Loss of immiscible nitrogen from metallic melt explains Earth’s missing nitrogen
Affiliations | Corresponding Author | Cite as | Funding information- Share this article
Article views:3,050Cumulative count of HTML views and PDF downloads.
- Download Citation
- Rights & Permissions
top
Abstract
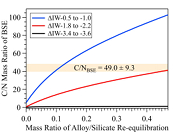
Figures and Tables
![]() Figure 1 Representative X-ray radiographic images showing the evolution of the miscibility gap in Fe-N-C system under high pressure and high temperature conditions (run 4-17). This in situ X-ray radiography experiment starts at 0.4 GPa with Fe3N as the starting material. (a) The sample is below solidus at 0.4 GPa and 1483 K. (b) Around 1729 K, immiscible N-rich supercritical fluid coexists with metallic liquid. (c) At higher temperatures, N-rich supercritical fluid partially dissolves into metallic liquid. (d) Lowering temperature re-exsolves N-rich supercritical fluid from metallic liquid. The corresponding movie is shown in Video S-1. | ![]() Figure 2 The pressure dependence of N solubility in Fe-N-C melt. The solid circles are for Fe-N starting materials; the open circles are for Fe-N-C starting materials. Symbol sizes are proportional to the temperatures (1400-2300 K) at which the experiments are quenched and colours indicate initial compositions (see legend). The blue and red curves are modelled N solubilities in Fe-N-C melt at 2000 K and 2300 K, respectively, from Speelmanns et al. (2018). | ![]() Figure 3 The evolution of the BSE C/N ratio with the degree of re-equilibration between alloy and silicate melts during core formation. The blue curve is calculated at oxidised conditions (ΔIW-0.5 to ΔIW-1.0); the red curve is for reduced conditions (ΔIW-1.8 to ΔIW-2.2) and the black curve is for very reduced conditions (ΔIW-3.4 to ΔIW-3.6). The horizontal yellow bar marks the range of the estimated present BSE C/N ratio (Bergin et al., 2015). |
Figure 1 | Figure 2 | Figure 3 |
top
Introduction
The habitability of Earth and other planetary bodies depends on the incorporation, distribution, and speciation of volatile elements including carbon and nitrogen. In Earth, the BSE C/N ratio is estimated to be higher than that of planetary building blocks including enstatite, carbonaceous chondrites, interstellar dust and gas (e.g., Marty, 2012
Marty, B. (2012) The origins and concentrations of water, carbon, nitrogen and noble gases on Earth. Earth and Planetary Science Letters 313–314, 56–66.
; Bergin et al., 2015Bergin, E.A., Blake, G.A., Ciesla, F., Hirschmann, M.M., Li, J. (2015) Tracing the ingredients for a habitable earth from interstellar space through planet formation. Proceedings of the National Academy of Sciences 112, 8965–8970.
). Recent studies estimate the BSE C/N ratio to be superchondritic based on measurements of gas bubbles trapped in mid-ocean ridge and ocean island basalts (Marty, 2012Marty, B. (2012) The origins and concentrations of water, carbon, nitrogen and noble gases on Earth. Earth and Planetary Science Letters 313–314, 56–66.
; Halliday, 2013Halliday, A.N. (2013) The origins of volatiles in the terrestrial planets. Geochimica et Cosmochimica Acta 105, 146–171.
; Bergin et al., 2015Bergin, E.A., Blake, G.A., Ciesla, F., Hirschmann, M.M., Li, J. (2015) Tracing the ingredients for a habitable earth from interstellar space through planet formation. Proceedings of the National Academy of Sciences 112, 8965–8970.
), and recent estimates of the BSE C/N ratio converge to 49 ± 9 (Bergin et al., 2015Bergin, E.A., Blake, G.A., Ciesla, F., Hirschmann, M.M., Li, J. (2015) Tracing the ingredients for a habitable earth from interstellar space through planet formation. Proceedings of the National Academy of Sciences 112, 8965–8970.
). In contrast, C/N ratios measured in primitive CI chondrites (17 ± 3; Alexander et al., 2013Alexander, C.M.O., Howard, K.T., Bowden, R., Fogel, M.L. (2013) The classification of CM and CR chondrites using bulk H, C and N abundances and isotopic compositions. Geochimica et Cosmochimica Acta 123, 244–260.
) and enstatite chondrites (14 ± 12; Grady and Wright, 2003Grady, M.M., Wright, I.P. (2003) Elemental and Isotopic Abundances of Carbon and Nitrogen in Meteorites. Space Science Reviews 106, 231–248.
) are significantly lower. Explaining these observations thus requires one or more mechanisms that preferentially deplete N relative to C from the mantle source of basalt magmas.The key processes responsible for C and N redistribution among Earth reservoirs were equilibrium partitioning between the magma ocean and the growing metallic core and degassing of the magma ocean to the early atmosphere, but these processes have been insufficient to explain the estimated high BSE C/N ratio (Bergin et al., 2015
Bergin, E.A., Blake, G.A., Ciesla, F., Hirschmann, M.M., Li, J. (2015) Tracing the ingredients for a habitable earth from interstellar space through planet formation. Proceedings of the National Academy of Sciences 112, 8965–8970.
; Hirschmann, 2016Hirschmann, M.M. (2016) Constraints on the early delivery and fractionation of Earth’s major volatiles from C/H, C/N, and C/S ratios. American Mineralogist 101, 540–553.
; Dalou et al., 2017Dalou, C., Hirschmann, M.M., von der Handt, A., Mosenfelder, J., Armstrong, L.S. (2017) Nitrogen and carbon fractionation during core–mantle differentiation at shallow depth. Earth and Planetary Science Letters 458, 141–151.
). Core formation is expected to decrease the BSE C/N ratio because at relevant conditions C is a much stronger siderophile element than N (e.g., Dalou et al., 2017Dalou, C., Hirschmann, M.M., von der Handt, A., Mosenfelder, J., Armstrong, L.S. (2017) Nitrogen and carbon fractionation during core–mantle differentiation at shallow depth. Earth and Planetary Science Letters 458, 141–151.
). Degassing has not been thought to significantly affect the C/N ratio, as chemical analysis of volatiles in silicate glasses quenched from high pressure-temperature (P-T) experiments indicates that the solubilities of C and N in the magma ocean are comparable (Hirschmann, 2016Hirschmann, M.M. (2016) Constraints on the early delivery and fractionation of Earth’s major volatiles from C/H, C/N, and C/S ratios. American Mineralogist 101, 540–553.
).The major host phase for both C and N in the solidifying planet is likely to be core-forming alloy, therefore understanding the Fe-N-C system under relevant conditions may hold the key to the paradox of the high BSE C/N ratio. While the Fe-C phase diagram has been well studied over a wide range of pressures (e.g., Fei and Brosh, 2014
Fei, Y., Brosh, E. (2014) Experimental study and thermodynamic calculations of phase relations in the Fe–C system at high pressure. Earth and Planetary Science Letters 408, 155–162.
; Liu et al., 2016Liu, J., Li, J., Hrubiak, R., Smith, J.S. (2016) Origins of ultralow velocity zones through slab-derived metallic melt. Proceedings of the National Academy of Sciences 113, 5547–5551.
), very limited data are available on the phase relations of the Fe-N system (e.g., Guillermet and Du, 1994Guillermet, A.F., Du, H. (1994) Thermodynamic Analysis of the Fe-N System Using the Compound-Energy Model with Predictions of the Vibrational Entropy. Zeitschrift Fur Metallkunde 85, 154–163.
). In particular, melting behaviours are expected to be different in these two systems, as the C-rich end member, graphite/diamond, has a melting point of 4000-5000 K at relevant pressures (Grumbach and Martin, 1996Grumbach, M.P., Martin, R.M. (1996) Phase diagram of carbon at high pressures and temperatures. Physical Review B 54, 15730–15741.
), while the N-rich end member would be gas/supercritical fluid in the BSE.top
Immiscible N-rich Fluid
In order to test whether the mobility of N-rich gas/fluid could provide a possible mechanism to leak N during accretion and core formation, we investigated melting relations in the Fe-N-C system under high pressures by in situ X-ray radiography and X-ray diffraction (XRD) coupled with Paris-Edinburgh (PE) cells and ex situ chemical analysis. The starting materials are either iron nitrides (Fe3N and Fe4N) or a mixture of iron and iron nitride powders with or without graphite powders. The starting N and C contents range from 0-7.7 wt. % and 0-10.0 wt. %, respectively (Table S-1). A standard PE sample assembly configuration was employed (Kono et al., 2014
Kono, Y., Park, C., Kenney-Benson, C., Shen, G., Wang, Y. (2014) Toward comprehensive studies of liquids at high pressures and high temperatures: Combined structure, elastic wave velocity, and viscosity measurements in the Paris–Edinburgh cell. Physics of the Earth and Planetary Interiors 228, 269–280.
), which used X-ray transparent MgO as the sample capsule and a cylindrical graphite tube outside sample capsule as the heater. Assemblages were first compressed to target pressures and gradually heated to be fully molten. Then the molten samples were quenched below 500 K within 5 seconds to preserve compositions for ex situ chemical analysis (Table S-1).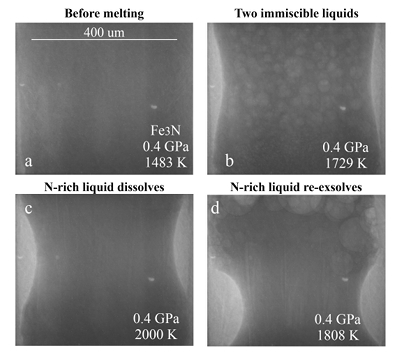
Figure 1 Representative X-ray radiographic images showing the evolution of the miscibility gap in Fe-N-C system under high pressure and high temperature conditions (run 4-17). This in situ X-ray radiography experiment starts at 0.4 GPa with Fe3N as the starting material. (a) The sample is below solidus at 0.4 GPa and 1483 K. (b) Around 1729 K, immiscible N-rich supercritical fluid coexists with metallic liquid. (c) At higher temperatures, N-rich supercritical fluid partially dissolves into metallic liquid. (d) Lowering temperature re-exsolves N-rich supercritical fluid from metallic liquid. The corresponding movie is shown in Video S-1.
Below the solidus, starting materials for all runs were homogenous at ~3 mm spatial resolution of X-ray radiographic images (e.g., Fig. 1a). An upper bound for the onset of partial melting at higher temperature was indicated by the appearance of 10s-micron regions of low X-ray absorption (e.g., Fig. S-1b). At higher temperatures, these lighter regions merged to form 10s to 100s-micron droplets which moved vigorously (Fig. 1b). XRD patterns during heating also recorded the melting process (Fig. S-1d): at 0.4 GPa and 300 K, the XRD pattern confirmed Fe3N as the starting material; at ~1690 K, most crystalline peaks disappeared with an obvious increase in background at ~34-80 keV (Fig. S-1d), which originated from diffuse scattering signal. The corresponding X-ray radiographic image (Fig. S1-b) showed the ubiquitous occurrence of the low X-ray absorption regions. Therefore, both the XRD pattern and X-ray radiographic image indicated a partially molten state of the Fe-N sample. Turbulent flow of two phases occurred above ~1725 K (Video S-1), and the corresponding XRD pattern exhibited diffuse scattering with no XRD peaks (Fig. S-1d) and thus indicated conditions above the liquidus but below the solvus. At higher temperatures, the immiscible droplets partially dissolved into the metallic liquid but persisted to the highest temperature investigated in run 4-17 (~2000 K, Fig. 1c). Lowering temperature made more immiscible droplets re-exsolve (Fig. 1d), confirming that the disappearance of the immiscible droplets from Figures 1b to 1c cannot be explained by loss of N-rich fluid through the MgO capsule. In comparison, no inhomogeneity appeared at all in Fe melting experiments at 0.8 GPa up to 2103 K (Fig. S-2).
The immiscible droplets with lighter colours in X-ray radiographic images are enriched in N because the contrast in X-ray radiographic images reflects density differences (Kono et al., 2015
Kono, Y., Kenney-Benson, C., Shibazaki, Y., Park, C., Wang, Y., Shen, G. (2015) X-ray imaging for studying behavior of liquids at high pressures and high temperatures using Paris-Edinburgh press. Review of Scientific Instruments 86, 072207.
). The N-rich nature of the immiscible fluid is confirmed by ex situ chemical analysis of the quenched samples: for experiments quenched with the presence of immiscible liquids, the regions which are lighter in X-ray radiographic images correspond to voids in recovered samples (e.g., Figs. S-3 to S-6); N contents in the recovered samples are lower than the starting values when immiscible melting occurs (Table S-1). For example, N content in run 4-16 decreases from 5.9 % in the starting materials to 1.5 % in the recovered sample (Fig. S-3). With increasing temperature, the increase of N solubility in metallic melt (e.g., Fig. 1 and Video S-1) can be explained by the concave-down shape of the solvus, which marks the phase boundary between one liquid and two immiscible liquids (Fig. S-7). A similar phenomenon has been observed in the Fe-O system (Kowalski and Spencer, 1995Kowalski, M., Spencer, P.J. (1995) Thermodynamic reevaluation of the C-O, Fe-O and Ni-O systems: Remodelling of the liquid, BCC and FCC phases. Calphad 19, 229–243.
; Tsuno et al., 2007Tsuno, K., Ohtani, E., Terasaki, H. (2007) Immiscible two-liquid regions in the Fe–O–S system at high pressure: Implications for planetary cores. Physics of the Earth and Planetary Interiors 160, 75–85.
): at 1 bar, O solubility increases from ~0 to ~5 mol. % when temperature increases from ~1811 K to ~2340 K (Kowalski and Spencer, 1995Kowalski, M., Spencer, P.J. (1995) Thermodynamic reevaluation of the C-O, Fe-O and Ni-O systems: Remodelling of the liquid, BCC and FCC phases. Calphad 19, 229–243.
). Such a miscibility gap persists to at least 21 GPa in the Fe-O system and O solubility in metallic melt increases with a rate of 0.01-0.03 mol./K between 15-21 GPa and 2050-2350 K (Tsuno et al., 2007Tsuno, K., Ohtani, E., Terasaki, H. (2007) Immiscible two-liquid regions in the Fe–O–S system at high pressure: Implications for planetary cores. Physics of the Earth and Planetary Interiors 160, 75–85.
). For experiments with C added to the starting materials, immiscible melting also occurs (Figs. S-5 and S-6). C contents in the recovered samples are the same as starting materials within uncertainty (Table S-1). This indicates that C does not dissolve into N-rich fluid during immiscible melting.The combination of in situ and ex situ analysis was employed to constrain the solubility of N in Fe-N-C alloys (Fig. 2). Near 1 bar in all compositions up to 7.7 wt. % N and 10.0 wt. % C, immiscibility of N-rich supercritical fluid and metallic melt observed in situ persists to at least 2273 K, the highest temperature investigated here. Solubility of N in Fe-N-C alloy increases from almost 0 near 1 bar to ~6.8 wt. % N at 6.3 GPa, as the highest pressure investigated here (Fig. 2). The pressure required for closing the miscibility gap increases with the starting N and C contents (Fig. 2). For Fe-1.4 wt. % N starting material at about 0-0.1 GPa, immiscible liquids persist to at least 1980 K, but no immiscible liquids appear at ~1.4 GPa up to 2000 K (Fig. S-6). In contrast, for Fe-7.7 wt. % N starting composition the solvus persists to at least 6.3 GPa (Fig. 2). Adding 5-10 wt. % C in the starting materials expands the pressure range of the solvus by about 2-5 GPa (Fig. 2). This is because C is more siderophilic than N and it expels N from metallic melt to the immiscible fluid.

Figure 2 The pressure dependence of N solubility in Fe-N-C melt. The solid circles are for Fe-N starting materials; the open circles are for Fe-N-C starting materials. Symbol sizes are proportional to the temperatures (1400-2300 K) at which the experiments are quenched and colours indicate initial compositions (see legend). The blue and red curves are modelled N solubilities in Fe-N-C melt at 2000 K and 2300 K, respectively, from Speelmanns et al. (2018)
Speelmanns, I.M., Schmidt, M.W., Liebske, C. (2018) Nitrogen Solubility in Core Materials. Geophysical Research Letters 45, 7434–7443.
.A recent study also infers the presence of N-rich fluid from Fe-N-C melt based on the ex situ analysis of quenched samples (Speelmanns et al., 2018
Speelmanns, I.M., Schmidt, M.W., Liebske, C. (2018) Nitrogen Solubility in Core Materials. Geophysical Research Letters 45, 7434–7443.
). The trend of their N solubility model is consistent with our data (Fig. 2). However, the C and Pt contaminations up to 12.5 wt. % from sample capsules are not incorporated into their N solubility models (Speelmanns et al., 2018Speelmanns, I.M., Schmidt, M.W., Liebske, C. (2018) Nitrogen Solubility in Core Materials. Geophysical Research Letters 45, 7434–7443.
), which may explain the offsets between these two studies (Fig. 2). The presence of immiscible N-rich fluid in silicate-alloy systems has been observed in a previous partitioning study on metallic-silicate melts up to 10 GPa (Roskosz et al., 2013Roskosz, M., Bouhifd, M.A., Jephcoat, A.P., Marty, B., Mysen, B.O. (2013) Nitrogen solubility in molten metal and silicate at high pressure and temperature. Geochimica et Cosmochimica Acta 121, 15–28.
), supporting that this immiscible phenomenon could occur in a realistic magma ocean phase assemblage.top
Missing N in the Mantle
The conundrum of missing N in the mantle can be solved by accounting for immiscibility of N in the Fe-N-C system in modelling Earth’s N budget. Our model calculates the BSE C/N ratio in three steps (Fig. S-8 and SI): first, we assume Earth-forming impactors have alloy core and silicate mantle in equilibrium with average alloy/silicate mass ratio of 0.46, same as the Earth’s core/mantle mass ratio. The amount of N stored in metallic core is controlled by oxygen fugacity (Dalou et al., 2017
Dalou, C., Hirschmann, M.M., von der Handt, A., Mosenfelder, J., Armstrong, L.S. (2017) Nitrogen and carbon fractionation during core–mantle differentiation at shallow depth. Earth and Planetary Science Letters 458, 141–151.
): 90.2 % N would stay in the core at oxidised bodies (ΔIW-0.5 to -1.0); this number decreases to 69.7 % and 12.1 % at modestly reduced (ΔIW-1.8 to -2.2) and more reduced bodies (ΔIW-3.4 to -3.6), respectively (Fig. S-9). In contrast, previous models assume all C and N are initially stored in silicate (Bergin et al., 2015Bergin, E.A., Blake, G.A., Ciesla, F., Hirschmann, M.M., Li, J. (2015) Tracing the ingredients for a habitable earth from interstellar space through planet formation. Proceedings of the National Academy of Sciences 112, 8965–8970.
; Hirschmann, 2016Hirschmann, M.M. (2016) Constraints on the early delivery and fractionation of Earth’s major volatiles from C/H, C/N, and C/S ratios. American Mineralogist 101, 540–553.
; Dalou et al., 2017Dalou, C., Hirschmann, M.M., von der Handt, A., Mosenfelder, J., Armstrong, L.S. (2017) Nitrogen and carbon fractionation during core–mantle differentiation at shallow depth. Earth and Planetary Science Letters 458, 141–151.
). This assumption sets the initial BSE C/N ratio at 25 without loss of the primordial atmosphere, which is about 20-24 higher than our initial BSE C/N ratios (Fig. 3). Secondly, we assume that extensive melting of alloy during high energy impacts induces immiscible melting, resulting in loss of all N stored in impactors’ cores to space. In general, the fraction of N released from an impactor depends on the core/mantle mass ratios in the accreting bodies: the degree of melting during accretion/melting, the extent of immiscibility between N-rich supercritical fluid and metallic melt, and the fraction of atmospheric N loss to space. As our model assumes impactor cores melt completely and release N at the magma ocean surface, it yields an upper limit for N loss at this stage. The loss of N results in an extremely high C/N ratio in the metallic phase. Once such N-depleted metallic droplets sink below the depth where solubility of N in alloy increases (Fig. S-8), N could partition from the magma ocean into N-depleted alloy droplets and therefore elevate the BSE C/N ratio as the alloy joins Earth’s growing core.Overall, the loss of N from impactor cores could significantly lower Earth’s bulk N budget and therefore raise the BSE C/N ratio (Fig. 3). Because N partitions more strongly into alloy under more oxidised conditions, more N is subject to loss from oxidised accreting bodies (Fig. S-9). Similarly, the subsequent core formation would also result in a higher C/N ratio of the BSE under oxidised conditions (Fig. 3). Over time during core formation, fO2 is estimated to evolve from lower values of ΔIW-3.9 to ΔIW-1.9 to higher values of ΔIW-1.9 to ΔIW-1.0 (Rubie et al., 2011
Rubie, D.C., Frost, D.J., Mann, U., Asahara, Y., Nimmo, F., Tsuno, K., Kegler, P., Holzheid, A., Palme, H. (2011) Heterogeneous accretion, composition and core–mantle differentiation of the Earth. Earth and Planetary Science Letters 301, 31–42.
, Badro et al., 2015Badro, J., Brodholt, J.P., Piet, H., Siebert, J., Ryerson, F.J. (2015) Core formation and core composition from coupled geochemical and geophysical constraints. Proceedings of the National Academy of Sciences 112, 12310–12314.
). Therefore, the BSE C/N ratio is expected to evolve from 0.03-0.5 at very reduced conditions to 0.7-41.4 at reduced conditions and finally to 5-130.0 at slightly reduced conditions (Fig. 3). Compared with previous models (Bergin et al., 2015Bergin, E.A., Blake, G.A., Ciesla, F., Hirschmann, M.M., Li, J. (2015) Tracing the ingredients for a habitable earth from interstellar space through planet formation. Proceedings of the National Academy of Sciences 112, 8965–8970.
), our model matches the estimated BSE C/N ratio for an extensive range of redox conditions, the degree of alloy-silicate re-equilibration and extent of loss of nascent atmosphere during accretion and core formation. Notably, both C and N form accessory minerals, such as diamonds, iron carbides and iron/titanium/chromium nitrides (e.g., Fegley, 1983Fegley, B. (1983) Primordial retention of nitrogen by terrestrial planets and meteorites. Journal of Geophysical Research: Solid Earth 88, A853–A868.
; Javoy, 1997Javoy, M. (1997) The major volatile elements of the Earth: Their origin, behavior, and fate. Geophysical Research Letters 24, 177–180.
; Kaminsky and Wirth, 2017Kaminsky, F., Wirth, R. (2017) Nitrides and carbonitrides from the lowermost mantle and their importance in the search for Earth’s “lost” nitrogen. American Mineralogist 102, 1667–1676.
), which have not been considered in the estimated BSE C/N ratio yet. In addition, the presence of deep mantle carbonates (e.g., Brenker et al., 2007Brenker, F.E., Vollmer, C., Vincze, L., Vekemans, B., Szymanski, A., Janssens, K., Szaloki, I., Nasdala, L., Joswig, W., Kaminsky, F. (2007) Carbonates from the lower part of transition zone or even the lower mantle. Earth and Planetary Science Letters 260, 1–9.
) and high solubilities (10s to 1000s mg/g) of N in transition zone and lower mantle minerals (Yoshioka et al., 2018Yoshioka, T., Wiedenbeck, M., Shcheka, S., Keppler, H. (2018) Nitrogen solubility in the deep mantle and the origin of Earth’s primordial nitrogen budget. Earth and Planetary Science Letters 488, 134–143.
), both of which are sensitive to redox and pH conditions (e.g., Rohrbach and Schmidt, 2011Rohrbach, A., Schmidt, M.W. (2011) Redox freezing and melting in the Earth’s deep mantle resulting from carbon-iron redox coupling. Nature 472, 209–212.
; Mikhail et al., 2017Mikhail, S., Barry, P.H., Sverjensky, D.A. (2017) The relationship between mantle pH and the deep nitrogen cycle. Geochimica et Cosmochimica Acta 209, 149–160.
) , would also influence the estimation of the BSE C/N ratio.Vapourisation during accretion has recently been evoked to explain Mg, Si and Fe isotopic compositions in the BSE (Hin et al., 2017
Hin, R.C., Coath, C.D., Carter, P.J., Nimmo, F., Lai, Y.-J., Pogge von Strandmann, P.A.E., Willbold, M., Leinhardt, Z.M., Walter, M.J., Elliott, T. (2017) Magnesium isotope evidence that accretional vapour loss shapes planetary compositions. Nature 549, 511–515.
) and the pattern of volatile element depletion (Norris and Wood, 2017Norris, C.A., Wood, B.J. (2017) Earth’s volatile contents established by melting and vaporization. Nature 549, 507–510.
), indicating its critical role in the early stage of Earth evolution. Considering the high C/N ratios (>10) of most planetary bodies (Bergin et al., 2015Bergin, E.A., Blake, G.A., Ciesla, F., Hirschmann, M.M., Li, J. (2015) Tracing the ingredients for a habitable earth from interstellar space through planet formation. Proceedings of the National Academy of Sciences 112, 8965–8970.
), the C/N ratios of the impactors’ cores would be even higher and therefore promote immiscible melting during impact. In addition, for Earth-like planets at the proposed synestia stage (Lock and Stewart, 2017Lock, S.J., Stewart, S.T. (2017) The structure of terrestrial bodies: Impact heating, corotation limits, and synestias. Journal of Geophysical Research: Planets 122, 2016JE005239.
), the magma ocean is surrounded by massive vapourised materials, which are at pressures between 0.1 bar and 1.0 GPa. In this stage, the loss of N through immiscible fluid could significantly shape the BSE C/N ratio.
Figure 3 The evolution of the BSE C/N ratio with the degree of re-equilibration between alloy and silicate melts during core formation. The blue curve is calculated at oxidised conditions (ΔIW-0.5 to ΔIW-1.0); the red curve is for reduced conditions (ΔIW-1.8 to ΔIW-2.2) and the black curve is for very reduced conditions (ΔIW-3.4 to ΔIW-3.6). The horizontal yellow bar marks the range of the estimated present BSE C/N ratio (Bergin et al., 2015
Bergin, E.A., Blake, G.A., Ciesla, F., Hirschmann, M.M., Li, J. (2015) Tracing the ingredients for a habitable earth from interstellar space through planet formation. Proceedings of the National Academy of Sciences 112, 8965–8970.
).top
Acknowledgements
S.M. Dorfman acknowledges funding from Michigan State University, the Sloan Foundation’s Deep Carbon Observatory Grant G-2017-9954, and NSF EAR-1751664. J. Li acknowledges partial support from NSF AST 1344133, NASA NNX15AG54G, and Sloan Foundation Deep Carbon Observatory Grant G-2017-9954.
Editor: Simon Redfern
top
Author Contributions
J. Liu and S.M. Dorfman designed research; J.C. Liu, S.M. Dorfman, M. Lv, J. Li, F. Zhu, Y. Kono performed experiments; J. Liu analysed the data and built the model; J. Liu and S.M. Dorfman wrote the paper.
top
References
Alexander, C.M.O., Howard, K.T., Bowden, R., Fogel, M.L. (2013) The classification of CM and CR chondrites using bulk H, C and N abundances and isotopic compositions. Geochimica et Cosmochimica Acta 123, 244–260.

In contrast, C/N ratios measured in primitive CI chondrites (17 ± 3; Alexander et al., 2013) and enstatite chondrites (14 ± 12; Grady and Wright, 2003) are significantly lower.
View in article
Badro, J., Brodholt, J.P., Piet, H., Siebert, J., Ryerson, F.J. (2015) Core formation and core composition from coupled geochemical and geophysical constraints. Proceedings of the National Academy of Sciences 112, 12310–12314.

Over time during core formation, fO2 is estimated to evolve from lower values of ΔIW-3.9 to ΔIW-1.9 to higher values of ΔIW-1.9 to ΔIW-1.0 (Rubie et al., 2011, Badro et al., 2015).
View in article
Bergin, E.A., Blake, G.A., Ciesla, F., Hirschmann, M.M., Li, J. (2015) Tracing the ingredients for a habitable earth from interstellar space through planet formation. Proceedings of the National Academy of Sciences 112, 8965–8970.

In Earth, the BSE C/N ratio is estimated to be higher than that of planetary building blocks including enstatite, carbonaceous chondrites, interstellar dust and gas (e.g., Marty, 2012; Bergin et al., 2015).
View in article
Recent studies estimate the BSE C/N ratio to be superchondritic based on measurements of gas bubbles trapped in mid-ocean ridge and ocean island basalts (Marty, 2012; Halliday, 2013; Bergin et al., 2015), and recent estimates of the BSE C/N ratio converge to 49 ± 9 (Bergin et al., 2015).
View in article
The key processes responsible for C and N redistribution among Earth reservoirs were equilibrium partitioning between the magma ocean and the growing metallic core and degassing of the magma ocean to the early atmosphere, but these processes have been insufficient to explain the estimated high BSE C/N ratio (Bergin et al., 2015; Hirschmann, 2016; Dalou et al., 2017).
View in article
In contrast, previous models assume all C and N are initially stored in silicate (Bergin et al., 2015; Hirschmann, 2016; Dalou et al., 2017).
View in article
Compared with previous models (Bergin et al., 2015), our model matches the estimated BSE C/N ratio for an extensive range of redox conditions, the degree of alloy-silicate re-equilibration and extent of loss of nascent atmosphere during accretion and core formation.
View in article
Considering the high C/N ratios (>10) of most planetary bodies (Bergin et al., 2015), the C/N ratios of the impactors’ cores would be even higher and therefore promote immiscible melting during impact.
View in article
Figure 3 [...] The horizontal yellow bar marks the range of the estimated present BSE C/N ratio (Bergin et al., 2015).
View in article
Brenker, F.E., Vollmer, C., Vincze, L., Vekemans, B., Szymanski, A., Janssens, K., Szaloki, I., Nasdala, L., Joswig, W., Kaminsky, F. (2007) Carbonates from the lower part of transition zone or even the lower mantle. Earth and Planetary Science Letters 260, 1–9.

In addition, the presence of deep mantle carbonates (e.g., Brenker et al., 2007) and high solubilities (10s to 1000s mg/g) of N in transition zone and lower mantle minerals (Yoshioka et al., 2018), both of which are sensitive to redox and pH conditions (e.g., Rohrbach and Schmidt, 2011; Mikhail et al., 2017) , would also influence the estimation of the BSE C/N ratio.
View in article
Dalou, C., Hirschmann, M.M., von der Handt, A., Mosenfelder, J., Armstrong, L.S. (2017) Nitrogen and carbon fractionation during core–mantle differentiation at shallow depth. Earth and Planetary Science Letters 458, 141–151.

The key processes responsible for C and N redistribution among Earth reservoirs were equilibrium partitioning between the magma ocean and the growing metallic core and degassing of the magma ocean to the early atmosphere, but these processes have been insufficient to explain the estimated high BSE C/N ratio (Bergin et al., 2015; Hirschmann, 2016; Dalou et al., 2017).
View in article
Core formation is expected to decrease the BSE C/N ratio because at relevant conditions C is a much stronger siderophile element than N (e.g., Dalou et al., 2017).
View in article
The amount of N stored in metallic core is controlled by oxygen fugacity (Dalou et al., 2017): 90.2 % N would stay in the core at oxidised bodies (ΔIW-0.5 to -1.0); this number decreases to 69.7 % and 12.1 % at modestly reduced (ΔIW-1.8 to -2.2) and more reduced bodies (ΔIW-3.4 to -3.6), respectively (Fig. S-9).
View in article
In contrast, previous models assume all C and N are initially stored in silicate (Bergin et al., 2015; Hirschmann, 2016; Dalou et al., 2017).
View in article
Fegley, B. (1983) Primordial retention of nitrogen by terrestrial planets and meteorites. Journal of Geophysical Research: Solid Earth 88, A853–A868.

Notably, both C and N form accessory minerals, such as diamonds, iron carbides and iron/titanium/chromium nitrides (e.g., Fegley, 1983; Javoy, 1997; Kaminsky and Wirth, 2017), which have not been considered in the estimated BSE C/N ratio yet.
View in article
Fei, Y., Brosh, E. (2014) Experimental study and thermodynamic calculations of phase relations in the Fe–C system at high pressure. Earth and Planetary Science Letters 408, 155–162.

While the Fe-C phase diagram has been well studied over a wide range of pressures (e.g., Fei and Brosh, 2014; Liu et al., 2016), very limited data are available on the phase relations of the Fe-N system (e.g., Guillermet and Du, 1994).
View in article
Grady, M.M., Wright, I.P. (2003) Elemental and Isotopic Abundances of Carbon and Nitrogen in Meteorites. Space Science Reviews 106, 231–248.

In contrast, C/N ratios measured in primitive CI chondrites (17 ± 3; Alexander et al., 2013) and enstatite chondrites (14 ± 12; Grady and Wright, 2003) are significantly lower.
View in article
Grumbach, M.P., Martin, R.M. (1996) Phase diagram of carbon at high pressures and temperatures. Physical Review B 54, 15730–15741.

In particular, melting behaviours are expected to be different in these two systems, as the C-rich end member, graphite/diamond, has a melting point of 4000-5000 K at relevant pressures (Grumbach and Martin, 1996), while the N-rich end member would be gas/supercritical fluid in the BSE.
View in article
Guillermet, A.F., Du, H. (1994) Thermodynamic Analysis of the Fe-N System Using the Compound-Energy Model with Predictions of the Vibrational Entropy. Zeitschrift Fur Metallkunde 85, 154–163.

While the Fe-C phase diagram has been well studied over a wide range of pressures (e.g., Fei and Brosh, 2014; Liu et al., 2016), very limited data are available on the phase relations of the Fe-N system (e.g., Guillermet and Du, 1994).
View in article
Halliday, A.N. (2013) The origins of volatiles in the terrestrial planets. Geochimica et Cosmochimica Acta 105, 146–171.

Recent studies estimate the BSE C/N ratio to be superchondritic based on measurements of gas bubbles trapped in mid-ocean ridge and ocean island basalts (Marty, 2012; Halliday, 2013; Bergin et al., 2015), and recent estimates of the BSE C/N ratio converge to 49 ± 9 (Bergin et al., 2015).
View in article
Hin, R.C., Coath, C.D., Carter, P.J., Nimmo, F., Lai, Y.-J., Pogge von Strandmann, P.A.E., Willbold, M., Leinhardt, Z.M., Walter, M.J., Elliott, T. (2017) Magnesium isotope evidence that accretional vapour loss shapes planetary compositions. Nature 549, 511–515.

Vapourisation during accretion has recently been evoked to explain Mg, Si and Fe isotopic compositions in the BSE (Hin et al., 2017) and the pattern of volatile element depletion (Norris and Wood, 2017), indicating its critical role in the early stage of Earth evolution.
View in article
Hirschmann, M.M. (2016) Constraints on the early delivery and fractionation of Earth’s major volatiles from C/H, C/N, and C/S ratios. American Mineralogist 101, 540–553.

The key processes responsible for C and N redistribution among Earth reservoirs were equilibrium partitioning between the magma ocean and the growing metallic core and degassing of the magma ocean to the early atmosphere, but these processes have been insufficient to explain the estimated high BSE C/N ratio (Bergin et al., 2015; Hirschmann, 2016; Dalou et al., 2017).
View in article
Degassing has not been thought to significantly affect the C/N ratio, as chemical analysis of volatiles in silicate glasses quenched from high pressure-temperature (P-T) experiments indicates that the solubilities of C and N in the magma ocean are comparable (Hirschmann, 2016).
View in article
In contrast, previous models assume all C and N are initially stored in silicate (Bergin et al., 2015; Hirschmann, 2016; Dalou et al., 2017).
View in article
Javoy, M. (1997) The major volatile elements of the Earth: Their origin, behavior, and fate. Geophysical Research Letters 24, 177–180.

Notably, both C and N form accessory minerals, such as diamonds, iron carbides and iron/titanium/chromium nitrides (e.g., Fegley, 1983; Javoy, 1997; Kaminsky and Wirth, 2017), which have not been considered in the estimated BSE C/N ratio yet.
View in article
Kaminsky, F., Wirth, R. (2017) Nitrides and carbonitrides from the lowermost mantle and their importance in the search for Earth’s “lost” nitrogen. American Mineralogist 102, 1667–1676.

Notably, both C and N form accessory minerals, such as diamonds, iron carbides and iron/titanium/chromium nitrides (e.g., Fegley, 1983; Javoy, 1997; Kaminsky and Wirth, 2017), which have not been considered in the estimated BSE C/N ratio yet.
View in article
Kono, Y., Park, C., Kenney-Benson, C., Shen, G., Wang, Y. (2014) Toward comprehensive studies of liquids at high pressures and high temperatures: Combined structure, elastic wave velocity, and viscosity measurements in the Paris–Edinburgh cell. Physics of the Earth and Planetary Interiors 228, 269–280.

A standard PE sample assembly configuration was employed (Kono et al., 2014), which used X-ray transparent MgO as the sample capsule and a cylindrical graphite tube outside sample capsule as the heater.
View in article
Kono, Y., Kenney-Benson, C., Shibazaki, Y., Park, C., Wang, Y., Shen, G. (2015) X-ray imaging for studying behavior of liquids at high pressures and high temperatures using Paris-Edinburgh press. Review of Scientific Instruments 86, 072207.

The immiscible droplets with lighter colours in X-ray radiographic images are enriched in N because the contrast in X-ray radiographic images reflects density differences (Kono et al., 2015).
View in article
Kowalski, M., Spencer, P.J. (1995) Thermodynamic reevaluation of the C-O, Fe-O and Ni-O systems: Remodelling of the liquid, BCC and FCC phases. Calphad 19, 229–243.

A similar phenomenon has been observed in the Fe-O system (Kowalski and Spencer, 1995; Tsuno et al., 2007): at 1 bar, O solubility increases from ~0 to ~5 mol. % when temperature increases from ~1811 K to ~2340 K (Kowalski and Spencer, 1995).
View in article
Liu, J., Li, J., Hrubiak, R., Smith, J.S. (2016) Origins of ultralow velocity zones through slab-derived metallic melt. Proceedings of the National Academy of Sciences 113, 5547–5551.

While the Fe-C phase diagram has been well studied over a wide range of pressures (e.g., Fei and Brosh, 2014; Liu et al., 2016), very limited data are available on the phase relations of the Fe-N system (e.g., Guillermet and Du, 1994).
View in article
Lock, S.J., Stewart, S.T. (2017) The structure of terrestrial bodies: Impact heating, corotation limits, and synestias. Journal of Geophysical Research: Planets 122, 2016JE005239.

In addition, for Earth-like planets at the proposed synestia stage (Lock and Stewart, 2017), the magma ocean is surrounded by massive vapourised materials, which are at pressures between 0.1 bar and 1.0 GPa.
View in article
Marty, B. (2012) The origins and concentrations of water, carbon, nitrogen and noble gases on Earth. Earth and Planetary Science Letters 313–314, 56–66.

In Earth, the BSE C/N ratio is estimated to be higher than that of planetary building blocks including enstatite, carbonaceous chondrites, interstellar dust and gas (e.g., Marty, 2012; Bergin et al., 2015).
View in article
Recent studies estimate the BSE C/N ratio to be superchondritic based on measurements of gas bubbles trapped in mid-ocean ridge and ocean island basalts (Marty, 2012; Halliday, 2013; Bergin et al., 2015), and recent estimates of the BSE C/N ratio converge to 49 ± 9 (Bergin et al., 2015).
View in article
Mikhail, S., Barry, P.H., Sverjensky, D.A. (2017) The relationship between mantle pH and the deep nitrogen cycle. Geochimica et Cosmochimica Acta 209, 149–160.

In addition, the presence of deep mantle carbonates (e.g., Brenker et al., 2007) and high solubilities (10s to 1000s mg/g) of N in transition zone and lower mantle minerals (Yoshioka et al., 2018), both of which are sensitive to redox and pH conditions (e.g., Rohrbach and Schmidt, 2011; Mikhail et al., 2017) , would also influence the estimation of the BSE C/N ratio.
View in article
Norris, C.A., Wood, B.J. (2017) Earth’s volatile contents established by melting and vaporization. Nature 549, 507–510.

Vapourisation during accretion has recently been evoked to explain Mg, Si and Fe isotopic compositions in the BSE (Hin et al., 2017) and the pattern of volatile element depletion (Norris and Wood, 2017), indicating its critical role in the early stage of Earth evolution.
View in article
Rohrbach, A., Schmidt, M.W. (2011) Redox freezing and melting in the Earth’s deep mantle resulting from carbon-iron redox coupling. Nature 472, 209–212.

In addition, the presence of deep mantle carbonates (e.g., Brenker et al., 2007) and high solubilities (10s to 1000s mg/g) of N in transition zone and lower mantle minerals (Yoshioka et al., 2018), both of which are sensitive to redox and pH conditions (e.g., Rohrbach and Schmidt, 2011; Mikhail et al., 2017) , would also influence the estimation of the BSE C/N ratio.
View in article
Roskosz, M., Bouhifd, M.A., Jephcoat, A.P., Marty, B., Mysen, B.O. (2013) Nitrogen solubility in molten metal and silicate at high pressure and temperature. Geochimica et Cosmochimica Acta 121, 15–28.

The presence of immiscible N-rich fluid in silicate-alloy systems has been observed in a previous partitioning study on metallic-silicate melts up to 10 GPa (Roskosz et al., 2013), supporting that this immiscible phenomenon could occur in a realistic magma ocean phase assemblage.
View in article
Rubie, D.C., Frost, D.J., Mann, U., Asahara, Y., Nimmo, F., Tsuno, K., Kegler, P., Holzheid, A., Palme, H. (2011) Heterogeneous accretion, composition and core–mantle differentiation of the Earth. Earth and Planetary Science Letters 301, 31–42.

Over time during core formation, fO2 is estimated to evolve from lower values of ΔIW-3.9 to ΔIW-1.9 to higher values of ΔIW-1.9 to ΔIW-1.0 (Rubie et al., 2011, Badro et al., 2015).
View in article
Speelmanns, I.M., Schmidt, M.W., Liebske, C. (2018) Nitrogen Solubility in Core Materials. Geophysical Research Letters 45, 7434–7443.

Figure 2 [...] The blue and red curves are modelled N solubilities in Fe-N-C melt at 2000 K and 2300 K, respectively, from Speelmanns et al. (2018).
View in article
A recent study also infers the presence of N-rich fluid from Fe-N-C melt based on the ex situ analysis of quenched samples (Speelmanns et al., 2018).
View in article
However, the C and Pt contaminations up to 12.5 wt. % from sample capsules are not incorporated into their N solubility models (Speelmanns et al., 2018), which may explain the offsets between these two studies (Fig. 2).
View in article
Tsuno, K., Ohtani, E., Terasaki, H. (2007) Immiscible two-liquid regions in the Fe–O–S system at high pressure: Implications for planetary cores. Physics of the Earth and Planetary Interiors 160, 75–85.

A similar phenomenon has been observed in the Fe-O system (Kowalski and Spencer, 1995; Tsuno et al., 2007): at 1 bar, O solubility increases from ~0 to ~5 mol. % when temperature increases from ~1811 K to ~2340 K (Kowalski and Spencer, 1995).
View in article
Such a miscibility gap persists to at least 21 GPa in the Fe-O system and O solubility in metallic melt increases with a rate of 0.01-0.03 mol./K between 15-21 GPa and 2050-2350 K (Tsuno et al., 2007).
View in article
Yoshioka, T., Wiedenbeck, M., Shcheka, S., Keppler, H. (2018) Nitrogen solubility in the deep mantle and the origin of Earth’s primordial nitrogen budget. Earth and Planetary Science Letters 488, 134–143.

In addition, the presence of deep mantle carbonates (e.g., Brenker et al., 2007) and high solubilities (10s to 1000s mg/g) of N in transition zone and lower mantle minerals (Yoshioka et al., 2018), both of which are sensitive to redox and pH conditions (e.g., Rohrbach and Schmidt, 2011; Mikhail et al., 2017) , would also influence the estimation of the BSE C/N ratio.
View in article
top
Supplementary Information
The Supplementary Information includes:
- Synchrotron X-ray Radiography Experiments
- Chemical Analysis of Recovered Samples
- The Evolution of the BSE C/N Ratio during Core Formation
- Table S-1
- Figures S-1 to S-10
- Video S-1
- Supplementary Information References
Download Video S-1 (AVI).
Download the Supplementary Information (PDF).
Figures and Tables
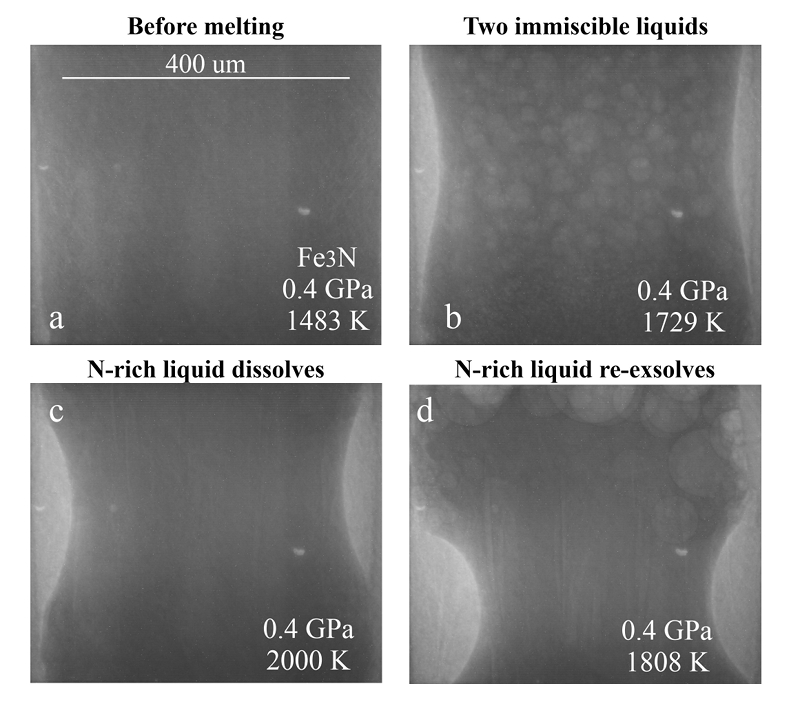
Figure 1 Representative X-ray radiographic images showing the evolution of the miscibility gap in Fe-N-C system under high pressure and high temperature conditions (run 4-17). This in situ X-ray radiography experiment starts at 0.4 GPa with Fe3N as the starting material. (a) The sample is below solidus at 0.4 GPa and 1483 K. (b) Around 1729 K, immiscible N-rich supercritical fluid coexists with metallic liquid. (c) At higher temperatures, N-rich supercritical fluid partially dissolves into metallic liquid. (d) Lowering temperature re-exsolves N-rich supercritical fluid from metallic liquid. The corresponding movie is shown in Video S-1.
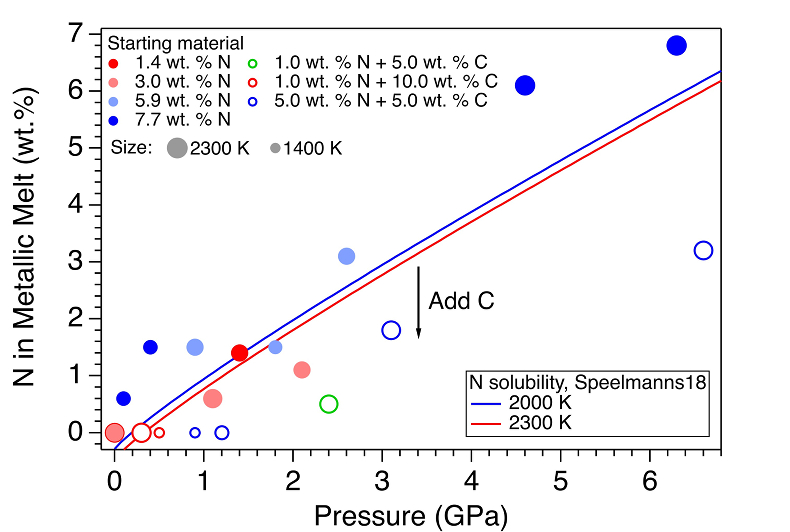
Figure 2 The pressure dependence of N solubility in Fe-N-C melt. The solid circles are for Fe-N starting materials; the open circles are for Fe-N-C starting materials. Symbol sizes are proportional to the temperatures (1400-2300 K) at which the experiments are quenched and colours indicate initial compositions (see legend). The blue and red curves are modelled N solubilities in Fe-N-C melt at 2000 K and 2300 K, respectively, from Speelmanns et al. (2018)
Speelmanns, I.M., Schmidt, M.W., Liebske, C. (2018) Nitrogen Solubility in Core Materials. Geophysical Research Letters 45, 7434–7443.
.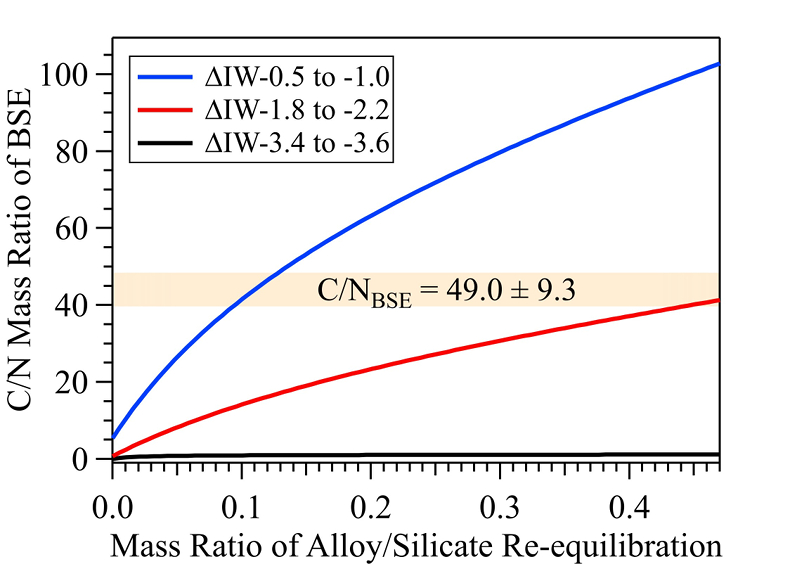
Figure 3 The evolution of the BSE C/N ratio with the degree of re-equilibration between alloy and silicate melts during core formation. The blue curve is calculated at oxidised conditions (ΔIW-0.5 to ΔIW-1.0); the red curve is for reduced conditions (ΔIW-1.8 to ΔIW-2.2) and the black curve is for very reduced conditions (ΔIW-3.4 to ΔIW-3.6). The horizontal yellow bar marks the range of the estimated present BSE C/N ratio (Bergin et al., 2015
Bergin, E.A., Blake, G.A., Ciesla, F., Hirschmann, M.M., Li, J. (2015) Tracing the ingredients for a habitable earth from interstellar space through planet formation. Proceedings of the National Academy of Sciences 112, 8965–8970.
).