Carbon and hydrogen isotope fractionation in lipid biosynthesis by Sporosarcina sp. DSK25
Affiliations | Corresponding Author | Cite as | Funding information-
Share this article
Article views:54Cumulative count of HTML views and PDF downloads.
- Download Citation
-
Rights & Permissions
top
Abstract
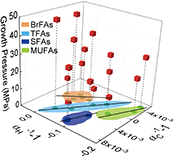
Figures and Tables
![]() Figure 1 Growth curve of Sporosarcina sp. DSK25 under different pressures. |
![]() Figure 2 Carbon and hydrogen isotope fractionation of Sporosarcina sp. DSK25 changes with different growth pressures. |
![]() Table 1 (a) Abundance (in percentage) of fatty acids extracted from Sporosarcina sp. DSK25 grown under different pressures. (b) δ13C values (‰) of fatty acids isolated from Sporosarcina sp. DSK25 grown under different pressures. (c) δD values (‰) of fatty acids isolated from Sporosarcina sp. DSK25 grown under different pressures. |
![]() Figure 3 Plot of hydrogen versus carbon isotope fractionation factors observed in lipid biosynthesis by Sporosarcina sp. DSK25 grown at pressures of 0.1, 10, 20, 30, 40 and 50 MPa. The slope of the regression curves gives the lambda (Λ) values. TFAs: total fatty acids; MUFAs: monounsaturated fatty acids; SFAs: saturated fatty acids; BrFAs: branched-chain fatty acids; αH: fractionation factor of hydrogen isotope, αC: fractionation factor of carbon isotope. |
Figure 1 | Figure 2 | Table 1 | Figure 3 |
top
Introduction
δ13C and δD of lipids have been widely utilised as proxies in studying marine biogeochemical cycles (Li et al., 2009
Li, C., Sessions, A.L., Kinnaman, F.S., Valentine, D.L. (2009) Hydrogen-isotopic variability in lipids from Santa Barbara Basin sediments. Geochimica et Cosmochimica Acta 73, 4803–4823.
), apportioning sources of marine organic matter (Chikaraishi et al., 2005Chikaraishi, Y., Yamada, Y., Naraoka, H. (2005) Carbon and hydrogen isotopic compositions of sterols from riverine and marine sediments. Limnology and Oceanography 50, 1763–1770.
), determining microbial metabolisms (Kümmel et al., 2016Kümmel, S., Starke, R., Chen, G., Musat, F., Richnow, H.H., Vogt, C. (2016) Hydrogen isotope fractionation as a tool to identify aerobic and anaerobic PAH biodegradation. Environmental Science and Technology 50, 3091–3100.
; Wijker et al., 2019Wijker, R.S., Sessions, A.L., Fuhrer, T., Phan, M. (2019) 2H/1H variation in microbial lipids is controlled by NADPH metabolism. Proceedings of the National Academy of Sciences 116, 12173–12182.
), reconstructing palaeoenvironments (Meyers, 1997Meyers, P.A. (1997) Organic geochemical proxies of paleoceanographic, paleolimnologic, and paleoclimatic processes. Organic Geochemistry 27, 213–250.
) and tracing ancient biogeochemical processes in the geologic record (Sessions et al., 2004Sessions, A.L., Sylva, S.P., Summons, R.E., Hayes, J.M. (2004) Isotopic exchange of carbonbound hydrogen over geologic timescales. Geochimica et Cosmochimica Acta 68, 1545–1559.
). However, using δD or δ13C values alone may be inconclusive or cause confusion or even errors because of the large variability in δ13C and δD of microbial lipids. The latter, for example, can vary up to 400 ‰ for microbial lipids (Wijker et al., 2019Wijker, R.S., Sessions, A.L., Fuhrer, T., Phan, M. (2019) 2H/1H variation in microbial lipids is controlled by NADPH metabolism. Proceedings of the National Academy of Sciences 116, 12173–12182.
), depending on the type of microbial metabolism (Zhang et al., 2009Zhang, X., Gillespie, A.L., Sessions, A.L. (2009) Large D/H variations in bacterial lipids reflect central metabolic pathways. Proceedings of the National Academy of Sciences 106, 12580–12586.
), lipid biosynthetic pathway (Fang et al., 2006Fang, J., Uhle, M., Billmark, K., Bartlett, D.H., Kato, C. (2006) Fractionation of carbon isotopes in biosynthesis of fatty acids by a piezophilic bacterium Moritella japonica strain DSK1. Geochimica et Cosmochimica Acta 70, 1753–1760.
, 2014Fang, J., Li, C., Zhang, L., Davis, T., Kato, C., Bartlett, D.H. (2014) Hydrogen isotope fractionation in lipid biosynthesis by the piezophilic bacterium Moritella japonica DSK1. Chemical Geology 367, 34–38.
) and environmental factors. For instance, Fang et al. (2006)Fang, J., Uhle, M., Billmark, K., Bartlett, D.H., Kato, C. (2006) Fractionation of carbon isotopes in biosynthesis of fatty acids by a piezophilic bacterium Moritella japonica strain DSK1. Geochimica et Cosmochimica Acta 70, 1753–1760.
showed that fatty acid biomarkers with highly negative δ13C values may be derived from marine bacterial secondary metabolism, not from bacterial utilisation of a 13C-depleted carbon source (e.g., methane), because carbon isotope fractionation in the biosynthesis of lipids is correlated with bacterial growth pressure. In a recent study, Miller et al. (2018)Miller, H.M., Chaudhry, N., Conrad, M.E., Bill, M., Kopf, S.H., Templeton, A.S. (2018) Large carbon isotope variability during methanogenesis under alkaline conditions. Geochimica et Cosmochimica Acta 237, 18–31.
observed large variations in isotope fractionation during methanogenesis under alkaline conditions, where carbon isotope fractionation increased with pH, reaching a maximum 13C depletion of −85 ‰, whereas hydrogen isotope fractionation did not vary with pH. Thus, the two dimensional compound specific isotope analysis approach, employing δ13C and δD simultaneously, has been favourably used in distinguishing biotic from abiotic processes, or identifying aerobic and anaerobic metabolism (Feisthauer et al., 2011Feisthauer, S., Vogt, C., Modrzynski, J., Szlenkier, M., Krüger, M., Siegert, M., Richnow, H.H. (2011) Different types of methane monooxygenases produce similar carbon and hydrogen isotope fractionation patterns during methane oxidation. Geochimica et Cosmochimica Acta 75, 1173–1184.
; Kümmel et al., 2016Kümmel, S., Starke, R., Chen, G., Musat, F., Richnow, H.H., Vogt, C. (2016) Hydrogen isotope fractionation as a tool to identify aerobic and anaerobic PAH biodegradation. Environmental Science and Technology 50, 3091–3100.
; Musat et al., 2016Musat, F., Vogt, C., Richnow, H.H. (2016) Carbon and hydrogen stable isotope fractionation associated with the aerobic and anaerobic degradation of saturated and alkylated aromatic hydrocarbons. Journal of Molecular Microbiology and Biotechnology 26, 211–226.
), among other applications. For example, the combined use of carbon and hydrogen isotope analyses allowed Feisthauer et al. (2011)Feisthauer, S., Vogt, C., Modrzynski, J., Szlenkier, M., Krüger, M., Siegert, M., Richnow, H.H. (2011) Different types of methane monooxygenases produce similar carbon and hydrogen isotope fractionation patterns during methane oxidation. Geochimica et Cosmochimica Acta 75, 1173–1184.
to monitor and assess the occurrence of microbial methane oxidation in the marine and terrestrial environments.Additionally, the current stable isotope geochemical theory, established based on surface bacteria rather than deep sea piezophilic bacteria, is not applicable to studying deep ocean biogeochemistry because of the pressure induced changes in microbial physiology and isotope fractionation (Fang et al., 2006
Fang, J., Uhle, M., Billmark, K., Bartlett, D.H., Kato, C. (2006) Fractionation of carbon isotopes in biosynthesis of fatty acids by a piezophilic bacterium Moritella japonica strain DSK1. Geochimica et Cosmochimica Acta 70, 1753–1760.
). However, previous studies focused mainly on investigating carbon and hydrogen isotope fractionations in lipid biosynthesis by gram-negative piezophilic bacteria (Fang et al., 2006Fang, J., Uhle, M., Billmark, K., Bartlett, D.H., Kato, C. (2006) Fractionation of carbon isotopes in biosynthesis of fatty acids by a piezophilic bacterium Moritella japonica strain DSK1. Geochimica et Cosmochimica Acta 70, 1753–1760.
, 2014Fang, J., Li, C., Zhang, L., Davis, T., Kato, C., Bartlett, D.H. (2014) Hydrogen isotope fractionation in lipid biosynthesis by the piezophilic bacterium Moritella japonica DSK1. Chemical Geology 367, 34–38.
), and there have been no reports of similar studies on gram-positive piezophilic bacteria. Yet, gram-positive bacteria likely play a similarly important role as gram-negative bacteria in mediating biogeochemical processes, especially in the deep ocean and the deep biosphere (Fang et al., 2017Fang, J., Kato, C., Runko, G.M., Nogi, Y., Hori, T., Li, J., Morono, Y., Inagaki, F. (2017) Predominance of viable spore-forming piezophilic bacteria in high-pressure enrichment cultures from ∼1.5 to 2.4 km-deep coal-bearing sediments below the ocean floor. Frontiers in Microbiology 8, 137.
).Motivated by these concerns, we designed experiments aiming to quantify carbon and hydrogen isotope fractionation simultaneously in lipid biosynthesis by the gram-positive piezotolerant bacterium Sporosarcina sp. DSK25. Results from this study allow us to derive the lambda index for deciphering microbially mediated biogeochemical processes in pressured environments of the deep ocean and the deep biosphere.
top
Materials and Methods
Sporosarcina sp. DSK25 is a piezotolerant bacterium isolated from the Japan Trench at a depth of 6500 m (Kato et al., 1995
Kato, C., Suzuki, S., Hata, S., Ito, T., Horikoshi, K. (1995) The properties of a protease activated by high pressure from Sporosarcina sp. strain DSK25 isolated from deep-sea sediment. JAMSTECR 32, 7–13.
). DSK25 was grown in custom-built high pressure vessels (Fang et al., 2006Fang, J., Uhle, M., Billmark, K., Bartlett, D.H., Kato, C. (2006) Fractionation of carbon isotopes in biosynthesis of fatty acids by a piezophilic bacterium Moritella japonica strain DSK1. Geochimica et Cosmochimica Acta 70, 1753–1760.
). Growth medium was prepared using sterile filtered natural seawater (Sigma-Aldrich Co.) with glucose as the sole carbon source, supplemented with yeast extract (0.04 %, Supplementary Information, SI). The medium was distributed into airtight pouches, and then incubated in high pressure vessels at 35 °C (optimal growth temperature of the strain) and pressures of 0.1, 10, 20, 30, 40 and 50 MPa (SI). Cultures were removed at the stationary phase (Fig. 1). Cell pellets were collected after centrifugation.Bacterial lipids were extracted using a one phase solvent system containing dichloromethane, methanol, and phosphate buffer (Fang and Findlay, 1996
Fang, J., Findlay, R.H. (1996) The use of a classic lipid extraction method for simultaneous recovery of organic pollutants and microbial lipids from sediments. Journal of Microbiological Methods 27, 63–71.
). Intact polar membrane lipids (IPLs) were obtained from the total lipids by using miniature columns containing 100 mg silicic acid, eluted with 5 mL of methanol, followed by a trans-methylation procedure to produce fatty acid methyl esters (FAMEs) (Fang and Findlay, 1996Fang, J., Findlay, R.H. (1996) The use of a classic lipid extraction method for simultaneous recovery of organic pollutants and microbial lipids from sediments. Journal of Microbiological Methods 27, 63–71.
). FAMEs were analysed using gas chromatography mass spectrometry. The δD and δ13C of FAMEs were determined by an isotope ratio mass spectrometer (Fang et al., 2006Fang, J., Uhle, M., Billmark, K., Bartlett, D.H., Kato, C. (2006) Fractionation of carbon isotopes in biosynthesis of fatty acids by a piezophilic bacterium Moritella japonica strain DSK1. Geochimica et Cosmochimica Acta 70, 1753–1760.
, 2014Fang, J., Li, C., Zhang, L., Davis, T., Kato, C., Bartlett, D.H. (2014) Hydrogen isotope fractionation in lipid biosynthesis by the piezophilic bacterium Moritella japonica DSK1. Chemical Geology 367, 34–38.
). δ notion was given by:
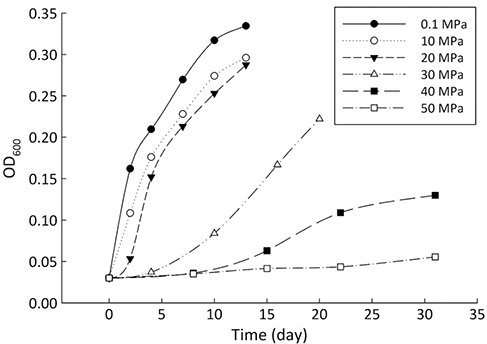
Figure 1 Growth curve of Sporosarcina sp. DSK25 under different pressures.
top
Results
DSK25 synthesised saturated (SFAs), monounsaturated (MUFAs) and branched chain fatty acids (BrFAs). The three groups of FAs constitute 35.6, 38.0 and 26.4 % of the total fatty acids (TFAs), respectively (Table 1a; Fig. S-2). This lipid profile is similar to that observed in gram-positive non-piezophilic bacteria grown at atmospheric pressure, except that higher proportions of BrFAs were observed in DSK25 (e.g., Kaneda, 1991
Kaneda, T.O.S.H.I. (1991) Iso-and anteiso-fatty acids in bacteria: biosynthesis, function, and taxonomic significance. Microbiological Reviews 55, 288–302.
). BrFAs had the most depleted δ13C values, −29.9 ‰, abundance weighted averaging (awa) over all growth pressures (Table 1b), whereas straight chain fatty acids (SCFAs) had relatively higher δ13C values (awa): −24.2 and −19.3 ‰ for SFAs and MUFAs (Table 1b), respectively. Yet C16:0 and C16:1 were more depleted in 13C than BrFAs at high pressures (e.g., 40 and 50 MPa).The calculated carbon isotopic enrichment factor, ɛ, showed a strong correlation with pressure, with R2 of 0.75 (p < 0.1) and 0.84 (p < 0.02) for SFAs and MUFAs, respectively (Fig. 2). A progressive increase in ɛ with pressure was observed, as evidenced by ɛfa/g, −12.6, −16.2, −15.3, −16.2, −21.0 and −22.6 ‰ at 0.1, 10, 20, 30, 40 and 50 MPa, respectively. A linear correlation was observed between δ13C and FAs carbon chain length (p < 0.1, Fig. S-1).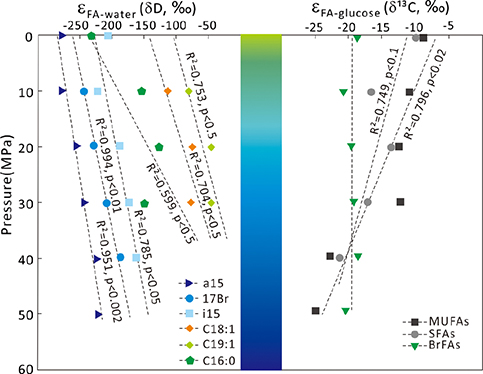
Figure 2 Carbon and hydrogen isotope fractionation of Sporosarcina sp. DSK25 changes with different growth pressures.
Table 1 (a) Abundance (in percentage) of fatty acids extracted from Sporosarcina sp. DSK25 grown under different pressures. (b) δ13C values (‰) of fatty acids isolated from Sporosarcina sp. DSK25 grown under different pressures. (c) δD values (‰) of fatty acids isolated from Sporosarcina sp. DSK25 grown under different pressures.
(a) | ||||||
Compound | Pressure (MPa) | |||||
0.1 | 10 | 20 | 30 | 40 | 50 | |
i15:0a | 4.63 | 6.69 | 7.30 | 5.74 | 10.54 | 5.19 |
a15:0 | 16.09 | 14.60 | 18.93 | 20.96 | 56.41 | 69.99 |
C16:0 | 29.93 | 20.36 | 6.28 | 5.86 | 2.75 | 5.49 |
C16:1 | 7.10 | - | - | - | - | - |
C17:0 | - | 1.22 | - | - | - | - |
C17:1 | 9.15 | 8.90 | - | - | - | - |
i17:0 | 1.33 | - | - | 2.00 | 4.43 | 2.24 |
a17:0 | - | - | 2.13 | - | 1.92 | - |
17:0br
|
6.65 | 6.26 | 10.29 | 8.90 | 23.95 | 17.09 |
C18:1 | 13.63 | 22.94 | 40.69 | 36.17 | - | - |
C19:1 | 11.49 | 19.03 | 14.38 | 20.37 | - | - |
(b) | ||||||
Compound | Pressure (MPa) | |||||
0.1 | 10 | 20 | 30 | 40 | 50 | |
i15:0 | −29.3 | −29.7 | −28.5 | −29.0 | −28.2 | −30.1 |
a15:0 | −29.9 | −30.5 | −29.2 | −30.0 | −28.7 | −30.7 |
C16:0 | −20.6 | −27.3 | −24.3 | −27.7 | −32.0 | - |
C16:1 | −21.2 | −19.4 | −31.6 | −35.0 | −33.4 | −35.7 |
i16:0 | - | −30.1 | - | - | - | - |
a16:0 | - | −32.4 | - | - | - | - |
C17:1 | −20.1 | −26.9 | - | - | - | - |
a17:1 | - | - | - | - | - | −34.7 |
i17:0 | −30.8 | −34.2 | −31.8 | −31.8 | −30.7 | - |
a17:0 | −27.8 | −32.0 | −31.8 | −28.6 | −29.7 | −32.7 |
C18:1 | −19.4 | −19.4 | −17.1 | −15.7 | - | - |
C19:1 | −18.4 | −20.6 | −20.6 | −17.9 | - | - |
(c) | ||||||
Compound | Pressure (MPa) | |||||
0.1 | 10 | 20 | 30 | 40 | 50 | |
i15:0 | −188.6 | −203.6 | −174.1 | −158.8 | −150.5 | - |
a15:0 | −254.9 | −255.5 | −234.7 | −224.1 | −205.7 | −203.1 |
C16:0 | −213.4 | −141.6 | −117.0 | −137.3 | - | - |
C16:1 | −226.9 | - | - | - | - | - |
C17:1 | - | −127.8 | - | - | - | - |
i17:0 | - | - | - | - | −167.1 | - |
17:0br | - | −223.2 | −210.0 | −191.9 | −173.2 | - |
C18:1 | - | −104.7 | −68.8 | −70.9 | - | - |
C19:1 | - | −74.2 | −41.9 | −41.7 | - | - |
-Fatty acids that were either absent or at a too low concentration for a reliable determination of δD or δ13C values.
*Methyl branched fatty acid. The position of the methyl group was not identified on GC-MS.
Similarly, BrFAs were more depleted in D compared to MUFAs and SFAs under all pressures (Table 1c) (p < 0.05 between BrFAs and SFAs), except that C16:0 and C16:1 were more depleted in D than i15:0 under atmospheric pressure. The δD values (awa) for SFAs, MUFAs and BrFAs were −173.2, −76.9 and −196.1 ‰, respectively. Average hydrogen isotope fractionation for SFAs, MUFAs and BrFAs was −165.7, −72.8, and −222.0 ‰ relative to growth water, and −155.3, −61.1, and −212.2 ‰ relative to glucose. A strong negative linear correlation was observed between hydrogen isotope fractionation and bacterial growth pressure (Fig. 2), with R2 values of 0.99 (p < 0.01), 0.95 (p < 0.002), 0.79 (p < 0.05), 0.75 (p < 0.5) and 0.70 (p < 0.5) for 17:0br, a15:0, i15:0, C19:1 and C18:1, respectively. A strong linear correlation between δD values and FAs carbon chain length was also observed (P < 0.01, Fig. S-1).
top
Discussion
Pressure dependent carbon and hydrogen isotope fractionation in lipid biosynthesis. The deep ocean and the deep biosphere are the most extensive pressured habitat on Earth, hydrostatic pressure ranges over four or more orders of magnitude (Bartlett and Welch, 1995
Bartlett, D.H., Welch, T.J. (1995) ompH gene expression is regulated by multiple environmental cues in addition to high pressure in the deep-sea bacterium Photobacterium species strain SS9. Journal of Bacteriology 177, 1008–1016.
). Pressure affects microbial physiology, metabolism and carbon isotope fractionation in the biosynthesis of lipids (Fang et al., 2006Fang, J., Uhle, M., Billmark, K., Bartlett, D.H., Kato, C. (2006) Fractionation of carbon isotopes in biosynthesis of fatty acids by a piezophilic bacterium Moritella japonica strain DSK1. Geochimica et Cosmochimica Acta 70, 1753–1760.
, 2014Fang, J., Li, C., Zhang, L., Davis, T., Kato, C., Bartlett, D.H. (2014) Hydrogen isotope fractionation in lipid biosynthesis by the piezophilic bacterium Moritella japonica DSK1. Chemical Geology 367, 34–38.
). Thus, Fang et al. (2010)Fang, J., Zhang, L., Bazylinski, D.A. (2010) Deep-sea piezosphere and piezophiles: geomicrobiology and biogeochemistry. Trends in Microbiology 18, 413–422.
suggested establishing a new field of geochemistry: high pressure microbial isotope geochemistry.Our results show that, similar to their gram-negative counterpart (Fang et al., 2006
Fang, J., Uhle, M., Billmark, K., Bartlett, D.H., Kato, C. (2006) Fractionation of carbon isotopes in biosynthesis of fatty acids by a piezophilic bacterium Moritella japonica strain DSK1. Geochimica et Cosmochimica Acta 70, 1753–1760.
), the deep sea gram-positive piezotolerant bacterium DSK25 exhibited pressure dependent carbon isotope fractionation between fatty acids and glucose, with ɛ increasing with bacterial growth pressure. The kinetic carbon isotope effect, ɛPDH, is calculated as 25.2, 30.4, 34.5, 49.2 and 56.0 ‰ at 10, 20, 30, 40 and 50 MPa, respectively (see calculations presented in SI), suggesting a consistent pressure dependent carbon isotopic fractionation. However, the magnitude of carbon isotope fractionation observed here is much lower than that found in the gram-negative piezophilic bacterium DSK1, by 2.5 and 1.9 ‰ at 10 and 20 MPa, respectively (Fang et al., 2006Fang, J., Uhle, M., Billmark, K., Bartlett, D.H., Kato, C. (2006) Fractionation of carbon isotopes in biosynthesis of fatty acids by a piezophilic bacterium Moritella japonica strain DSK1. Geochimica et Cosmochimica Acta 70, 1753–1760.
). Such differences might be related to the physiological differences between the two groups of bacteria (gram-positive and -negative bacteria). We hypothesise that bacterial cell wall physiology and properties of the cell envelope affect isotope fractionation. Specifically, gram-positive bacteria, lacking the restrictive outer membrane, experience less substrate mass transfer limitation at cell membranes than gram-negative bacteria and therefore, less isotope fractionation. Additionally, microbial stress response and fitness optimisation may also play a role on carbon isotope fractionation (Fang et al., 2006Fang, J., Uhle, M., Billmark, K., Bartlett, D.H., Kato, C. (2006) Fractionation of carbon isotopes in biosynthesis of fatty acids by a piezophilic bacterium Moritella japonica strain DSK1. Geochimica et Cosmochimica Acta 70, 1753–1760.
). These hypotheses await to be tested in future studies.Compared with SCFAs, BrFAs have the most depleted δ13C and δD values (awa), except for the δ13C values of C16:0 and C16:1 under high pressures and δD values of the two fatty acids at atmospheric pressure (Tables 1b and 1c). It seems plausible that microbial growth pressure affects the biosynthesis and isotopic composition of BrFAs and SCFAs differently. One hypothesis is that, under high pressures, biosynthesis of BrFAs may compete with that of SCFAs for D-depleted substrates and may be biosynthesised prior to SCFAs, leading to the remaining substrate being enriched in D, and thus SCFAs enriched in D. Alternatively, this resulted from microbial utilisation of different energy metabolic pathways in growth and metabolism (Wijker et al., 2019
Wijker, R.S., Sessions, A.L., Fuhrer, T., Phan, M. (2019) 2H/1H variation in microbial lipids is controlled by NADPH metabolism. Proceedings of the National Academy of Sciences 116, 12173–12182.
). Despite the fact that both BrFAs and SCFAs are synthesised by the same biosynthetic pathway with almost the same enzymes (Fig. S-3) and that the mechanisms of lipid biosynthesis are strongly conserved across most bacteria phyla (Rock and Jackowski, 2002), the reducing power NADPH produced by different metabolic pathways retain different isotopic compositions, and consequently differing δD of fatty acids, as NADPH provides the largest source of hydrogen in microbial biosynthesis of fatty acids (Zhang et al., 2009Zhang, X., Gillespie, A.L., Sessions, A.L. (2009) Large D/H variations in bacterial lipids reflect central metabolic pathways. Proceedings of the National Academy of Sciences 106, 12580–12586.
; Wijker et al., 2019Wijker, R.S., Sessions, A.L., Fuhrer, T., Phan, M. (2019) 2H/1H variation in microbial lipids is controlled by NADPH metabolism. Proceedings of the National Academy of Sciences 116, 12173–12182.
; see discussion below).Hydrogen isotopic fractionations relative to growth water, ɛFA/w, and to glucose, ɛFA/g, are also pressure dependent, but in an inverse pattern (Table 1c, Fig. 2). Findings from this and previous works (Fang et al., 2006
Fang, J., Uhle, M., Billmark, K., Bartlett, D.H., Kato, C. (2006) Fractionation of carbon isotopes in biosynthesis of fatty acids by a piezophilic bacterium Moritella japonica strain DSK1. Geochimica et Cosmochimica Acta 70, 1753–1760.
, 2014Fang, J., Li, C., Zhang, L., Davis, T., Kato, C., Bartlett, D.H. (2014) Hydrogen isotope fractionation in lipid biosynthesis by the piezophilic bacterium Moritella japonica DSK1. Chemical Geology 367, 34–38.
) suggest that the pressure dependent fractionation of carbon and hydrogen isotopes may be a general trait for both gram-positive and -negative piezophiles in the deep ocean. Hydrogen isotope fractionation may be governed by pressure regulated kinetic isotope effects during lipid biosynthesis. Fang et al. (2014)Fang, J., Li, C., Zhang, L., Davis, T., Kato, C., Bartlett, D.H. (2014) Hydrogen isotope fractionation in lipid biosynthesis by the piezophilic bacterium Moritella japonica DSK1. Chemical Geology 367, 34–38.
suggested that lipid biosynthetic pathways (the PKS vs. the FAS pathway) and the pressure affected hydrogen tunneling are the determining factors in controlling δD of lipids biosynthesised by the piezophilic gram-negative bacterium DSK1 under high pressures. Zhang et al. (2009)Zhang, X., Gillespie, A.L., Sessions, A.L. (2009) Large D/H variations in bacterial lipids reflect central metabolic pathways. Proceedings of the National Academy of Sciences 106, 12580–12586.
showed that the δD signal of NADPH derived from different metabolic pathways may control the δD of microbial lipids, with NADPH from heterotrophic growth on the TCA-cycle yielding the most enriched lipid δD. Recently, Wijker et al. (2019)Wijker, R.S., Sessions, A.L., Fuhrer, T., Phan, M. (2019) 2H/1H variation in microbial lipids is controlled by NADPH metabolism. Proceedings of the National Academy of Sciences 116, 12173–12182.
found that the flux of NADPH through the TCA-cycle was strongly positively correlated with ɛL/W, producing the most positive fractionations. We hypothesise that the up-regulation of the TCA-cycle may occur at the expense of the PP and EMP pathways under high pressures, leading to D-enrichment of lipids. The exchange of hydrogen between NADPH and growth water, which could result in D enrichment in lipids, was studied in previous works (Zhang et al., 2009Zhang, X., Gillespie, A.L., Sessions, A.L. (2009) Large D/H variations in bacterial lipids reflect central metabolic pathways. Proceedings of the National Academy of Sciences 106, 12580–12586.
; Wijker et al., 2019Wijker, R.S., Sessions, A.L., Fuhrer, T., Phan, M. (2019) 2H/1H variation in microbial lipids is controlled by NADPH metabolism. Proceedings of the National Academy of Sciences 116, 12173–12182.
). For example, more H exchange between NADPH and extracellular water would happen in the TCA cycle than in other metabolic pathways (Sachs et al., 2016Sachs, J.P., Maloney, A.E., Gregersen, J., Paschall, C. (2016) Effect of salinity on 2H/1H fractionation in lipids from continuous cultures of the coccolithophorid Emiliania huxleyi. Geochimica et Cosmochimica Acta 189, 96–109.
and references therein). In addition, bacterial growth rate was quite low at high pressures (Fig. 1). Therefore, an alternative hypothesis is that the exchange of hydrogen between NADPH and growth water could result in D-enrichment in lipids under high growth pressures, where bacteria doubling time is prolonged.The δ13C and δD-derived lambda index. It is apparent that both carbon and hydrogen isotope fractionations are pressure dependent and that different groups of FAs exhibit distinct isotopic characteristics. Our results lead us to propose the lambda index (Λ), ratio of hydrogen versus carbon discrimination which can be calculated based on


Feisthauer, S., Vogt, C., Modrzynski, J., Szlenkier, M., Krüger, M., Siegert, M., Richnow, H.H. (2011) Different types of methane monooxygenases produce similar carbon and hydrogen isotope fractionation patterns during methane oxidation. Geochimica et Cosmochimica Acta 75, 1173–1184.
). Λ values of 11.3 ± 1.1, 7.9 ± 3.3 and 6.7 ± 2.0 were obtained for TFAs, MUFAs and SFAs, respectively (Fig. 3). Previous studies showed that the Λ index differed in aerobic and anaerobic microbiological processes (Dorer et al., 2014Dorer, C., Vogt, C., Kleinsteuber, S., Stams, A.J., Richnow, H.H. (2014) Compound-specific isotope analysis as a tool to characterize biodegradation of ethylbenzene. Environmental Science and Technology 48, 9122–9132.
). Feisthauer et al. (2011Feisthauer, S., Vogt, C., Modrzynski, J., Szlenkier, M., Krüger, M., Siegert, M., Richnow, H.H. (2011) Different types of methane monooxygenases produce similar carbon and hydrogen isotope fractionation patterns during methane oxidation. Geochimica et Cosmochimica Acta 75, 1173–1184.
) reported that the lambda values ranged from 7.3 ± 0.3 to 10.5 ± 0.5 for aerobic methane oxidising bacteria, significantly different from that for sulfate and nitrate reducing bacteria (Dorer et al., 2014Dorer, C., Vogt, C., Kleinsteuber, S., Stams, A.J., Richnow, H.H. (2014) Compound-specific isotope analysis as a tool to characterize biodegradation of ethylbenzene. Environmental Science and Technology 48, 9122–9132.
). Musat et al. (2016)Musat, F., Vogt, C., Richnow, H.H. (2016) Carbon and hydrogen stable isotope fractionation associated with the aerobic and anaerobic degradation of saturated and alkylated aromatic hydrocarbons. Journal of Molecular Microbiology and Biotechnology 26, 211–226.
used the Λ values to differentiate aerobic and anaerobic degradation processes of saturated and alkylated aromatic hydrocarbons.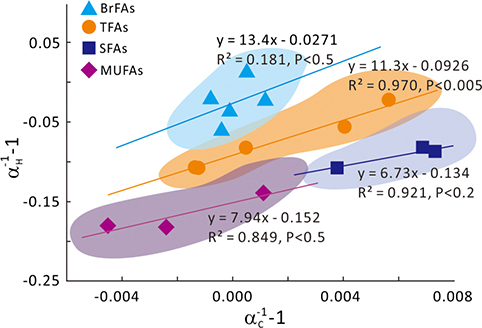
Figure 3 Plot of hydrogen versus carbon isotope fractionation factors observed in lipid biosynthesis by Sporosarcina sp. DSK25 grown at pressures of 0.1, 10, 20, 30, 40 and 50 MPa. The slope of the regression curves gives the lambda (Λ) values. TFAs: total fatty acids; MUFAs: monounsaturated fatty acids; SFAs: saturated fatty acids; BrFAs: branched-chain fatty acids; αH: fractionation factor of hydrogen isotope, αC: fractionation factor of carbon isotope.
Conceptually, carbon and hydrogen are inseparable atoms of lipid biomarkers, and would experience the same enzymatic reactions, but suffer from different hydrostatic pressure effects (e.g., hydrogen tunneling). An important observation of the present study is that bacterial growth pressure affects both




Kaneda, T.O.S.H.I. (1991) Iso-and anteiso-fatty acids in bacteria: biosynthesis, function, and taxonomic significance. Microbiological Reviews 55, 288–302.
; White et al., 1997White, D.C., Pinkart, H.C., Ringelberg, A.B. (1997) Biomass measurements: biochemical approaches. In: Hurst, C.J., Knudson, G.R., McInerney, M.J., Stetzenbach, L.D., Walter, M.V. (Eds.) Manual of Environmental Microbiology. ASM Press, Washington, D.C., 91–101.
) and certain sulfate reducing bacteria (Taylor and Parkes, 1983Taylor, J., Parkes, R.J. (1983) The cellular fatty acids of the sulphate-reducing bacteria, Desulfobacter sp., Desulfobulbus sp. and Desulfovibrio desulfuricans. Microbiology 129, 3303–3309.
), is different from that for MUFAs, abundant in gram-negative bacteria (White et al., 1997White, D.C., Pinkart, H.C., Ringelberg, A.B. (1997) Biomass measurements: biochemical approaches. In: Hurst, C.J., Knudson, G.R., McInerney, M.J., Stetzenbach, L.D., Walter, M.V. (Eds.) Manual of Environmental Microbiology. ASM Press, Washington, D.C., 91–101.
; Fang et al., 2006Fang, J., Uhle, M., Billmark, K., Bartlett, D.H., Kato, C. (2006) Fractionation of carbon isotopes in biosynthesis of fatty acids by a piezophilic bacterium Moritella japonica strain DSK1. Geochimica et Cosmochimica Acta 70, 1753–1760.
). However, it must be noted that there may be overlap in lipid biomarkers and lambda values between different groups of microbes. Therefore, caution is necessary when the lambda index is used to constrain microbes with different metabolisms. Nevertheless, the lambda values in this study are derived from bacteria grown at different pressures, fundamentally different from those reported in previous studies, and can therefore, be used for deciphering microbial metabolism and biogeochemical processes in pressured environments of the deep ocean and the deep biosphere. Future studies using δ13C and δD in investigating deep ocean microbial biogeochemical processes should take into consideration of hydrostatic pressure-induced changes in δ13C and δD.top
Conclusions
This study for the first time simultaneously quantified carbon and hydrogen isotope fractionations in the biosynthesis of fatty acids by a gram-positive piezotolerant bacterium, influenced by microbial physiology and growth pressure. Our results confirm the consistent pressure dependent fractionation of carbon and hydrogen isotopes, in both direction and magnitude. Our results suggest that pressure dependent isotope fractionation is a general feature of deep sea pressure-loving bacteria. We propose the dual isotope derived lambda index, calculated based on bacterial growth pressure for different groups of fatty acids biomarkers, can serve as a useful tool for constraining microbially-driven biogeochemical processes in the deep ocean and the deep biosphere. We hope that our approach will contribute to establishing high pressure isotope biogeochemistry, towards understanding of natural microbial biogeochemistry in pressured environments today and those in the geological past. Future culture-based research should be directed to studying the changing δ13C, δD and the lambda index of lipids for more deep sea microorganisms, associated with the shifting microbial metabolism and production of isotopically different NADPHs, under different hydrostatic pressures.
top
Acknowledgements
This research was supported by the National Natural Science Foundation of China (grant 41673085 to LZ, and 91951210 and 41773069 to JF) and by National Key R&D Program of China (grant 2018YFC0310600 to JF). We are grateful to editor of GPL and two anonymous reviewers whose insightful comments and suggestions greatly improved the manuscript.
Editor: Ariel Anbar
top
References
Bartlett, D.H., Welch, T.J. (1995) ompH gene expression is regulated by multiple environmental cues in addition to high pressure in the deep-sea bacterium Photobacterium species strain SS9. Journal of Bacteriology 177, 1008–1016.

The deep ocean and the deep biosphere are the most extensive pressured habitat on Earth, hydrostatic pressure ranges over four or more orders of magnitude (Bartlett and Welch, 1995).
View in article
Chikaraishi, Y., Yamada, Y., Naraoka, H. (2005) Carbon and hydrogen isotopic compositions of sterols from riverine and marine sediments. Limnology and Oceanography 50, 1763–1770.

δ13C and δD of lipids have been widely utilised as proxies in studying marine biogeochemical cycles (Li et al., 2009), apportioning sources of marine organic matter (Chikaraishi et al., 2005), determining microbial metabolisms (Kümmel et al., 2016; Wijker et al., 2019), reconstructing palaeoenvironments (Meyers, 1997) and tracing ancient biogeochemical processes in the geologic record (Sessions et al., 2004).
View in article
Dorer, C., Vogt, C., Kleinsteuber, S., Stams, A.J., Richnow, H.H. (2014) Compound-specific isotope analysis as a tool to characterize biodegradation of ethylbenzene. Environmental Science and Technology 48, 9122–9132.

Λ values of 11.3 ± 1.1, 7.9 ± 3.3 and 6.7 ± 2.0 were obtained for TFAs, MUFAs and SFAs, respectively (Fig. 3). Previous studies showed that the Λ index differed in aerobic and anaerobic microbiological processes (Dorer et al., 2014).
View in article
Fang, J., Findlay, R.H. (1996) The use of a classic lipid extraction method for simultaneous recovery of organic pollutants and microbial lipids from sediments. Journal of Microbiological Methods 27, 63–71.

Bacterial lipids were extracted using a one phase solvent system containing dichloromethane, methanol, and phosphate buffer (Fang and Findlay, 1996).
View in article
Fang, J., Uhle, M., Billmark, K., Bartlett, D.H., Kato, C. (2006) Fractionation of carbon isotopes in biosynthesis of fatty acids by a piezophilic bacterium Moritella japonica strain DSK1. Geochimica et Cosmochimica Acta 70, 1753–1760.

The latter, for example, can vary up to 400 ‰ for microbial lipids (Wijker et al., 2019), depending on the type of microbial metabolism (Zhang et al., 2009), lipid biosynthetic pathway (Fang et al., 2006, 2014) and environmental factors. For instance, Fang et al. (2006) showed that fatty acid biomarkers with highly negative δ13C values may be derived from marine bacterial secondary metabolism, not from bacterial utilisation of a 13C-depleted carbon source (e.g., methane), because carbon isotope fractionation in the biosynthesis of lipids is correlated with bacterial growth pressure.
View in article
Fang, J., Zhang, L., Bazylinski, D.A. (2010) Deep-sea piezosphere and piezophiles: geomicrobiology and biogeochemistry. Trends in Microbiology 18, 413–422.

Thus, Fang et al. (2010) suggested establishing a new field of geochemistry: high pressure microbial isotope geochemistry.
View in article
Fang, J., Li, C., Zhang, L., Davis, T., Kato, C., Bartlett, D.H. (2014) Hydrogen isotope fractionation in lipid biosynthesis by the piezophilic bacterium Moritella japonica DSK1. Chemical Geology 367, 34–38.

The latter, for example, can vary up to 400 ‰ for microbial lipids (Wijker et al., 2019), depending on the type of microbial metabolism (Zhang et al., 2009), lipid biosynthetic pathway (Fang et al., 2006, 2014) and environmental factors. For instance, Fang et al. (2006) showed that fatty acid biomarkers with highly negative δ13C values may be derived from marine bacterial secondary metabolism, not from bacterial utilisation of a 13C-depleted carbon source (e.g., methane), because carbon isotope fractionation in the biosynthesis of lipids is correlated with bacterial growth pressure.
View in article
Fang, J., Kato, C., Runko, G.M., Nogi, Y., Hori, T., Li, J., Morono, Y., Inagaki, F. (2017) Predominance of viable spore-forming piezophilic bacteria in high-pressure enrichment cultures from ∼1.5 to 2.4 km-deep coal-bearing sediments below the ocean floor. Frontiers in Microbiology 8, 137.

Yet, gram-positive bacteria likely play a similarly important role as gram-negative bacteria in mediating biogeochemical processes, especially in the deep ocean and the deep biosphere (Fang et al., 2017).
View in article
Feisthauer, S., Vogt, C., Modrzynski, J., Szlenkier, M., Krüger, M., Siegert, M., Richnow, H.H. (2011) Different types of methane monooxygenases produce similar carbon and hydrogen isotope fractionation patterns during methane oxidation. Geochimica et Cosmochimica Acta 75, 1173–1184.

Thus, the two dimensional compound specific isotope analysis approach, employing δ13C and δD simultaneously, has been favourably used in distinguishing biotic from abiotic processes, or identifying aerobic and anaerobic metabolism (Feisthauer et al., 2011; Kümmel et al., 2016; Musat et al., 2016), among other applications.
View in article
Kaneda, T.O.S.H.I. (1991) Iso-and anteiso-fatty acids in bacteria: biosynthesis, function, and taxonomic significance. Microbiological Reviews 55, 288–302.

This lipid profile is similar to that observed in gram-positive non-piezophilic bacteria grown at atmospheric pressure, except that higher proportions of BrFAs were observed in DSK25 (e.g., Kaneda, 1991).
View in article
Kato, C., Suzuki, S., Hata, S., Ito, T., Horikoshi, K. (1995) The properties of a protease activated by high pressure from Sporosarcina sp. strain DSK25 isolated from deep-sea sediment. JAMSTECR 32, 7–13.

Sporosarcina sp. DSK25 is a piezotolerant bacterium isolated from the Japan Trench at a depth of 6500 m (Kato et al., 1995).
View in article
Kümmel, S., Starke, R., Chen, G., Musat, F., Richnow, H.H., Vogt, C. (2016) Hydrogen isotope fractionation as a tool to identify aerobic and anaerobic PAH biodegradation. Environmental Science and Technology 50, 3091–3100.

δ13C and δD of lipids have been widely utilised as proxies in studying marine biogeochemical cycles (Li et al., 2009), apportioning sources of marine organic matter (Chikaraishi et al., 2005), determining microbial metabolisms (Kümmel et al., 2016; Wijker et al., 2019), reconstructing palaeoenvironments (Meyers, 1997) and tracing ancient biogeochemical processes in the geologic record (Sessions et al., 2004).
View in article
Li, C., Sessions, A.L., Kinnaman, F.S., Valentine, D.L. (2009) Hydrogen-isotopic variability in lipids from Santa Barbara Basin sediments. Geochimica et Cosmochimica Acta 73, 4803–4823.

δ13C and δD of lipids have been widely utilised as proxies in studying marine biogeochemical cycles (Li et al., 2009), apportioning sources of marine organic matter (Chikaraishi et al., 2005), determining microbial metabolisms (Kümmel et al., 2016; Wijker et al., 2019), reconstructing palaeoenvironments (Meyers, 1997) and tracing ancient biogeochemical processes in the geologic record (Sessions et al., 2004).
View in article
Meyers, P.A. (1997) Organic geochemical proxies of paleoceanographic, paleolimnologic, and paleoclimatic processes. Organic Geochemistry 27, 213–250.

δ13C and δD of lipids have been widely utilised as proxies in studying marine biogeochemical cycles (Li et al., 2009), apportioning sources of marine organic matter (Chikaraishi et al., 2005), determining microbial metabolisms (Kümmel et al., 2016; Wijker et al., 2019), reconstructing palaeoenvironments (Meyers, 1997) and tracing ancient biogeochemical processes in the geologic record (Sessions et al., 2004). However, using δD or δ13C values alone may be inconclusive or cause confusion or even errors because of the large variability in δ13C and δD of microbial lipids.
View in article
Miller, H.M., Chaudhry, N., Conrad, M.E., Bill, M., Kopf, S.H., Templeton, A.S. (2018) Large carbon isotope variability during methanogenesis under alkaline conditions. Geochimica et Cosmochimica Acta 237, 18–31.

In a recent study, Miller et al. (2018) observed large variations in isotope fractionation during methanogenesis under alkaline conditions, where carbon isotope fractionation increased with pH, reaching a maximum 13C depletion of −85 ‰, whereas hydrogen isotope fractionation did not vary with pH.
View in article
Musat, F., Vogt, C., Richnow, H.H. (2016) Carbon and hydrogen stable isotope fractionation associated with the aerobic and anaerobic degradation of saturated and alkylated aromatic hydrocarbons. Journal of Molecular Microbiology and Biotechnology 26, 211–226.

Thus, the two dimensional compound specific isotope analysis approach, employing δ13C and δD simultaneously, has been favourably used in distinguishing biotic from abiotic processes, or identifying aerobic and anaerobic metabolism (Feisthauer et al., 2011; Kümmel et al., 2016; Musat et al., 2016), among other applications. For example, the combined use of carbon and hydrogen isotope analyses allowed Feisthauer et al. (2011) to monitor and assess the occurrence of microbial methane oxidation in the marine and terrestrial environments.
View in article
Sachs, J.P., Maloney, A.E., Gregersen, J., Paschall, C. (2016) Effect of salinity on 2H/1H fractionation in lipids from continuous cultures of the coccolithophorid Emiliania huxleyi. Geochimica et Cosmochimica Acta 189, 96–109.

For example, more H exchange between NADPH and extracellular water would happen in the TCA cycle than in other metabolic pathways (Sachs et al., 2016 and references therein).
View in article
Sessions, A.L., Sylva, S.P., Summons, R.E., Hayes, J.M. (2004) Isotopic exchange of carbonbound hydrogen over geologic timescales. Geochimica et Cosmochimica Acta 68, 1545–1559.

δ13C and δD of lipids have been widely utilised as proxies in studying marine biogeochemical cycles (Li et al., 2009), apportioning sources of marine organic matter (Chikaraishi et al., 2005), determining microbial metabolisms (Kümmel et al., 2016; Wijker et al., 2019), reconstructing palaeoenvironments (Meyers, 1997) and tracing ancient biogeochemical processes in the geologic record (Sessions et al., 2004).
View in article
Taylor, J., Parkes, R.J. (1983) The cellular fatty acids of the sulphate-reducing bacteria, Desulfobacter sp., Desulfobulbus sp. and Desulfovibrio desulfuricans. Microbiology 129, 3303–3309.

For instance, Λ for BrFAs, biomarkers for gram-positive bacteria (Kaneda, 1991; White et al., 1997) and certain sulfate reducing bacteria (Taylor and Parkes, 1983), is different from that for MUFAs, abundant in gram-negative bacteria (White et al., 1997; Fang et al., 2006).
View in article
White, D.C., Pinkart, H.C., Ringelberg, A.B. (1997) Biomass measurements: biochemical approaches. In: Hurst, C.J., Knudson, G.R., McInerney, M.J., Stetzenbach, L.D., Walter, M.V. (Eds.) Manual of Environmental Microbiology. ASM Press, Washington, D.C., 91–101.

For one, the lambda index may serve as a useful tracer for determining the source of marine organic matter in the deep ocean. For the other, it has the potential to differentiate biogeochemical processes mediated by different groups of microorganisms. For instance, Λ for BrFAs, biomarkers for gram-positive bacteria (Kaneda, 1991; White et al., 1997) and certain sulfate reducing bacteria (Taylor and Parkes, 1983), is different from that for MUFAs, abundant in gram-negative bacteria (White et al., 1997; Fang et al., 2006).
View in article
Wijker, R.S., Sessions, A.L., Fuhrer, T., Phan, M. (2019) 2H/1H variation in microbial lipids is controlled by NADPH metabolism. Proceedings of the National Academy of Sciences 116, 12173–12182.

The latter, for example, can vary up to 400 ‰ for microbial lipids (Wijker et al., 2019), depending on the type of microbial metabolism (Zhang et al., 2009), lipid biosynthetic pathway (Fang et al., 2006, 2014) and environmental factors.
View in article
Zhang, X., Gillespie, A.L., Sessions, A.L. (2009) Large D/H variations in bacterial lipids reflect central metabolic pathways. Proceedings of the National Academy of Sciences 106, 12580–12586.

Despite the fact that both BrFAs and SCFAs are synthesised by the same biosynthetic pathway with almost the same enzymes (Fig. S-3) and that the mechanisms of lipid biosynthesis are strongly conserved across most bacteria phyla (Rock and Jackowski, 2002), the reducing power NADPH produced by different metabolic pathways retain different isotopic compositions, and consequently differing δD of fatty acids, as NADPH provides the largest source of hydrogen in microbial biosynthesis of fatty acids (Zhang et al., 2009; Wijker et al., 2019; see discussion below).
View in article
top
Supplementary Information
The Supplementary Information includes:
- Explanation for Graphical Abstract
- Materials and Methods
- Calculation of Isotope Enrichment, Fractionation Factors and the Kinetic Isotope Effect, εPDH
- Biosynthesis Pathways for Straight- and Branched-Chain Fatty Acids
- Calculation of Lambda Index
- Statistical Analysis for Correlation Coefficient
- Figures S-1 to S-3
- Supplementary Information References
Download the Supplementary Information (PDF)
Figures and Tables
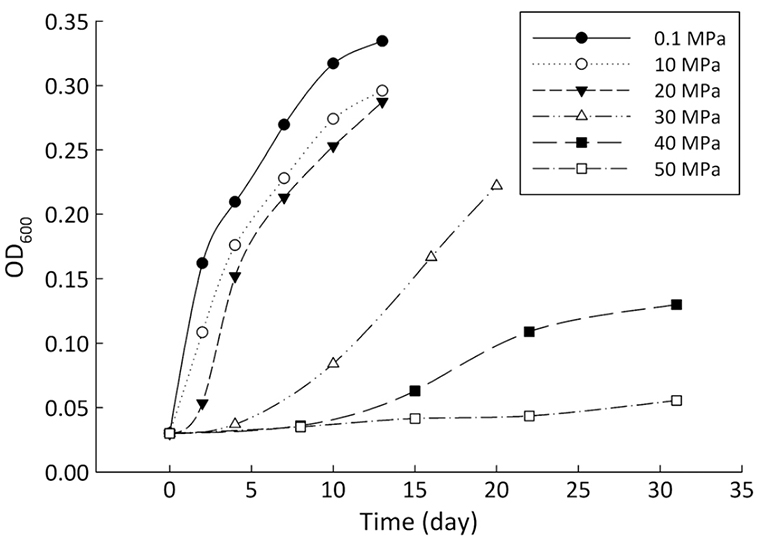
Figure 1 Growth curve of Sporosarcina sp. DSK25 under different pressures.
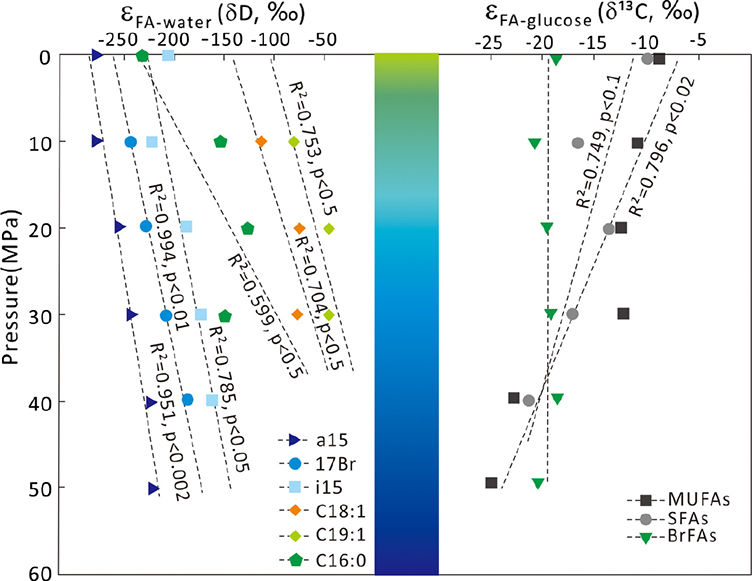
Figure 2 Carbon and hydrogen isotope fractionation of Sporosarcina sp. DSK25 changes with different growth pressures.
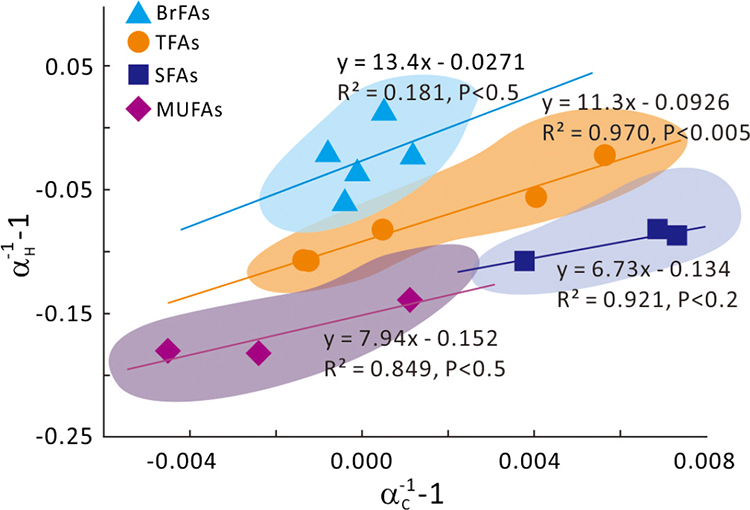
Figure 3 Plot of hydrogen versus carbon isotope fractionation factors observed in lipid biosynthesis by Sporosarcina sp. DSK25 grown at pressures of 0.1, 10, 20, 30, 40 and 50 MPa. The slope of the regression curves gives the lambda (Λ) values. TFAs: total fatty acids; MUFAs: monounsaturated fatty acids; SFAs: saturated fatty acids; BrFAs: branched-chain fatty acids; αH: fractionation factor of hydrogen isotope, αC: fractionation factor of carbon isotope.