Abiogenesis not required to explain the origin of volcanic-hydrothermal hydrocarbons
Affiliations | Corresponding Author | Cite as | Funding information- Share this article
Article views:4,524Cumulative count of HTML views and PDF downloads.
- Download Citation
- Rights & Permissions
top
Abstract
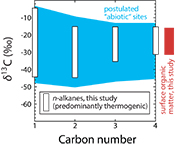
Figures and Tables
![]() Figure 1 Plot of δ13C of individual n-alkanes against carbon number. δ13C ranges of modern marine organic matter (Druffel et al., 1992; Sara et al., 2007), terrestrial C3 vegetation (Kohn, 2010) and Archean organic matter (Hayes and Waldbauer, 2006) are shown for comparison. (a) Emissions that are characterised by invariant δ13C-C2+. (b) Compilation of all n-alkane data analysed in this study. (c) Comparison between n-alkane data from this study (area in grey) and data available from abiotic sites (Supplementary Information): hydrothermal sites (blue); ophiolite gases (black); old craton gases (red) and inclusions in igneous rocks (green). | ![]() Figure 2 Plot of δ2H-CH4 vs. δ13C-CH4. Samples with an obvious microbial origin (δ13C-CH4 < –60 ‰, Fig. 1b) are not considered. (a) Data classified after the origin of external water feeding the hydrothermal system (Table S-1). Blue and green squares are representative of the carbon and hydrogen isotopic compositions of marine organic matter and C3 plants, respectively (Schoell, 1984). The carbon and hydrogen isotopic compositions of instantaneously generated fractions of methane deriving from open system cracking of marine and terrestrial (C3 plants) organic matter were modelled as a function of the fraction of precursor sites remaining inside the cracked organic matter (Supplementary Information). The cracking trend for methane deriving from marine organic matter (blue line) matches the variation of δ13C-CH4 and δ2H-CH4 observed for seawater-fed hydrothermal systems (blue data points). The cracking trend for methane from terrestrial organic matter (green line) corresponds to the slope described by most low δ13C-CH4 samples from meteoric water-fed hydrothermal systems (green data points), but – on average – occurs shifted relative to the latter to higher δ13C and δ2H. This implies that methane precursor sites in decomposing terrestrial organic matter either occur depleted in 13C and 2H with respect to the average C3 plant isotopic composition or that the corresponding carbon and hydrogen isotope fractionations (αC, αH) are larger than those obtained from xylite (Berner et al., 1995), with αH /αC remaining unchanged. Both possibilities are consistent with carbon isotope constraints on pyrolysis of coal (Cramer et al., 1998). (b) Data classified after the style of degassing (wells vs. fumaroles). (c) Comparison between methane data from this study and data available from other abiotic sites (Supplementary Information): labelling as in Figure 1c, extended by (hyper)alkaline spring data (open symbols). Field characteristic for methane from microbial (c) and confined sedimentary systems (b, c) redrawn after Schoell (1988). |
Figure 1 | Figure 2 |
top
Introduction
The ability to distinguish between biotic and abiotic hydrocarbon sources on Earth is essential to understand fully the formation of crustal hydrocarbon reservoirs, identify the origin of hydrocarbons such as methane on other planets and resolve the potential role of abiotic methane in the emergence of life. It is well known that crustal hydrocarbons largely derive from biotic sources, i.e. from microbial production and thermal decomposition of organic matter (e.g., Etiope and Sherwood Lollar, 2013
Etiope, G., Sherwood Lollar, B. (2013) Abiotic methane on Earth. Reviews of Geophysics 51, 276–299
; Etiope and Schoell, 2014Etiope, G., Schoell, M. (2014) Abiotic gas: atypical but not rare. Elements 10, 291–296.
). Abiotic hydrocarbon formation (i.e. generation from pure inorganic substances, without any involvement of organic carbon) has been postulated to take place in a variety of natural systems where inorganically derived CO or CO2, water, reducing reagents and catalysts and/or heat are available. These include hydrothermal and low temperature (T < 100 °C) mafic and ultramafic systems, subduction-related, volcanic-hydrothermal systems and igneous intrusions (e.g., Etiope and Sherwood Lollar, 2013Etiope, G., Sherwood Lollar, B. (2013) Abiotic methane on Earth. Reviews of Geophysics 51, 276–299
; Etiope and Schoell, 2014Etiope, G., Schoell, M. (2014) Abiotic gas: atypical but not rare. Elements 10, 291–296.
).Most prominently, the following criteria have been used to identify abiotic hydrocarbon occurrences: i) methane with δ13C ≥ –20 ‰ (e.g., Welhan and Craig, 1979
Welhan, J.A., Craig, H. (1979) Methane and hydrogen in East Pacific Rise hydrothermal fluids. Geophysical Research Letters 6, 829–831.
), ii) the occurrence of a carbon isotope reversal between ethane and methane (i.e. methane more enriched in 13C than ethane, contrary to what is observed for n-alkanes from confined sedimentary hydrocarbon reservoirs) (e.g., Des Marais et al., 1981Des Marais, D.J., Donchin, J.H., Nehring, N.L., Truesdell, A.H. (1981) Molecular carbon isotopic evidence for the origin of geothermal hydrocarbons. Nature 292, 826–828.
; Sherwood Lollar et al., 2002Sherwood Lollar, B., Westgate, T.D., Ward, J.A., Slater, G.F., Lacrampe-Couloume, G. (2002) Abiotic formation of gaseous alkanes in the Earth’s crust as a minor source of global hydrocarbon reservoirs. Nature 416, 522–524.
; Proskurowski et al., 2008Proskurowski, G., Lilley, M.D., Seewald, J.S., Früh-Green, G.L., Olson, E.J., Lupton, J.E., Sylva, S.P., Kelley, D.S. (2008) Abiotic hydrocarbon production at Lost City hydrothermal field. Science 319, 604–607.
) and iii) methane in apparent chemical and isotopic equilibrium with inorganically derived CO2 (Fiebig et al., 2007Fiebig, J., Woodland, A.B., Spangenberg, J., Oschmann, W. (2007) Natural evidence for rapid abiotic hydrothermal generation of CH4. Geochimica et Cosmochimica Acta 71, 3028–3039.
). Using these criteria, contrary views have been presented on the origin of volcanic-hydrothermal n-alkanes. Whereas Des Marais et al. (1981)Des Marais, D.J., Donchin, J.H., Nehring, N.L., Truesdell, A.H. (1981) Molecular carbon isotopic evidence for the origin of geothermal hydrocarbons. Nature 292, 826–828.
identified Yellowstone hydrocarbons to derive from a thermogenic source, Fiebig et al. (2007)Fiebig, J., Woodland, A.B., Spangenberg, J., Oschmann, W. (2007) Natural evidence for rapid abiotic hydrothermal generation of CH4. Geochimica et Cosmochimica Acta 71, 3028–3039.
ascribed n-alkanes from Nisyros, Vesuvio and Ischia to an abiotic origin. Recently, 13C-labelled experiments have called the relevance of abiotic hydrocarbon production under hydrothermal conditions into question, due to sluggish reaction rates (McCollom, 2016McCollom, T.M. (2016) Abiotic methane formation during experimental serpentinization of olivine. Proceedings of the National Academy of Sciences of the USA 113, 13965–13970.
). Here, we present and discuss the first global data set of the carbon and hydrogen isotope compositions of n-alkanes in hydrothermal fluids to gain more detailed insights into hydrocarbon formation under natural hydrothermal conditions.top
Methods
We sampled two phase well fluids (n = 29) and steam vent fumaroles (n = 61) from 28 volcanic-hydrothermal fields in New Zealand, Iceland, Argentina, USA (Alaska), Italy, Greece, Portugal (Azores) and Spain (Canary Islands) (Table S-1). Sampled locations represent all types of volcanism and a wide range of reservoir temperatures (200 - 450 °C), with the origin of external water being dominantly meteoric and/or seawater (Table S-1 and references therein). Terrestrial vegetation at the sampled sites is largely dominated by C3 plants (Still et al., 2003
Still, C.J., Berry, J.A., Collatz, G.J., DeFries, R.S. (2003) Global distribution of C3 and C4 vegetation: Carbon cycle implications. Global Biogeochemical Cycles 17, doi: 10.1029/2001GB001807.
). We analysed the carbon isotopic compositions of methane, ethane, propane and n-butane as well as the hydrogen isotopic compositions of methane and water in the discharged fluids (Supplementary Information; Tables S-2, S-3).top
Results and Discussion
For several locations, carbon isotopes are homogeneously distributed among ethane, propane and n-butane (C2+ hydrocarbons), with variations in δ13C2+ ≤ 1.0 ‰ (Fig. 1a). At Reykjanes, δ13C-C2+ of –16 ‰ (well 11) and –17 to –18 ‰ (well 12) overlap with the carbon isotopic composition of particulate organic carbon (–17.5 ‰ ≥ δ13C-POC ≥ –22.2 ‰) and modern sedimentary organic matter (–16.5 ‰ ≥ δ13C-SOM ≥ –19.4 ‰) that are characteristic of the water masses surrounding the Reykjanes peninsula (Sara et al., 2007
Sara, G., De Pirro, M., Romano, C., Rumolo, P., Sprovieri, M., Mazzola, A. (2007) Sources of organic matter for intertidal consumers on Ascophyllum-shores (SW Iceland): a multi-stable isotope approach. Helgoland Marine Research 61, 297–302.
). For Esguicho (Furnas village) δ13C-C2+ of –28 to –29 ‰ perfectly agree with the average carbon isotopic composition of terrestrial plants growing in the Furnas caldera (–28.4 ‰; Pasquier-Cardin et al., 1999Pasquier-Cardin, A., Allard, P., Ferreira, T., Hatte, C., Coutinho, R., Fontugne, M., Jaudon, M. (1999) Magma-derived CO2 emissions recorded in 14C and 13C content of plants growing in Furnas caldera, Azores. Journal of Volcanology and Geothermal Research 92, 195–207.
). The hydrothermal reservoir at Reykjanes is predominantly fed by seawater, whereas the hydrothermal system beneath Furnas village is exclusively sourced by meteoric water (Table S-1). The same patterns - invariant δ13C-C2+, but absolute values changing with the source of water - are observed at Nisyros (seawater-fed hydrothermal system, δ13C-C2+ around –18 ‰), Ischia and Rotokawa well 14 (both meteoric water-fed systems, δ13C-C2+ around –27 ‰) (Fig. 1a; Table S-1). These observations strongly imply that local organic matter is transported by external waters into the corresponding hydrothermal reservoirs, where it is finally subjected to high temperature pyrolysis. At these temperatures, the carbon isotope fractionation between the C2+ hydrocarbons and the source organic matter becomes insignificantly small and, hence, the δ13C-C2+ becomes indicative of the bulk organic matter decomposing at depth.
Figure 1 Plot of δ13C of individual n-alkanes against carbon number. δ13C ranges of modern marine organic matter (Druffel et al., 1992
Druffel, E.R.M., Williams, P.M., Bauer, J.E., Ertel, J.R. (1992) Cycling of dissolved and particulate organic matter in the open ocean. Journal of Geophysical Research 97, 15639–15659.
; Sara et al., 2007Sara, G., De Pirro, M., Romano, C., Rumolo, P., Sprovieri, M., Mazzola, A. (2007) Sources of organic matter for intertidal consumers on Ascophyllum-shores (SW Iceland): a multi-stable isotope approach. Helgoland Marine Research 61, 297–302.
), terrestrial C3 vegetation (Kohn, 2010Kohn, M.J. (2010) Carbon isotope compositions of terrestrial C3 plants as indicators of (paleo)ecology and (paleo)climate. Proceedings of the National Academy of Sciences of the USA 107, 19691–19695.
) and Archean organic matter (Hayes and Waldbauer, 2006Hayes, J.M., Waldbauer, J.R. (2006) The carbon cycle and associated redox processes through time. Philosophical Transactions of the Royal Society B 361, 931–950.
) are shown for comparison. (a) Emissions that are characterised by invariant δ13C-C2+. (b) Compilation of all n-alkane data analysed in this study. (c) Comparison between n-alkane data from this study (area in grey) and data available from abiotic sites (Supplementary Information): hydrothermal sites (blue); ophiolite gases (black); old craton gases (red) and inclusions in igneous rocks (green).Thermogenic C2+ hydrocarbon production is not restricted to systems with invariant δ13C-C2+, but is most likely important in all systems. Although the majority of sampled discharges exhibit significant differences among δ13C-C2+ values (Tables S-2, S-3), the carbon isotopic composition of n-butane, the longest n-alkane analysed in this study, consistently occurs within the range reported for modern marine dissolved organic carbon (–18 ‰ ≥ δ13C-DOC ≥ –23 ‰; Druffel et al., 1992
Druffel, E.R.M., Williams, P.M., Bauer, J.E., Ertel, J.R. (1992) Cycling of dissolved and particulate organic matter in the open ocean. Journal of Geophysical Research 97, 15639–15659.
), modern marine particulate organic carbon (–17 ‰ ≥ δ13C-POC ≥ –25 ‰; Druffel et al., 1992Druffel, E.R.M., Williams, P.M., Bauer, J.E., Ertel, J.R. (1992) Cycling of dissolved and particulate organic matter in the open ocean. Journal of Geophysical Research 97, 15639–15659.
; Sara et al., 2007Sara, G., De Pirro, M., Romano, C., Rumolo, P., Sprovieri, M., Mazzola, A. (2007) Sources of organic matter for intertidal consumers on Ascophyllum-shores (SW Iceland): a multi-stable isotope approach. Helgoland Marine Research 61, 297–302.
) and modern terrestrial C3 plants (–20 ‰ ≥ δ13C ≥ –37 ‰, with most data clustering between –23 ‰ ≥ δ13C ≥ –31.5 ‰ and averaging at δ13C = –28.5 ‰; Kohn, 2010Kohn, M.J. (2010) Carbon isotope compositions of terrestrial C3 plants as indicators of (paleo)ecology and (paleo)climate. Proceedings of the National Academy of Sciences of the USA 107, 19691–19695.
) (Fig. 1b). Moreover, relative variations in δ13C decrease in the order ethane – propane – n-butane (Fig. 1b). Both patterns are consistent with isotope fractionation principles of organic matter degradation according to which the carbon isotope fractionation between source organic matter and the evolving gaseous n-alkane decreases with the number of carbon atoms constituting the n-alkane (Tang et al., 2000Tang, Y., Perry, J.K., Jenden, P.D., Schoell, M. (2000) Mathematical modeling of stable carbon isotope ratios in natural gases. Geochimica et Cosmochimica Acta 64, 2673–2687.
). In addition to the modern organic matter supplied by external waters, older organic matter contained in sediments can also contribute to overall hydrocarbon production, as becomes evident from Rotokawa δ13C-C2+ data (Table S-2). Although the Rotokawa hydrothermal reservoir is exclusively sourced by meteoric waters (Table S-1), its δ13C-n-C4 values range from –27 to –17 ‰, pointing to the occurrence of a marine next to a terrestrial organic source. The marine organic end member is likely hosted in Mesozoic greywacke located at relatively shallow depths of 1-3 km underneath Rotokawa (Table S-1 and references therein).Several additional observations imply that methane in the sampled discharges is also predominantly derived from the thermal decomposition of organic matter, and that the source organics are transported by external waters from the surface to reservoir depth. First, even if methane samples with an obvious microbial origin (Furnas B and Furnas Lake 2, Azores; Table S-3), as indicated by relatively strong depletions in 13C and 2H; Whiticar et al., 1999
Whiticar, M.J. (1999) Carbon and hydrogen isotope systematics of bacterial formation and oxidation of methane. Chemical Geology 161, 291–314.
) are excluded, methane still exhibits the largest variations in δ13C of all analysed n-alkanes (Fig. 1b). Second, in δ13C vs. δ2H space (Fig. 2a), methane from meteoric water- and seawater-fed hydrothermal systems plots along trends that are characteristic of open system, high temperature cracking of terrestrial and marine organic matter, respectively (Berner et al., 1995Berner, U., Faber, E., Scheeder, G., Panten, D. (1995) Primary cracking of algal and landplant kerogens: kinetic models of isotope variations in methane, ethane and propane. Chemical Geology 126, 233–245.
; Supplementary Information). Third, in the same space, methane from volcanic-hydrothermal systems plots into a field that has a shape similar to that characteristic of thermogenic methane from confined sedimentary systems but is, relative to the latter, shifted to higher δ13C values (Fig. 2b). Generally, the magnitude of carbon isotope fractionation between precursor organic matter and evolving n-alkane decreases with increasing temperature (e.g., Tang et al., 2000Tang, Y., Perry, J.K., Jenden, P.D., Schoell, M. (2000) Mathematical modeling of stable carbon isotope ratios in natural gases. Geochimica et Cosmochimica Acta 64, 2673–2687.
). The observed 13C-enrichment of thermogenic methane in volcanic-hydrothermal fluids may, therefore, result from relatively high reservoir temperatures of 200 - 450 °C (Table S-1), well-exceeding those of confined sedimentary reservoirs where methane generation takes place primarily between ~150 - 220 °C (Quigley and MacKenzie, 1988Quigley, T.M., MacKenzie, A.S. (1988) The temperatures of oil and gas formation in the sub-surface. Nature 333, 549–552.
). In addition, modern marine organic matter is enriched in 13C by 5 to 10 ‰ relative to the marine organic matter of pre-Cenozoic age (Hayes et al., 1999Hayes, J.M., Strauss, H., Kaufmann, A.J. (1999) The abundance of 13C in marine organic matter and isotopic fractionation in the global biogeochemical cycle of carbon during the past 800 Ma. Chemical Geology 161, 103–125.
) that provides the source of kerogen in confined sedimentary reservoirs. Fourth, in Icelandic systems, which are characterised by the absence of organic sediments, DOC and POC concentrations of meteoric water and/or seawater alone are sufficiently high to balance n-alkane concentrations in the discharged fluids (Fig. S-1). Fifth, under steady state conditions, water recharge rates at depth should be higher in well reservoirs than in naturally degassing systems, as in well systems the reservoir fluid is continuously exploited at the surface in addition to the steam. The flux of fresh, immature organics (depleted in 13C) through the reservoir should, therefore, be higher in well systems. This is consistent with the observation that well discharges display on average lower δ13C-CH4 values than fumaroles (Fig. 2b).
Figure 2 Plot of δ2H-CH4 vs. δ13C-CH4. Samples with an obvious microbial origin (δ13C-CH4 < –60 ‰, Fig. 1b) are not considered. (a) Data classified after the origin of external water feeding the hydrothermal system (Table S-1). Blue and green squares are representative of the carbon and hydrogen isotopic compositions of marine organic matter and C3 plants, respectively (Schoell, 1984
Schoell, M. (1984) Wasserstoff- und Kohlenstoffisotope in organischen Substanzen, Erdölen und Erdgasen. Geologisches Jahrbuch D67, 1–161.
). The carbon and hydrogen isotopic compositions of instantaneously generated fractions of methane deriving from open system cracking of marine and terrestrial (C3 plants) organic matter were modelled as a function of the fraction of precursor sites remaining inside the cracked organic matter (Supplementary Information). The cracking trend for methane deriving from marine organic matter (blue line) matches the variation of δ13C-CH4 and δ2H-CH4 observed for seawater-fed hydrothermal systems (blue data points). The cracking trend for methane from terrestrial organic matter (green line) corresponds to the slope described by most low δ13C-CH4 samples from meteoric water-fed hydrothermal systems (green data points), but – on average – occurs shifted relative to the latter to higher δ13C and δ2H. This implies that methane precursor sites in decomposing terrestrial organic matter either occur depleted in 13C and 2H with respect to the average C3 plant isotopic composition or that the corresponding carbon and hydrogen isotope fractionations (αC, αH) are larger than those obtained from xylite (Berner et al., 1995Berner, U., Faber, E., Scheeder, G., Panten, D. (1995) Primary cracking of algal and landplant kerogens: kinetic models of isotope variations in methane, ethane and propane. Chemical Geology 126, 233–245.
), with αH /αC remaining unchanged. Both possibilities are consistent with carbon isotope constraints on pyrolysis of coal (Cramer et al., 1998Cramer, B., Krooss, B.M., Littke, R. (1998) Modelling isotope fractionation during primary cracking of natural gas: a reaction kinetic approach. Chemical Geology 149, 235–250.
). (b) Data classified after the style of degassing (wells vs. fumaroles). (c) Comparison between methane data from this study and data available from other abiotic sites (Supplementary Information): labelling as in Figure 1c, extended by (hyper)alkaline spring data (open symbols). Field characteristic for methane from microbial (c) and confined sedimentary systems (b, c) redrawn after Schoell (1988)Schoell, M. (1988) Multiple origins of methane in the Earth. Chemical Geology 71, 1–10.
.Two reasons may account for 2H departing from the predicted cracking and degassing trends at high organic matter maturities characterised by δ13C-CH4 ≥ –20 ‰ (Fig. 2a). First, hydrogen isotope exchange between CH4 and water may occur at the elevated temperatures characteristic of hydrothermal systems, driving CH4 towards isotopic equilibrium with water at T ≥ 300 °C (Fig. S-2a). Alternatively, the decomposing organic matter at depth may be able to exchange hydrogen with the reservoir water such that the hydrogen isotopic composition of the organic matter and that of the methane becomes progressively buffered by water with increasing temperature and/or increasing organic matter maturity.
The isotopic signature of n-alkanes from potential abiotic natural sites and our samples are shown for comparison in Figs. 1c and 2c. There is significant overlap between our data and the inferred abiotic data set. However, based on our observations we consider a predominantly abiotic origin for the volcanic-hydrothermal hydrocarbons to be highly unlikely. In particular, a predominantly abiotic origin of the n-alkanes analysed in this study would require that our overall data set fortuitously follows isotopic fractionation principles that are characteristic of thermogenesis as detailed above.
Our observation that the overall isotopic trends displayed by volcanic-hydrothermal hydrocarbons are consistent with a predominant thermogenic origin for these gases has important implications for the reliability of criteria previously applied to identify abiotic hydrocarbon occurrences. First, δ13C-CH4 values exceeding those characteristic for methane from confined sedimentary systems are not indicative of abiogenesis (Fig. 2a). Second, the occurrence of a carbon isotope reversal (as is observed for several locations in this study; see Tables S-2, S-3) cannot be used as evidence for abiotic n-alkane generation either. Reversals can be obtained from thermogenic degradation and open system degassing alone (Fig. S-3), or from mixing of thermogenic n-alkanes from two or more sources of distinct organic maturity (Taran et al., 2007
Taran, Y.A., Kliger, G.A., Sevastyanov, V.S. (2007) Carbon isotope effects in the open-system Fischer–Tropsch synthesis. Geochimica et Cosmochimica Acta 71, 4474–4487.
). Third, because δ13C-CH4 is controlled by the relative fluxes of organic carbon into and methane carbon out of the system, carbon isotope equilibrium between CH4 and CO2 might not be attained, such that the apparent fractionation would only fortuitously correspond to equilibrium in some cases (Figs. S-2b, S-4).top
Conclusions
δ2H and δ13C of CH4 as well as δ13C of C2+ of n-alkanes from volcanic-hydrothermal emissions follow isotopic trends that are consistent with the principles of organic matter degradation under relatively high temperatures and open system conditions. No significant contribution from an abiotic source is required to explain the isotopic compositions and trends displayed by these n-alkanes. Source organic matter is supplied by external, surface-derived waters circulating through these systems and, if present, by sedimentary host rocks. Previously applied criteria characteristic for thermogenic hydrocarbon classification were developed for confined sedimentary thermogenic systems such as oil and gas fields that were not open to degassing during organic matter maturation. We argue that these criteria are not applicable to thermogenic systems open to degassing. Under such open system conditions, unusual enrichment in 13C and even carbon isotope reversals between methane and ethane can be obtained. As a consequence, reported abiotic hydrocarbon occurrences may be significantly overestimated, by mistakenly ascribing thermogenic hydrocarbons to an abiotic origin.
top
Acknowledgements
This work became possible through DFG grant FI 948/8-1.
Editor: Liane G. Benning
top
References
Berner, U., Faber, E., Scheeder, G., Panten, D. (1995) Primary cracking of algal and landplant kerogens: kinetic models of isotope variations in methane, ethane and propane. Chemical Geology 126, 233–245.

Second, in δ13C vs. δ2H space (Fig. 2a), methane from meteoric water- and seawater-fed hydrothermal systems plots along trends that are characteristic of open system, high temperature cracking of terrestrial and marine organic matter, respectively (Berner et al., 1995; Supplementary Information).
View in article
Figure 2 [...] This implies that methane precursor sites in decomposing terrestrial organic matter either occur depleted in 13C and 2H with respect to the average C3 plant isotopic composition or that the corresponding carbon and hydrogen isotope fractionations (αC, αH) are larger than those obtained from xylite (Berner et al., 1995), with αH /αC remaining unchanged.
View in article
Cramer, B., Krooss, B.M., Littke, R. (1998) Modelling isotope fractionation during primary cracking of natural gas: a reaction kinetic approach. Chemical Geology 149, 235–250.

Figure 2 [...] Both possibilities are consistent with carbon isotope constraints on pyrolysis of coal (Cramer et al., 1998).
View in article
Des Marais, D.J., Donchin, J.H., Nehring, N.L., Truesdell, A.H. (1981) Molecular carbon isotopic evidence for the origin of geothermal hydrocarbons. Nature 292, 826–828.

Most prominently, the following criteria have been used to identify abiotic hydrocarbon occurrences: i) methane with δ13C ≥ –20 ‰ (e.g., Welhan and Craig, 1979), ii) the occurrence of a carbon isotope reversal between ethane and methane (i.e. methane more enriched in 13C than ethane, contrary to what is observed for n-alkanes from confined sedimentary hydrocarbon reservoirs) (e.g., Des Marais et al., 1981; Sherwood Lollar et al., 2002; Proskurowski et al., 2008) and iii) methane in apparent chemical and isotopic equilibrium with inorganically derived CO2 (Fiebig et al., 2007).
View in article
Whereas Des Marais et al. (1981) identified Yellowstone hydrocarbons to derive from a thermogenic source, Fiebig et al. (2007) ascribed n-alkanes from Nisyros, Vesuvio and Ischia to an abiotic origin.
View in article
Druffel, E.R.M., Williams, P.M., Bauer, J.E., Ertel, J.R. (1992) Cycling of dissolved and particulate organic matter in the open ocean. Journal of Geophysical Research 97, 15639–15659.

Figure 1 [...] δ13C ranges of modern marine organic matter (Druffel et al., 1992; Sara et al., 2007), terrestrial C3 vegetation (Kohn, 2010) and Archean organic matter (Hayes and Waldbauer, 2006) are shown for comparison.
View in article
Although the majority of sampled discharges exhibit significant differences among δ13C-C2+ values (Tables S-2, S-3), the carbon isotopic composition of n-butane, the longest n-alkane analysed in this study, consistently occurs within the range reported for modern marine dissolved organic carbon (–18 ‰ ≥ δ13C-DOC ≥ –23 ‰; Druffel et al., 1992), modern marine particulate organic carbon (–17 ‰ ≥ δ13C-POC ≥ –25 ‰; Druffel et al., 1992; Sara et al., 2007) and modern terrestrial C3 plants (–20 ‰ ≥ δ13C ≥ –37 ‰, with most data clustering between –23 ‰ ≥ δ13C ≥ –31.5 ‰ and averaging at δ13C = –28.5 ‰; Kohn, 2010) (Fig. 1b).
View in article
Etiope, G., Sherwood Lollar, B. (2013) Abiotic methane on Earth. Reviews of Geophysics 51, 276–299

It is well known that crustal hydrocarbons largely derive from biotic sources, i.e. from microbial production and thermal decomposition of organic matter (e.g., Etiope and Sherwood Lollar, 2013; Etiope and Schoell, 2014).
View in article
These include hydrothermal and low temperature (T < 100 °C) mafic and ultramafic systems, subduction-related, volcanic-hydrothermal systems and igneous intrusions (e.g., Etiope and Sherwood Lollar, 2013; Etiope and Schoell, 2014).
View in article
Etiope, G., Schoell, M. (2014) Abiotic gas: atypical but not rare. Elements 10, 291–296.

It is well known that crustal hydrocarbons largely derive from biotic sources, i.e. from microbial production and thermal decomposition of organic matter (e.g., Etiope and Sherwood Lollar, 2013; Etiope and Schoell, 2014).
View in article
These include hydrothermal and low temperature (T < 100 °C) mafic and ultramafic systems, subduction-related, volcanic-hydrothermal systems and igneous intrusions (e.g., Etiope and Sherwood Lollar, 2013; Etiope and Schoell, 2014).
View in article
Fiebig, J., Woodland, A.B., Spangenberg, J., Oschmann, W. (2007) Natural evidence for rapid abiotic hydrothermal generation of CH4. Geochimica et Cosmochimica Acta 71, 3028–3039.

Most prominently, the following criteria have been used to identify abiotic hydrocarbon occurrences: i) methane with δ13C ≥ –20 ‰ (e.g., Welhan and Craig, 1979), ii) the occurrence of a carbon isotope reversal between ethane and methane (i.e. methane more enriched in 13C than ethane, contrary to what is observed for n-alkanes from confined sedimentary hydrocarbon reservoirs) (e.g., Des Marais et al., 1981; Sherwood Lollar et al., 2002; Proskurowski et al., 2008) and iii) methane in apparent chemical and isotopic equilibrium with inorganically derived CO2 (Fiebig et al., 2007).
View in article
Whereas Des Marais et al. (1981) identified Yellowstone hydrocarbons to derive from a thermogenic source, Fiebig et al. (2007) ascribed n-alkanes from Nisyros, Vesuvio and Ischia to an abiotic origin.
View in article
Hayes, J.M., Strauss, H., Kaufmann, A.J. (1999) The abundance of 13C in marine organic matter and isotopic fractionation in the global biogeochemical cycle of carbon during the past 800 Ma. Chemical Geology 161, 103–125.

In addition, modern marine organic matter is enriched in 13C by 5 to 10 ‰ relative to the marine organic matter of pre-Cenozoic age (Hayes et al., 1999) that provides the source of kerogen in confined sedimentary reservoirs.
View in article
Hayes, J.M., Waldbauer, J.R. (2006) The carbon cycle and associated redox processes through time. Philosophical Transactions of the Royal Society B 361, 931–950.

Figure 1 [...] δ13C ranges of modern marine organic matter (Druffel et al., 1992; Sara et al., 2007), terrestrial C3 vegetation (Kohn, 2010) and Archean organic matter (Hayes and Waldbauer, 2006) are shown for comparison.
View in article
Kohn, M.J. (2010) Carbon isotope compositions of terrestrial C3 plants as indicators of (paleo)ecology and (paleo)climate. Proceedings of the National Academy of Sciences of the USA 107, 19691–19695.

Figure 1 [...] δ13C ranges of modern marine organic matter (Druffel et al., 1992; Sara et al., 2007), terrestrial C3 vegetation (Kohn, 2010) and Archean organic matter (Hayes and Waldbauer, 2006) are shown for comparison.
View in article
Although the majority of sampled discharges exhibit significant differences among δ13C-C2+ values (Tables S-2, S-3), the carbon isotopic composition of n-butane, the longest n-alkane analysed in this study, consistently occurs within the range reported for modern marine dissolved organic carbon (–18 ‰ ≥ δ13C-DOC ≥ –23 ‰; Druffel et al., 1992), modern marine particulate organic carbon (–17 ‰ ≥ δ13C-POC ≥ –25 ‰; Druffel et al., 1992; Sara et al., 2007) and modern terrestrial C3 plants (–20 ‰ ≥ δ13C ≥ –37 ‰, with most data clustering between –23 ‰ ≥ δ13C ≥ –31.5 ‰ and averaging at δ13C = –28.5 ‰; Kohn, 2010) (Fig. 1b).
View in article
McCollom, T.M. (2016) Abiotic methane formation during experimental serpentinization of olivine. Proceedings of the National Academy of Sciences of the USA 113, 13965–13970.

Recently, 13C-labelled experiments have called the relevance of abiotic hydrocarbon production under hydrothermal conditions into question, due to sluggish reaction rates (McCollom, 2016).
View in article
Pasquier-Cardin, A., Allard, P., Ferreira, T., Hatte, C., Coutinho, R., Fontugne, M., Jaudon, M. (1999) Magma-derived CO2 emissions recorded in 14C and 13C content of plants growing in Furnas caldera, Azores. Journal of Volcanology and Geothermal Research 92, 195–207.

For Esguicho (Furnas village) δ13C-C2+ of –28 to –29 ‰ perfectly agree with the average carbon isotopic composition of terrestrial plants growing in the Furnas caldera (–28.4 ‰; Pasquier-Cardin et al., 1999).
View in article
Proskurowski, G., Lilley, M.D., Seewald, J.S., Früh-Green, G.L., Olson, E.J., Lupton, J.E., Sylva, S.P., Kelley, D.S. (2008) Abiotic hydrocarbon production at Lost City hydrothermal field. Science 319, 604–607.

Most prominently, the following criteria have been used to identify abiotic hydrocarbon occurrences: i) methane with δ13C ≥ –20 ‰ (e.g., Welhan and Craig, 1979), ii) the occurrence of a carbon isotope reversal between ethane and methane (i.e. methane more enriched in 13C than ethane, contrary to what is observed for n-alkanes from confined sedimentary hydrocarbon reservoirs) (e.g., Des Marais et al., 1981; Sherwood Lollar et al., 2002; Proskurowski et al., 2008) and iii) methane in apparent chemical and isotopic equilibrium with inorganically derived CO2 (Fiebig et al., 2007).
View in article
Quigley, T.M., MacKenzie, A.S. (1988) The temperatures of oil and gas formation in the sub-surface. Nature 333, 549–552.

The observed 13C-enrichment of thermogenic methane in volcanic-hydrothermal fluids may, therefore, result from relatively high reservoir temperatures of 200 - 450 °C (Table S-1), well-exceeding those of confined sedimentary reservoirs where methane generation takes place primarily between ~150 - 220 °C (Quigley and MacKenzie, 1988).
View in article
Sara, G., De Pirro, M., Romano, C., Rumolo, P., Sprovieri, M., Mazzola, A. (2007) Sources of organic matter for intertidal consumers on Ascophyllum-shores (SW Iceland): a multi-stable isotope approach. Helgoland Marine Research 61, 297–302.

At Reykjanes, δ13C-C2+ of –16 ‰ (well 11) and –17 to –18 ‰ (well 12) overlap with the carbon isotopic composition of particulate organic carbon (–17.5 ‰ ≥ δ13C-POC ≥ –22.2 ‰) and modern sedimentary organic matter (–16.5 ‰ ≥ δ13C-SOM ≥ –19.4 ‰) that are characteristic of the water masses surrounding the Reykjanes peninsula (Sara et al., 2007).
View in article
Figure 1 [...] δ13C ranges of modern marine organic matter (Druffel et al., 1992; Sara et al., 2007), terrestrial C3 vegetation (Kohn, 2010) and Archean organic matter (Hayes and Waldbauer, 2006) are shown for comparison.
View in article
Although the majority of sampled discharges exhibit significant differences among δ13C-C2+ values (Tables S-2, S-3), the carbon isotopic composition of n-butane, the longest n-alkane analysed in this study, consistently occurs within the range reported for modern marine dissolved organic carbon (–18 ‰ ≥ δ13C-DOC ≥ –23 ‰; Druffel et al., 1992), modern marine particulate organic carbon (–17 ‰ ≥ δ13C-POC ≥ –25 ‰; Druffel et al., 1992; Sara et al., 2007) and modern terrestrial C3 plants (–20 ‰ ≥ δ13C ≥ –37 ‰, with most data clustering between –23 ‰ ≥ δ13C ≥ –31.5 ‰ and averaging at δ13C = –28.5 ‰; Kohn, 2010) (Fig. 1b).
View in article
Schoell, M. (1984) Wasserstoff- und Kohlenstoffisotope in organischen Substanzen, Erdölen und Erdgasen. Geologisches Jahrbuch D67, 1–161.

Figure 2 [...] Blue and green squares are representative of the carbon and hydrogen isotopic compositions of marine organic matter and C3 plants, respectively (Schoell, 1984).
View in article
Schoell, M. (1988) Multiple origins of methane in the Earth. Chemical Geology 71, 1–10.

Figure 2 [...] Field characteristic for methane from microbial (c) and confined sedimentary systems (b, c) redrawn after Schoell (1988).
View in article
Sherwood Lollar, B., Westgate, T.D., Ward, J.A., Slater, G.F., Lacrampe-Couloume, G. (2002) Abiotic formation of gaseous alkanes in the Earth’s crust as a minor source of global hydrocarbon reservoirs. Nature 416, 522–524.

Most prominently, the following criteria have been used to identify abiotic hydrocarbon occurrences: i) methane with δ13C ≥ –20 ‰ (e.g., Welhan and Craig, 1979), ii) the occurrence of a carbon isotope reversal between ethane and methane (i.e. methane more enriched in 13C than ethane, contrary to what is observed for n-alkanes from confined sedimentary hydrocarbon reservoirs) (e.g., Des Marais et al., 1981; Sherwood Lollar et al., 2002; Proskurowski et al., 2008) and iii) methane in apparent chemical and isotopic equilibrium with inorganically derived CO2 (Fiebig et al., 2007).
View in article
Still, C.J., Berry, J.A., Collatz, G.J., DeFries, R.S. (2003) Global distribution of C3 and C4 vegetation: Carbon cycle implications. Global Biogeochemical Cycles 17, doi: 10.1029/2001GB001807.

Terrestrial vegetation at the sampled sites is largely dominated by C3 plants (Still et al., 2003).
View in article
Tang, Y., Perry, J.K., Jenden, P.D., Schoell, M. (2000) Mathematical modeling of stable carbon isotope ratios in natural gases. Geochimica et Cosmochimica Acta 64, 2673–2687.

Both patterns are consistent with isotope fractionation principles of organic matter degradation according to which the carbon isotope fractionation between source organic matter and the evolving gaseous n-alkane decreases with the number of carbon atoms constituting the n-alkane (Tang et al., 2000).
View in article
Generally, the magnitude of carbon isotope fractionation between precursor organic matter and evolving n-alkane decreases with increasing temperature (e.g., Tang et al., 2000).
View in article
Taran, Y.A., Kliger, G.A., Sevastyanov, V.S. (2007) Carbon isotope effects in the open-system Fischer–Tropsch synthesis. Geochimica et Cosmochimica Acta 71, 4474–4487.

Reversals can be obtained from thermogenic degradation and open system degassing alone (Fig. S-3), or from mixing of thermogenic n-alkanes from two or more sources of distinct organic maturity (Taran et al., 2007).
View in article
Welhan, J.A., Craig, H. (1979) Methane and hydrogen in East Pacific Rise hydrothermal fluids. Geophysical Research Letters 6, 829–831.

Most prominently, the following criteria have been used to identify abiotic hydrocarbon occurrences: i) methane with δ13C ≥ –20 ‰ (e.g., Welhan and Craig, 1979), ii) the occurrence of a carbon isotope reversal between ethane and methane (i.e. methane more enriched in 13C than ethane, contrary to what is observed for n-alkanes from confined sedimentary hydrocarbon reservoirs) (e.g., Des Marais et al., 1981; Sherwood Lollar et al., 2002; Proskurowski et al., 2008) and iii) methane in apparent chemical and isotopic equilibrium with inorganically derived CO2 (Fiebig et al., 2007).
View in article
Whiticar, M.J. (1999) Carbon and hydrogen isotope systematics of bacterial formation and oxidation of methane. Chemical Geology 161, 291–314.

First, even if methane samples with an obvious microbial origin (Furnas B and Furnas Lake 2, Azores; Table S-3), as indicated by relatively strong depletions in 13C and 2H; Whiticar et al., 1999) are excluded, methane still exhibits the largest variations in δ13C of all analysed n-alkanes (Fig. 1b).
View in article
top
Supplementary Information
The Supplementary Information includes:
- Methods
- Sampled Locations
- Data Modelling
- Additional Data (Displayed in Figures)
- Tables S-1 to S-3
- Figures S-1 to S-4
- Supplementary Information References
Download the Supplementary Information (PDF).
Figures and Tables

Figure 1 Plot of δ13C of individual n-alkanes against carbon number. δ13C ranges of modern marine organic matter (Druffel et al., 1992
Druffel, E.R.M., Williams, P.M., Bauer, J.E., Ertel, J.R. (1992) Cycling of dissolved and particulate organic matter in the open ocean. Journal of Geophysical Research 97, 15639–15659.
; Sara et al., 2007Sara, G., De Pirro, M., Romano, C., Rumolo, P., Sprovieri, M., Mazzola, A. (2007) Sources of organic matter for intertidal consumers on Ascophyllum-shores (SW Iceland): a multi-stable isotope approach. Helgoland Marine Research 61, 297–302.
), terrestrial C3 vegetation (Kohn, 2010Kohn, M.J. (2010) Carbon isotope compositions of terrestrial C3 plants as indicators of (paleo)ecology and (paleo)climate. Proceedings of the National Academy of Sciences of the USA 107, 19691–19695.
) and Archean organic matter (Hayes and Waldbauer, 2006Hayes, J.M., Waldbauer, J.R. (2006) The carbon cycle and associated redox processes through time. Philosophical Transactions of the Royal Society B 361, 931–950.
) are shown for comparison. (a) Emissions that are characterised by invariant δ13C-C2+. (b) Compilation of all n-alkane data analysed in this study. (c) Comparison between n-alkane data from this study (area in grey) and data available from abiotic sites (Supplementary Information): hydrothermal sites (blue); ophiolite gases (black); old craton gases (red) and inclusions in igneous rocks (green).
Figure 2 Plot of δ2H-CH4 vs. δ13C-CH4. Samples with an obvious microbial origin (δ13C-CH4 < –60 ‰, Fig. 1b) are not considered. (a) Data classified after the origin of external water feeding the hydrothermal system (Table S-1). Blue and green squares are representative of the carbon and hydrogen isotopic compositions of marine organic matter and C3 plants, respectively (Schoell, 1984
Schoell, M. (1984) Wasserstoff- und Kohlenstoffisotope in organischen Substanzen, Erdölen und Erdgasen. Geologisches Jahrbuch D67, 1–161.
). The carbon and hydrogen isotopic compositions of instantaneously generated fractions of methane deriving from open system cracking of marine and terrestrial (C3 plants) organic matter were modelled as a function of the fraction of precursor sites remaining inside the cracked organic matter (Supplementary Information). The cracking trend for methane deriving from marine organic matter (blue line) matches the variation of δ13C-CH4 and δ2H-CH4 observed for seawater-fed hydrothermal systems (blue data points). The cracking trend for methane from terrestrial organic matter (green line) corresponds to the slope described by most low δ13C-CH4 samples from meteoric water-fed hydrothermal systems (green data points), but – on average – occurs shifted relative to the latter to higher δ13C and δ2H. This implies that methane precursor sites in decomposing terrestrial organic matter either occur depleted in 13C and 2H with respect to the average C3 plant isotopic composition or that the corresponding carbon and hydrogen isotope fractionations (αC, αH) are larger than those obtained from xylite (Berner et al., 1995Berner, U., Faber, E., Scheeder, G., Panten, D. (1995) Primary cracking of algal and landplant kerogens: kinetic models of isotope variations in methane, ethane and propane. Chemical Geology 126, 233–245.
), with αH /αC remaining unchanged. Both possibilities are consistent with carbon isotope constraints on pyrolysis of coal (Cramer et al., 1998Cramer, B., Krooss, B.M., Littke, R. (1998) Modelling isotope fractionation during primary cracking of natural gas: a reaction kinetic approach. Chemical Geology 149, 235–250.
). (b) Data classified after the style of degassing (wells vs. fumaroles). (c) Comparison between methane data from this study and data available from other abiotic sites (Supplementary Information): labelling as in Figure 1c, extended by (hyper)alkaline spring data (open symbols). Field characteristic for methane from microbial (c) and confined sedimentary systems (b, c) redrawn after Schoell (1988)Schoell, M. (1988) Multiple origins of methane in the Earth. Chemical Geology 71, 1–10.
.