’s core. Geochimica et Cosmochimica Acta 196, 252-270.
), and by enrichment in meteorite hosted sulfides (e.g., Williams and Archer, 2011Williams, H.M., Archer, C. (2011) Copper stable isotopes as tracers of metal–sulphide segregation and fractional crystallisation processes on iron meteorite parent bodies. Geochimica et Cosmochimica Acta 75, 3166-3178.
). Geochemical and geophysical studies support the existence of a lunar core exhibiting an extant liquid outer component (Weber et al., 2011Weber, R.C., Lin, P.Y., Garnero, E.J., Williams, Q., Lognonne, P. (2011) Seismic detection of the lunar core. Science 331, 309-312.
), and hence the presence of a significant light element core component. Segregation of a metal-rich core from the LMO during lunar core-mantle differentiation, and subsequent mantle cooling and sulfide precipitation (Mavrogenes and O'Neill, 1999Mavrogenes, J.A., O'Neill, H.S.C. (1999) The relative effects of pressure, temperature and oxygen fugacity on the solubility of sulfide in mafic magmas. Geochimica et Cosmochimica Acta 63, 1173-1180.
), can sequester significant amounts of Cu and, to a lesser extent, Zn, without noticeable influence on lithophile volatiles such as Rb, K, Li, and Ga (Siebert et al., 2011Siebert, J., Corgne, A., Ryerson, F.J. (2011) Systematics of metal–silicate partitioning for many siderophile elements applied to Earth’s core formation. Geochimica et Cosmochimica Acta 75, 1451-1489.
). Given significant Cu isotope fractionation among metallic, silicate, and sulfide melts (Savage et al., 2015Savage, P.S., Moynier, F., Chen, H., Shofner, G., Siebert, J., Badro, J., Puchtel, I. (2015) Copper isotope evidence for large-scale sulphide fractionation during Earth’s differentiation. Geochemical Perspectives Letters 1, 53-64
), this process will inevitably fractionate lunar Cu isotopes, and, potentially, Zn isotopes. The role of core segregation in shaping the isotope composition of the silicate Moon is, as yet, unclear because equilibrium isotope fractionation factors of Zn and Cu between phases associated with lunar core formation (metal, sulfide, and silicate), remain poorly constrained. Hence, we experimentally explored the equilibrium Zn and Cu isotope fractionation among metallic, sulfide, and silicate melts at conditions relevant to lunar core formation.top
Zinc and Copper Isotope Experiments to Simulate LMO Differentiation
We conducted a series of experiments in graphite capsules at 1.5 GPa using piston cylinder apparatus (Tables S-1 to S-4, Fig. S-1 and Methods, see Supplementary Information). The experimental charges contained approximately 50 % silicate of a basaltic composition, equilibrated with ~50 % metal or sulfide with variable Ni content, under fully molten conditions (Tables S-1 to S-5 and Fig. S-1).
For both Cu and Zn isotopes, the iron-rich liquids are slightly enriched in heavier isotopes relative to the silicate melts. In contrast, sulfide melts exhibit demonstrably lighter isotope compositions than the silicate (Fig. 2), with measured Cu isotope fractionation factors consistent with both previous studies of iron meteorites (Williams and Archer, 2011
Williams, H.M., Archer, C. (2011) Copper stable isotopes as tracers of metal–sulphide segregation and fractional crystallisation processes on iron meteorite parent bodies. Geochimica et Cosmochimica Acta 75, 3166-3178.
) and experimentally determined Cu isotope fractionation for metal/silicate and sulfide/silicate liquids (Savage et al., 2015Savage, P.S., Moynier, F., Chen, H., Shofner, G., Siebert, J., Badro, J., Puchtel, I. (2015) Copper isotope evidence for large-scale sulphide fractionation during Earth’s differentiation. Geochemical Perspectives Letters 1, 53-64
) (Table S-6). Zn isotopes display a smaller degree of fractionation than Cu isotopes (Fig. 2). Overall, the isotope fractionation of both elements decreases with increasing temperature. The nickel sulfide content affects the fractionation of both Cu and Zn isotopes (Fig. 2), with high Ni (~25 wt. % Ni in sulfide) experiments exhibiting less fractionation than those with low Ni (0.1 to 1.2 wt. % Ni in sulfide, Table S-1).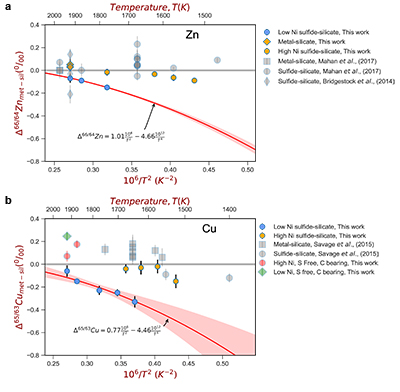
Figure 2 (a,b) Zn and Cu isotope fractionation factors between liquid metal-silicate and sulfide-silicate as a function of temperature. Both increasing temperature and Ni content of sulfide decrease the Zn and Cu isotope fractionation, with the result that low Ni sulfides all exhibit a temperature dependent excess of isotopically light Cu and Zn. The pale-red band is the 95 % confidence interval for the regression shown.
top
Discussion
During lunar core-mantle differentiation, a carbon-bearing, iron-rich core will preferentially sequester isotopically heavy Cu and, potentially Zn, leading to the BSM exhibiting lower (lighter) values in δ66Zn and δ65Cu. This is contrary to observations; the BSM is isotopically heavier than the BSE in both Cu and Zn. Importantly this precludes carbon as a significant light element component of the lunar core. In contrast, sequestration of sulfide to the lunar core would leave the BSM isotopically heavy in both Cu and, potentially Zn, in accordance with the observed offset between silicate Moon and Earth (Fig. 1). It has been proposed that the proto-Moon initially accreted from the outermost volatile-rich disk generated by the giant impact, and is thus less volatile-depleted than the observed lunar rocks from the surface (Canup et al., 2015
Canup, R.M., Visscher, C., Salmon, J., Fegley Jr, B. (2015) Lunar volatile depletion due to incomplete accretion within an impact-generated disk. Nature Geoscience 8, 918-921.
). Although subsequent degassing of the LMO would result in a significant loss of volatiles from the BSM, its extent would have been mitigated by the presence of an early-formed lunar crust (Elkins-Tanton and Grove, 2011Elkins-Tanton, L., Grove, T. (2011) Water (hydrogen) in the lunar mantle: Results from petrology and magma ocean modeling. Earth and Planetary Science Letters 307, 173-179.
). The lunar interior may therefore have retained abundant volatiles, including sulfur, through inefficient degassing, as evidenced by the comparable sulfur content of lunar magmas and terrestrial mid-ocean ridge basalts (Hauri et al., 2017Hauri, E.H., Saal, A.E., Nakajima, M., Anand, M., Rutherford, M.J., Orman, J.A.V., Voyer, M.L. (2017) Origin and Evolution of Water in the Moon's Interior. Annual Review of Earth and Planetary Sciences 45, 89-111.
). In comparison to analogous terrestrial basalts, the solubility of sulfide in the melt decreases as the BSM undergoes secular cooling, exacerbated by the lunar mantle’s lower oxygen fugacity (O’Neill and Mavrogenes, 2002O’Neill, H.S.C., Mavrogenes, J.A. (2002) The sulfide capacity and the sulfur content at sulfide saturation of silicate melts at 1400 C and 1 bar. Journal of Petrology 43, 1049-1087.
), promoting the formation of an immiscible sulfide liquid.To explore this mechanism and its effect on Zn and Cu isotopic signatures, we calculated the δ66/64Zn and δ65/63Cu content of the BSM by considering the scenario of sulfide segregation from an ambient basaltic magma ocean and assuming the bulk Moon is predominantly comprised of material derived from the Earth’s mantle (Canup, 2012
Canup, R.M. (2012) Forming a Moon with an Earth-like Composition via a Giant Impact. Science 338, 1052-1055.
; Wade and Wood, 2016Wade, J., Wood, B.J. (2016) The oxidation state and mass of the Moon-forming impactor. Earth and Planetary Science Letters 442, 186-193.
).Under the conditions of lunar sulfide sequestration, Cu, unlike Zn, exhibits a strong preference for the sulfide phase; the lunar Cu sulfide/silicate partition coefficient, DCu-MoonSulfide/Silicate, ranges from 100 to 200, in contrast to Zn, which is ~1 (Kiseeva and Wood, 2015
Kiseeva, E.S., Wood, B.J. (2015) The effects of composition and temperature on chalcophile and lithophile element partitioning into magmatic sulphides. Earth and Planetary Science Letters 424, 280-294.
). This results in a sulfide liquid, initially in equilibrium with the silicate LMO prior to its sequestration to the lunar core, enriched in isotopically light Cu. This leads to a BSM exhibiting a significant depletion in Cu and becoming isotopically heavy in Cu. The result is a divergence of Cu isotopes between the BSE and BSM, with a negligible effect on isotopes of lithophile elements (e.g., Rb, Ga, and K). Zn, however, exhibits negligible elemental and isotopic fractionation upon lunar sulfide extraction; a consequence of both its lower isotopic fractionation and chalcophile behaviour. The limited Zn isotope fractionation that occurs during the evolution of mafic magma (Chen et al., 2013Chen, H., Savage, P.S., Teng, F.-Z., Helz, R.T., Moynier, F. (2013) Zinc isotope fractionation during magmatic differentiation and the isotopic composition of the bulk Earth. Earth and Planetary Science Letters 369, 34-42.
), coupled with the uniform δ66/64Zn in high Ti (1.50 ± 0.54 ‰, n = 17) and low Ti lunar basalts (1.35 ± 0.43 ‰, n = 17), suggest their Zn isotope compositions reflect that of the primitive lunar magmas (Kato et al., 2015Kato, C., Moynier, F., Valdes, M.C., Dhaliwal, J.K., Day, J.M.D. (2015) Extensive volatile loss during formation and differentiation of the Moon. Nature Communications 6, 7617.
). During terrestrial mantle melting, high degrees of melt extraction (>30 %) may fractionate Zn isotopes up to 0.16 ‰ (Doucet et al., 2016Doucet, L.S., Mattielli, N., Ionov, D.A., Debouge, W., Golovin, A.V. (2016) Zn isotopic heterogeneity in the mantle: A melting control? Earth and Planetary Science Letters 451, 232-240.
). The significantly lower melt extraction (~5-11 %) expressed by the mare basalts (Day and Walker, 2015Day, J.M., Walker, R.J. (2015) Highly siderophile element depletion in the Moon. Earth and Planetary Science Letters 423, 114-124.
) will consequently yield only subtle isotopic variations in Zn, negligible relative to the large offset between the BSE and lunar rocks.Modelling sulfide segregation by Rayleigh fractionation reveals that sulfur-rich lunar core formation cannot be responsible for the isotopically heavy δ66/64Zn BSM, nor for its elemental depletion. Given the limited mineralogical host phases for Zn, the most likely mechanism of lunar Zn depletion is that of volatility during lunar formation (Paniello et al., 2012
Paniello, R.C., Day, J.M., Moynier, F. (2012) Zinc isotopic evidence for the origin of the Moon. Nature 490, 376-379.
) or magma ocean degassing (Kato et al., 2015Kato, C., Moynier, F., Valdes, M.C., Dhaliwal, J.K., Day, J.M.D. (2015) Extensive volatile loss during formation and differentiation of the Moon. Nature Communications 6, 7617.
). In contrast, the silicate Moon’s high δ65/63Cu can be explained as a direct consequence of sulfide sequestration to the core (Fig. 3), a result of the initially molten Moon saturated in FeS and exacerbated by the subsequent drop in the sulfide content of lunar magmas on cooling (Mavrogenes and O'Neill, 1999Mavrogenes, J.A., O'Neill, H.S.C. (1999) The relative effects of pressure, temperature and oxygen fugacity on the solubility of sulfide in mafic magmas. Geochimica et Cosmochimica Acta 63, 1173-1180.
). In addition, sulfide sequestration depletes the silicate Moon in the chalcophile elements Cu and Ni (Walter et al., 2000Walter, M.J., Newsom, H.E., Ertel, W., Holzeid, A. (2000) Siderophile elements in the Earth and Moon: metal/silicate partitioning and implications for core formation. In: Canup, R.M., Righter, K. (Eds.) Origin of the Earth and Moon. University of Arizona Press, Tucson, 265-289.
), without significantly disturbing the W isotopic age of the Moon (Wade and Wood, 2016Wade, J., Wood, B.J. (2016) The oxidation state and mass of the Moon-forming impactor. Earth and Planetary Science Letters 442, 186-193.
), an element that displays little affinity for the sulfide phase.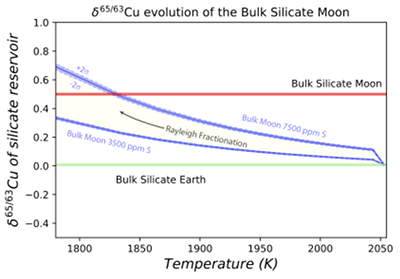
Figure 3 The Cu isotope evolution of the silicate Moon during lunar sulfide segregation. Assuming the Moon is derived from the precursor BSE, and the Moon’s core is predominantly FeS (equating to a bulk Moon of ~7500 ppm S), or just the outer core (3500 ppm S) and sulfide sequestration occurs by Rayleigh fractionation, the silicate Moon becomes progressively lighter in Cu. Horizontal lines represent values of the BSM estimated by lunar basalts (red) and the BSE (green) (Herzog et al., 2009
Herzog, G., Moynier, F., Albarède, F., Berezhnoy, A. (2009) Isotopic and elemental abundances of copper and zinc in lunar samples, Zagami, Pele’s hairs, and a terrestrial basalt. Geochimica et Cosmochimica Acta 73, 5884-5904.
). The pale blue band represents 2 standard deviations of the error on the mean of the fractionation regression.Sulfide retained in the deep Moon may therefore be an important reservoir for isotopically light Cu, but not Zn, which is best explained by its volatility during the Moon forming impact and subsequent degassing from the Lunar crust (Fig. 4.). The silicate Earth and Moon share almost identical Cr isotope compositions (Mougel et al., 2018
Mougel, B., Moynier, F., Göpel, C. (2018) Chromium isotopic homogeneity between the Moon, the Earth, and enstatite chondrites. Earth and Planetary Science Letters 481, 1-8.
), an element marginally more volatile than Fe (1296 K and 1334 K half-mass condensation temperatures respectively (Lodders, 2003Lodders, K. (2003) Solar System Abundances and Condensation Temperatures of The Elements. Astrophysical Journal 591, 1220-1247.
)), but which exhibits a similar sulfide/melt partitioning behaviour to Zn (Kiseeva and Wood, 2015Kiseeva, E.S., Wood, B.J. (2015) The effects of composition and temperature on chalcophile and lithophile element partitioning into magmatic sulphides. Earth and Planetary Science Letters 424, 280-294.
). Therefore, the segregation of sulfide (Brenan and Mungall, 2017Brenan, J.M., Mungall, J.E. (2017) A sulfide-saturated lunar mantle? 2017 EGU General Assembly, Geophysical Research Abstracts 19, EGU2017-6838.
) can drive the silicate Moon to Cu isotopic compositions, but this process has little effect on elements which display a lower preference for lunar sulfides, such as Zn and Cr. Compared to the BSE, the silicate Moon exhibits around a threefold depletion in Ni (Walter et al., 2000Walter, M.J., Newsom, H.E., Ertel, W., Holzeid, A. (2000) Siderophile elements in the Earth and Moon: metal/silicate partitioning and implications for core formation. In: Canup, R.M., Righter, K. (Eds.) Origin of the Earth and Moon. University of Arizona Press, Tucson, 265-289.
), an observation consistent with its extraction during lunar core formation via sulfide segregation (Wade and Wood, 2016Wade, J., Wood, B.J. (2016) The oxidation state and mass of the Moon-forming impactor. Earth and Planetary Science Letters 442, 186-193.
). Assuming the Moon’s Ni content is inherited primarily from the silicate Earth, this suggests the Moon’s core contains around 7.5 wt. % Ni. Increasing metallic Ni contents implies higher core sulfur contents for a given Cu isotope content exhibited by the silicate Moon.
Figure 4 Cu is hosted in lunar sulfides as a consequence of its significantly higher preference for the sulfide phase over silicate during LMO cooling and the consequent decreasing solubility of sulfide in the melt. Zn, however, is little affected implying the BSM Zn isotopic content is set by element volatility during Moon formation and LMO degassing. The BSM’s Cu isotopic composition reflects sulfide loss to the lunar core.
Sulfide saturation may arise from the secular cooling of a LMO containing excess sulfide derived from the Moon forming impactor, and/or the late addition of S to the lunar mantle. Because of the higher density of sulfide relative to the ambient magma, and the initially completely molten lunar mantle, excess or precipitating sulfide will sink into the lunar interior. The near-chondritic 187Os/188Os and chondrite-relative HSE abundance in high MgO mare basalts (Day and Walker, 2015
Day, J.M., Walker, R.J. (2015) Highly siderophile element depletion in the Moon. Earth and Planetary Science Letters 423, 114-124.
) implies that this sulfide component is efficiently extracted from the silicate Moon to the Lunar core, with little stranded in the source region of the Mare basalts (Brenan and Mungall, 2017Brenan, J.M., Mungall, J.E. (2017) A sulfide-saturated lunar mantle? 2017 EGU General Assembly, Geophysical Research Abstracts 19, EGU2017-6838.
). This addition of sulfide liquid to lunar core is in line with the studies that propose sulfur as a dominant light element in the lunar core (Rai and van Westrenen, 2014Rai, N., van Westrenen, W. (2014) Lunar core formation: New constraints from metal–silicate partitioning of siderophile elements. Earth and Planetary Science Letters 388, 343-352.
).top
Acknowledgements
This work is supported by the Strategic Priority Research Program (B) of Chinese Academy of Sciences (Grant No. XDB18000000), the National Science Foundation of China (41325011, 41630206). ESK was supported by NERC grant NE/L010828/1. JW acknowledges receipt of a NERC Independent Research Fellowship NE/K009540/1. We thank Huimin Yu and Xingchao Zhang for the help of Zn and Cu isotope analyses.
Editor: Helen Williams
top
Author Contributions
FH and ESK conceived the experimental study. ESK performed the high pressure experiments. ESK and YX conducted the electron probe measurement. YX and FH processed the samples and performed the SEM, LA-ICP-MS, and MC-ICP-MS analyses. JW performed EPMA/SEM analysis on the C-bearing samples, conceived the application, performed the data modelling and presentation. All authors contributed to interpretation of the data and writing the paper.
top
References
Brenan, J.M., Mungall, J.E. (2017) A sulfide-saturated lunar mantle? 2017 EGU General Assembly, Geophysical Research Abstracts 19, EGU2017-6838.

Therefore, the segregation of sulfide (Brenan and Mungall, 2017) can drive the silicate Moon to Cu isotopic compositions, but this process has little effect on elements which display a lower preference for lunar sulfides, such as Zn and Cr.
View in article
The near-chondritic 187Os/188Os and chondrite-relative HSE abundance in high MgO mare basalts (Day and Walker, 2015) implies that this sulfide component is efficiently extracted from the silicate Moon to the Lunar core, with little stranded in the source region of the Mare basalts (Brenan and Mungall, 2017).
View in article
Bridgestock, L.J., Williams, H., Rehkämper, M., Larner, F., Giscard, M.D., Hammond, S., Coles, B., Andreasen, R., Wood, B.J., Theis, K.J., Smith, C.L., Benedix, G.K., Schönbächler, M. (2014) Unlocking the zinc isotope systematics of iron meteorites. Earth and Planetary Science Letters 400, 153-164.

Figure 2
View in article
Canup, R.M. (2012) Forming a Moon with an Earth-like Composition via a Giant Impact. Science 338, 1052-1055.

To explore this mechanism and its effect on Zn and Cu isotopic signatures, we calculated the δ66/64Zn and δ65/63Cu content of the BSM by considering the scenario of sulfide segregation from an ambient basaltic magma ocean and assuming the bulk Moon is predominantly comprised of material derived from the Earth’s mantle (Canup, 2012; Wade and Wood, 2016).
View in article
Canup, R.M., Visscher, C., Salmon, J., Fegley Jr, B. (2015) Lunar volatile depletion due to incomplete accretion within an impact-generated disk. Nature Geoscience 8, 918-921.

It has been proposed that the proto-Moon initially accreted from the outermost volatile-rich disk generated by the giant impact, and is thus less volatile-depleted than the observed lunar rocks from the surface (Canup et al., 2015).
View in article
Chen, H., Savage, P.S., Teng, F.-Z., Helz, R.T., Moynier, F. (2013) Zinc isotope fractionation during magmatic differentiation and the isotopic composition of the bulk Earth. Earth and Planetary Science Letters 369, 34-42.

The limited Zn isotope fractionation that occurs during the evolution of mafic magma (Chen et al., 2013), coupled with the uniform δ66/64Zn in high Ti (1.50 ± 0.54 ‰, n = 17) and low Ti lunar basalts (1.35 ± 0.43 ‰, n = 17), suggest their Zn isotope compositions reflect that of the primitive lunar magmas (Kato et al., 2015).
View in article
Day, J.M., Moynier, F. (2014) Evaporative fractionation of volatile stable isotopes and their bearing on the origin of the Moon. Philosophical Transactions of the Royal Society A 372, 20130259.

These features have been interpreted as resulting from a global scale evaporation event associated with either the giant impact (Wang and Jacobsen, 2016), and/or the differentiation of the Lunar Magma Ocean (LMO) (Day and Moynier, 2014; Kato et al., 2015) and localised degassing of the mare basalt lavas (Sharp et al., 2010).
View in article
Day, J.M., Walker, R.J. (2015) Highly siderophile element depletion in the Moon. Earth and Planetary Science Letters 423, 114-124.

The significantly lower melt extraction (~5-11 %) expressed by the mare basalts (Day and Walker, 2015) will consequently yield only subtle isotopic variations in Zn, negligible relative to the large offset between the BSE and lunar rocks.
View in article
The near-chondritic 187Os/188Os and chondrite-relative HSE abundance in high MgO mare basalts (Day and Walker, 2015) implies that this sulfide component is efficiently extracted from the silicate Moon to the Lunar core, with little stranded in the source region of the Mare basalts (Brenan and Mungall, 2017).
View in article
Doucet, L.S., Mattielli, N., Ionov, D.A., Debouge, W., Golovin, A.V. (2016) Zn isotopic heterogeneity in the mantle: A melting control? Earth and Planetary Science Letters 451, 232-240.

During terrestrial mantle melting, high degrees of melt extraction (>30 %) may fractionate Zn isotopes up to 0.16 ‰ (Doucet et al., 2016).
View in article
Elkins-Tanton, L., Grove, T. (2011) Water (hydrogen) in the lunar mantle: Results from petrology and magma ocean modeling. Earth and Planetary Science Letters 307, 173-179.

Although subsequent degassing of the LMO would result in a significant loss of volatiles from the BSM, its extent would have been mitigated by the presence of an early-formed lunar crust (Elkins-Tanton and Grove, 2011).
View in article
Fitoussi, C., Bourdon, B. (2012) Silicon isotope evidence against an enstatite chondrite Earth. Science 335, 1477-1480.

These include the proportions of refractory elements and the stable isotope compositions of elements with half-mass condensation temperatures (T50) higher than 1100 K, such as Si (Fitoussi and Bourdon, 2012), Cr (Mougel et al., 2018), and W (Touboul et al., 2007).
View in article
Hauri, E.H., Saal, A.E., Nakajima, M., Anand, M., Rutherford, M.J., Orman, J.A.V., Voyer, M.L. (2017) Origin and Evolution of Water in the Moon's Interior. Annual Review of Earth and Planetary Sciences 45, 89-111.

The lunar interior may therefore have retained abundant volatiles, including sulfur, through inefficient degassing, as evidenced by the comparable sulfur content of lunar magmas and terrestrial mid-ocean ridge basalts (Hauri et al., 2017).
View in article
Herzog, G., Moynier, F., Albarède, F., Berezhnoy, A. (2009) Isotopic and elemental abundances of copper and zinc in lunar samples, Zagami, Pele’s hairs, and a terrestrial basalt. Geochimica et Cosmochimica Acta 73, 5884-5904.

Figure 3 [...] Horizontal lines represent values of the BSM estimated by lunar basalts (red) and the BSE (green) (Herzog et al., 2009).
View in article
Kato, C., Moynier, F., Valdes, M.C., Dhaliwal, J.K., Day, J.M.D. (2015) Extensive volatile loss during formation and differentiation of the Moon. Nature Communications 6, 7617.

These features have been interpreted as resulting from a global scale evaporation event associated with either the giant impact (Wang and Jacobsen, 2016), and/or the differentiation of the Lunar Magma Ocean (LMO) (Day and Moynier, 2014; Kato et al., 2015) and localised degassing of the mare basalt lavas (Sharp et al., 2010).
View in article
The limited Zn isotope fractionation that occurs during the evolution of mafic magma (Chen et al., 2013), coupled with the uniform δ66/64Zn in high Ti (1.50 ± 0.54 ‰, n = 17) and low Ti lunar basalts (1.35 ± 0.43 ‰, n = 17), suggest their Zn isotope compositions reflect that of the primitive lunar magmas (Kato et al., 2015).
View in article
Given the limited mineralogical host phases for Zn, the most likely mechanism of lunar Zn depletion is that of volatility during lunar formation (Paniello et al., 2012) or magma ocean degassing (Kato et al., 2015).
View in article
Kiseeva, E.S., Wood, B.J. (2015) The effects of composition and temperature on chalcophile and lithophile element partitioning into magmatic sulphides. Earth and Planetary Science Letters 424, 280-294.

Under the conditions of lunar sulfide sequestration, Cu, unlike Zn, exhibits a strong preference for the sulfide phase; the lunar Cu sulfide/silicate partition coefficient, DCu-MoonSulfide/Silicate, ranges from 100 to 200, in contrast to Zn, which is ~1 (Kiseeva and Wood, 2015).
View in article
The silicate Earth and Moon share almost identical Cr isotope compositions (Mougel et al., 2018), an element marginally more volatile than Fe (1296 K and 1334 K half-mass condensation temperatures respectively (Lodders, 2003)), but which exhibits a similar sulfide/melt partitioning behaviour to Zn (Kiseeva and Wood, 2015).
View in article
Lodders, K. (2003) Solar System Abundances and Condensation Temperatures of The Elements. Astrophysical Journal 591, 1220-1247.

Figure 1 Half-mass condensation temperatures (Lodders, 2003) for a solar system composition gas versus isotope composition.
View in article
The silicate Earth and Moon share almost identical Cr isotope compositions (Mougel et al., 2018), an element marginally more volatile than Fe (1296 K and 1334 K half-mass condensation temperatures respectively (Lodders, 2003)), but which exhibits a similar sulfide/melt partitioning behaviour to Zn (Kiseeva and Wood, 2015).
View in article
Mahan, B., Siebert, J., Pringle, E.A., Moynier, F. (2017) Elemental partitioning and isotopic fractionation of Zn between metal and silicate and geochemical estimation of the S content of the Earth’s core. Geochimica et Cosmochimica Acta 196, 252-270.

Zinc and copper exhibit both siderophile and chalcophile behaviours, evidenced both by metal-silicate-sulfide partitioning experiments (Siebert et al., 2011; Mahan et al., 2017), and by enrichment in meteorite hosted sulfides (e.g., Williams and Archer, 2011).
View in article
Figure 2
View in article
Mavrogenes, J.A., O'Neill, H.S.C. (1999) The relative effects of pressure, temperature and oxygen fugacity on the solubility of sulfide in mafic magmas. Geochimica Et Cosmochimica Acta 63, 1173-1180.

Segregation of a metal-rich core from the LMO during lunar core-mantle differentiation, and subsequent mantle cooling and sulfide precipitation (Mavrogenes and O'Neill, 1999), can sequester significant amounts of Cu and, to a lesser extent, Zn, without noticeable influence on lithophile volatiles such as Rb, K, Li, and Ga (Siebert et al., 2011).
View in article
In contrast, the silicate Moon’s high δ65/63Cu can be explained as a direct consequence of sulfide sequestration to the core (Fig. 3), a result of the initially molten Moon saturated in FeS and exacerbated by the subsequent drop in the sulfide content of lunar magmas on cooling (Mavrogenes and O'Neill, 1999).
View in article
Mougel, B., Moynier, F., Göpel, C. (2018) Chromium isotopic homogeneity between the Moon, the Earth, and enstatite chondrites. Earth and Planetary Science Letters 481, 1-8.

These include the proportions of refractory elements and the stable isotope compositions of elements with half-mass condensation temperatures (T50) higher than 1100 K, such as Si (Fitoussi and Bourdon, 2012), Cr (Mougel et al., 2018), and W (Touboul et al., 2007).
View in article
The silicate Earth and Moon share almost identical Cr isotope compositions (Mougel et al., 2018), an element marginally more volatile than Fe (1296 K and 1334 K half-mass condensation temperatures respectively (Lodders, 2003)), but which exhibits a similar sulfide/melt partitioning behaviour to Zn (Kiseeva and Wood, 2015).
View in article
Norris, C.A., Wood, B.J. (2017) Earth’s volatile contents established by melting and vaporization. Nature 549, 507.

Although this condensation sequence is for deposition from a reducing nebula gas to solid phases, evaporation from melt at the more oxidising conditions of lunar formation results in Zn and Cu exhibiting similar volatilities (Norris and Wood, 2017)
View in article
O’Neill, H.S.C., Mavrogenes, J.A. (2002) The sulfide capacity and the sulfur content at sulfide saturation of silicate melts at 1400 C and 1 bar. Journal of Petrology 43, 1049-1087.

In comparison to analogous terrestrial basalts, the solubility of sulfide in the melt decreases as the BSM undergoes secular cooling, exacerbated by the lunar mantle’s lower oxygen fugacity (O’Neill and Mavrogenes, 2002), promoting the formation of an immiscible sulfide liquid.
View in article
Paniello, R.C., Day, J.M., Moynier, F. (2012) Zinc isotopic evidence for the origin of the Moon. Nature 490, 376-379.

Given the limited mineralogical host phases for Zn, the most likely mechanism of lunar Zn depletion is that of volatility during lunar formation (Paniello et al., 2012) or magma ocean degassing (Kato et al., 2015).
View in article
Rai, N., van Westrenen, W. (2014) Lunar core formation: New constraints from metal–silicate partitioning of siderophile elements. Earth and Planetary Science Letters 388, 343-352.

This addition of sulfide liquid to lunar core is in line with the studies that propose sulfur as a dominant light element in the lunar core (Rai and van Westrenen, 2014).
View in article
Savage, P.S., Moynier, F., Chen, H., Shofner, G., Siebert, J., Badro, J., Puchtel, I. (2015) Copper isotope evidence for large-scale sulphide fractionation during Earth’s differentiation. Geochemical Perspectives Letters 1, 53-64

Given significant Cu isotope fractionation among metallic, silicate, and sulfide melts (Savage et al., 2015), this process will inevitably fractionate lunar Cu isotopes, and, potentially, Zn isotopes.
View in article
In contrast, sulfide melts exhibit demonstrably lighter isotope compositions than the silicate (Fig. 2), with measured Cu isotope fractionation factors consistent with both previous studies of iron meteorites (Williams and Archer, 2011) and experimentally determined Cu isotope fractionation for metal/silicate and sulfide/silicate liquids (Savage et al., 2015) (Table S-6).
View in article
Figure 2
View in article
Sharp, Z., Shearer, C., Barnes, J. (2010) The chlorine isotope composition of the Moon. 41st Lunar and Planetary Science Conference, Abstract 2424.

These features have been interpreted as resulting from a global scale evaporation event associated with either the giant impact (Wang and Jacobsen, 2016), and/or the differentiation of the Lunar Magma Ocean (LMO) (Day and Moynier, 2014; Kato et al., 2015) and localised degassing of the mare basalt lavas (Sharp et al., 2010).
View in article
Siebert, J., Corgne, A., Ryerson, F.J. (2011) Systematics of metal–silicate partitioning for many siderophile elements applied to Earth’s core formation. Geochimica et Cosmochimica Acta 75, 1451-1489.

Zinc and copper exhibit both siderophile and chalcophile behaviours, evidenced both by metal-silicate-sulfide partitioning experiments (Siebert et al., 2011; Mahan et al., 2017), and by enrichment in meteorite hosted sulfides (e.g., Williams and Archer, 2011).
View in article
Segregation of a metal-rich core from the LMO during lunar core-mantle differentiation, and subsequent mantle cooling and sulfide precipitation (Mavrogenes and O'Neill, 1999), can sequester significant amounts of Cu and, to a lesser extent, Zn, without noticeable influence on lithophile volatiles such as Rb, K, Li, and Ga (Siebert et al., 2011).
View in article
Touboul, M., Kleine, T., Bourdon, B., Palme, H., Wieler, R. (2007) Late formation and prolonged differentiation of the Moon inferred from W isotopes in lunar metals. Nature 450, 1206-1209.

These include the proportions of refractory elements and the stable isotope compositions of elements with half-mass condensation temperatures (T50) higher than 1100 K, such as Si (Fitoussi and Bourdon, 2012), Cr (Mougel et al., 2018), and W (Touboul et al., 2007).
View in article
Wade, J., Wood, B.J. (2016) The oxidation state and mass of the Moon-forming impactor. Earth and Planetary Science Letters 442, 186-193.

To explore this mechanism and its effect on Zn and Cu isotopic signatures, we calculated the δ66/64Zn and δ65/63Cu content of the BSM by considering the scenario of sulfide segregation from an ambient basaltic magma ocean and assuming the bulk Moon is predominantly comprised of material derived from the Earth’s mantle (Canup, 2012; Wade and Wood, 2016).
View in article
In addition, sulfide sequestration depletes the silicate Moon in the chalcophile elements Cu and Ni (Walter et al., 2000), without significantly disturbing the W isotopic age of the Moon (Wade and Wood, 2016), an element that displays little affinity for the sulfide phase.
View in article
Compared to the BSE, the silicate Moon exhibits around a threefold depletion in Ni (Walter et al., 2000), an observation consistent with its extraction during lunar core formation via sulfide segregation (Wade and Wood, 2016).
View in article
Walter, M.J., Newsom, H.E., Ertel, W., Holzeid, A. (2000) Siderophile elements in the Earth and Moon: metal/silicate partitioning and implications for core formation. In: Canup, R.M., Righter, K. (Eds.) Origin of the Earth and Moon. University of Arizona Press, Tucson, 265-289.

In addition, sulfide sequestration depletes the silicate Moon in the chalcophile elements Cu and Ni (Walter et al., 2000), without significantly disturbing the W isotopic age of the Moon (Wade and Wood, 2016), an element that displays little affinity for the sulfide phase.
View in article
Compared to the BSE, the silicate Moon exhibits around a threefold depletion in Ni (Walter et al., 2000), an observation consistent with its extraction during lunar core formation via sulfide segregation (Wade and Wood, 2016).
View in article
Wang, K., Jacobsen, S.B. (2016) Potassium isotopic evidence for a high-energy giant impact origin of the Moon. Nature 538, 487-490.

These features have been interpreted as resulting from a global scale evaporation event associated with either the giant impact (Wang and Jacobsen, 2016), and/or the differentiation of the Lunar Magma Ocean (LMO) (Day and Moynier, 2014; Kato et al., 2015) and localised degassing of the mare basalt lavas (Sharp et al., 2010).
View in article
Weber, R.C., Lin, P.Y., Garnero, E.J., Williams, Q., Lognonne, P. (2011) Seismic detection of the lunar core. Science 331, 309-312.

Geochemical and geophysical studies support the existence of a lunar core exhibiting an extant liquid outer component (Weber et al., 2011), and hence the presence of a significant light element core component.
View in article
Williams, H.M., Archer, C. (2011) Copper stable isotopes as tracers of metal–sulphide segregation and fractional crystallisation processes on iron meteorite parent bodies. Geochimica et Cosmochimica Acta 75, 3166-3178.

Zinc and copper exhibit both siderophile and chalcophile behaviours, evidenced both by metal-silicate-sulfide partitioning experiments (Siebert et al., 2011; Mahan et al., 2017), and by enrichment in meteorite hosted sulfides (e.g., Williams and Archer, 2011).
View in article
In contrast, sulfide melts exhibit demonstrably lighter isotope compositions than the silicate (Fig. 2), with measured Cu isotope fractionation factors consistent with both previous studies of iron meteorites (Williams and Archer, 2011) and experimentally determined Cu isotope fractionation for metal/silicate and sulfide/silicate liquids (Savage et al., 2015) (Table S-6).
View in article
top
Supplementary Information
The Supplementary Information includes:
- Petrological Experiments
- Electron Microprobe analysis
- Laser Ablation ICPS analysis
- Isotopic Analysis
- Modelling the Effect of Sulfide Sequestration on the Isotopic Content of the Silicate Moon
- Additional Information in Support of Figure 1
- Figures S-1 to S-4
- Tables S-1 to S-6
- Supplementary Information References
Download the Supplementary Information (PDF).
Figures and Tables

Figure 1 Half-mass condensation temperatures (Lodders, 2003) for a solar system composition gas versus isotope composition. Data reference for the isotopic compositions are detailed in the Supplementary Information. It should be noted, however, that the condensation temperatures displayed are for deposition from gas to solid in a nebula gas, not for evaporation from a liquid at the more oxidising conditions pertinent to Moon formation.
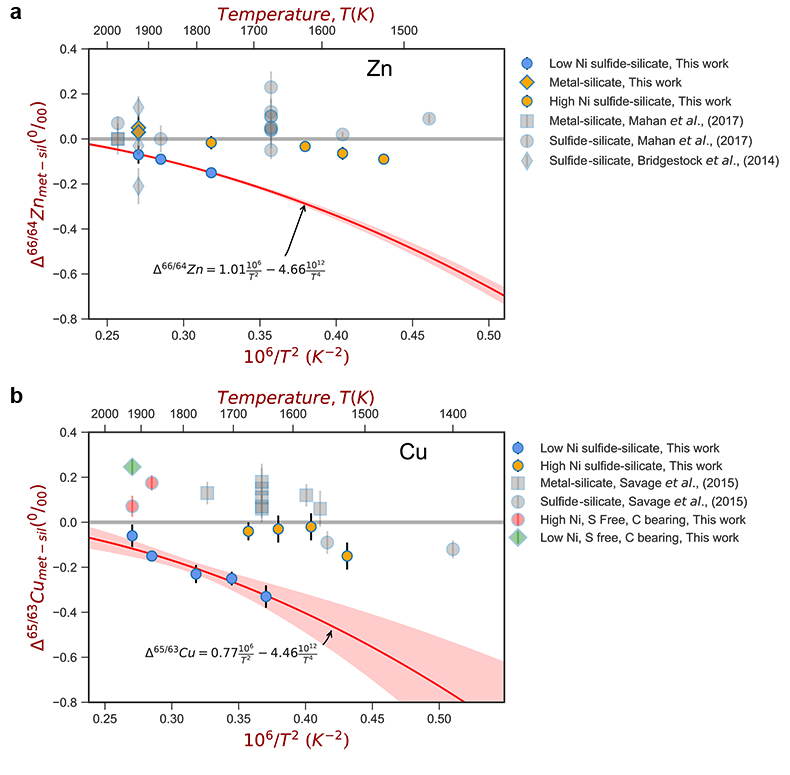
Figure 2 (a,b) Zn and Cu isotope fractionation factors between liquid metal-silicate and sulfide-silicate as a function of temperature. Both increasing temperature and Ni content of sulfide decrease the Zn and Cu isotope fractionation, with the result that low Ni sulfides all exhibit a temperature dependent excess of isotopically light Cu and Zn. The pale-red band is the 95 % confidence interval for the regression shown.

Figure 3 The Cu isotope evolution of the silicate Moon during lunar sulfide segregation. Assuming the Moon is derived from the precursor BSE, and the Moon’s core is predominantly FeS (equating to a bulk Moon of ~7500 ppm S), or just the outer core (3500 ppm S) and sulfide sequestration occurs by Rayleigh fractionation, the silicate Moon becomes progressively lighter in Cu. Horizontal lines represent values of the BSM estimated by lunar basalts (red) and the BSE (green) (Herzog et al., 2009
Herzog, G., Moynier, F., Albarède, F., Berezhnoy, A. (2009) Isotopic and elemental abundances of copper and zinc in lunar samples, Zagami, Pele’s hairs, and a terrestrial basalt. Geochimica et Cosmochimica Acta 73, 5884-5904.
). The pale blue band represents 2 standard deviations of the error on the mean of the fractionation regression.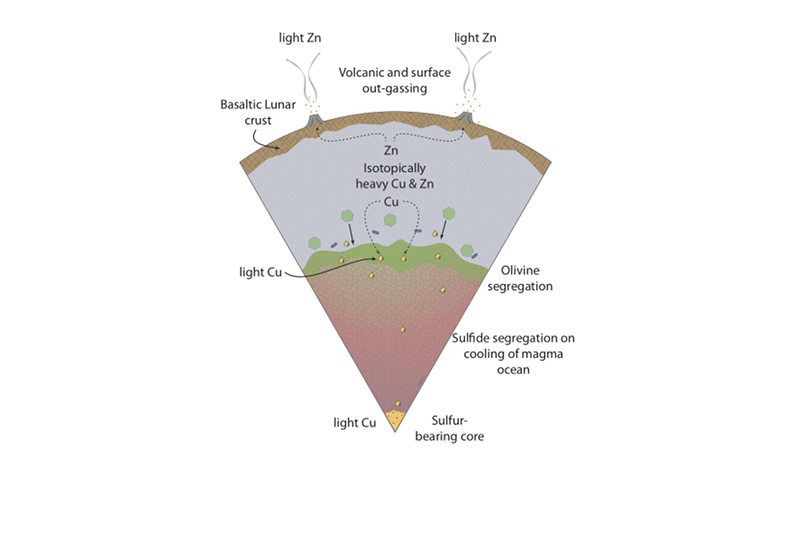
Figure 4 Cu is hosted in lunar sulfides as a consequence of its significantly higher preference for the sulfide phase over silicate during LMO cooling and the consequent decreasing solubility of sulfide in the melt. Zn, however, is little affected implying the BSM Zn isotopic content is set by element volatility during Moon formation and LMO degassing. The BSM’s Cu isotopic composition reflects sulfide loss to the lunar core.