Hydrothermal vents and organic ligands sustained the Precambrian copper budget
Affiliations | Corresponding Author | Cite as | Funding information- Share this article
-
Article views:460Cumulative count of HTML views and PDF downloads.
- Download Citation
- Rights & Permissions
top
Abstract
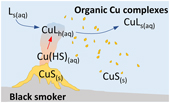
Figures
![]() Figure 1 Total Cu concentration in seawater for different model scenarios. (a) Modern ocean. (b) Fraction of hydrothermal Cu contribution to total marine Cu budget in the modern ocean. Scenarios 3 and 4 are deemed the most realistic according to previous work (Sander and Koschinsky, 2011). (c) Proterozoic ocean. (d) Effect of the seawater residence time on CuT for scenario 2 in the Proterozoic (panel c). (e) Archean ocean. (f) Effect of seawater temperature on CuT for scenario 2 in the Archean (panel e). | ![]() Figure 2 Dissolved copper speciation during mixing between hydrothermal fluid and seawater in the modern ocean under four different model scenarios (see Fig. 1). Note that seawater has an initial CuT concentration of zero; the seawater concentration shown in Figure 1 is calculated after combining the hydrothermal mixing model with the ocean box model (Fig. S-1). Solid lines = Cu2+ species, dashed lines = Cu+ species. The x axis shows the mixing ratio of seawater to hydrothermal fluid. Thick solid grey line = CuT. | ![]() Figure 3 Dissolved copper speciation in the Archean (a, b) and Proterozoic (c, d) during mixing between hydrothermal fluid and seawater, showing two different model scenarios for each time bin. As for Figure 2, seawater CuT is set to zero in these simulations and then calculated with the ocean box model in a separate step (Fig. S-1). Colour code and symbols as in Figure 2. In the absence of ligands, aqueous Cu(HS) is the dominant species in both the Archean and Proterozoic ocean (not plotted). Thick solid grey line = CuT. | ![]() Figure 4 Compilation of (a) the total marine Cu concentration and (b) the hydrothermal contribution to the marine Cu budget through time. For the Archean, the lower bound is scenario 2 in Figure 1e; the upper bound corresponds to scenario 4 in Figure 1e with a 10-fold longer residence time (Fig. S-7). For the Proterozoic, the lower bound is scenario 2 in Figure 1c with a 10-fold shorter residence time (Fig. 1d) while the upper bound corresponds to scenario 5 in Figure 1c with a modern residence time. |
Figure 1 | Figure 2 | Figure 3 | Figure 4 |
top
Introduction
Transition metals have long been recognised as essential for numerous cellular functions and metabolic pathways. The course of biological evolution from the Archean to the modern may therefore in part have been driven by the bioavailability of these elements in the early ocean. Earlier theoretical work (Saito et al., 2003
Saito, M.A., Sigman, D.M., Morel, F.M.M. (2003) The bioinorganic chemistry of the ancient ocean: the co-evolution of cyanobacterial metal requirements and biogeochemical cycles at the Archean-Proterozoic boundary? Inorganica Chimica Acta 356, 308–318.
) suggested extremely low concentrations of Cu and Zn in the Archean and Proterozoic ocean, which triggered several hypotheses about metal-limited biogeochemical cycles. For example, Zn scarcity has been invoked to explain the late rise of eukaryotes, which have a high Zn demand (Dupont et al., 2006Dupont, C.L., Yang, S., Palenik, B., Bourne, P.E. (2006) Modern proteomes contain putative imprints of ancient shifts in trace metal geochemistry. Proceedings of the National Academy of Sciences 103, 17822–17827.
), while Cu limitation has been proposed as a mechanism to build up high levels of N2O in the Proterozoic atmosphere (Buick, 2007Buick, R. (2007) Did the Proterozoic ‘Canfield Ocean’ cause a laughing gas greenhouse? Geobiology 5, 97–100.
). However, recent studies of marine sedimentary rocks do not support Zn- and Cu-depleted oceans in the Precambrian (Scott et al., 2012Scott, C., Planavsky, N.J., Dupont, C.L., Kendall, B., Gill, B.C., Robbins, L.J., Husband, K.F., Arnold, G.L., Wing, B., Poulton, S.W., Bekker, A., Anbar, A., Konhauser, K., Lyons, T.W. (2012) Bioavailability of zinc in marine systems through time. Nature Geoscience 6, 125–128.
; Large et al., 2014Large, R.R., Halpin, J.A., Danyushevsky, L.V., Maslennikov, V.V., Bull, S.W., Long, J.A., Gregory, D.D., Lounejeva, E., Lyons, T.W., Sack, P.J., McGoldrick, J.J., Calver, C.R. (2014) Trace element content of sedimentary pyrite as a new proxy for deep-time ocean-atmosphere evolution. Earth and Planetary Science Letters 389, 209–220.
; Chi Fru et al., 2016Chi Fru, E., Rodríguez, N.P., Partin, C.A., Lalonde, S.V., Andersson, P., Weiss, D.J., El Albani, A., Rodushkin, I., Konhauser, K.O. (2016) Cu isotopes in marine black shales record the Great Oxidation Event. Proceedings of the National Academy of Sciences 113, 4941–4946.
; Robbins et al., 2016Robbins, L.J., Lalonde, S.V., Planavsky, N.J., Partin, C.A., Reinhard, C.T., Kendall, B., Scott, C., Hardisty, D.S., Gill, B.C., Alessi, D.S., Dupont, C.L. (2016) Trace elements at the intersection of marine biological and geochemical evolution. Earth-Science Reviews 163, 323–348.
). To explain this discrepancy, some workers have suggested a stronger hydrothermal metal influx into the ocean (Scott et al., 2012Scott, C., Planavsky, N.J., Dupont, C.L., Kendall, B., Gill, B.C., Robbins, L.J., Husband, K.F., Arnold, G.L., Wing, B., Poulton, S.W., Bekker, A., Anbar, A., Konhauser, K., Lyons, T.W. (2012) Bioavailability of zinc in marine systems through time. Nature Geoscience 6, 125–128.
; Robbins et al., 2013Robbins, L.J., Lalonde, S.V., Saito, M.A., Planavsky, N.J., Miloszewska, A.M., Pecoits, E., Scott, C., Dupont, C.L., Kappler, A., Konhauser, K. (2013) Authigenic iron oxide proxies for marine zinc over geological time and implications for eukaryotic metallome evolution. Geobiology 11, 295–306.
). This hypothesis would be consistent with independent evidence that hydrothermal activity was elevated in the early Precambrian compared to today (Viehmann et al., 2015Viehmann, S., Bau, M., Hoffmann, J.E., Münker, C. (2015) Geochemistry of the Krivoy Rog Banded Iron Formation, Ukraine, and the impact of peak episodes of increased global magmatic activity on the trace element composition of Precambrian seawater. Precambrian Research 270, 165–180.
). Indeed, modern hydrothermal vents can disperse metals widely into the open ocean and enhance biological productivity in surface waters (e.g., Fitzsimmons et al., 2014Fitzsimmons, J.N., Boyle, E.A., Jenkins, W.J. (2014) Distal transport of dissolved hydrothermal iron in the deep South Pacific Ocean. Proceedings of the National Academy of Sciences 111, 16654–16661.
). However, whether this hydrothermal metal flux could have persisted in the Precambrian remains elusive.To address this knowledge gap, this study explores the role of organic ligands in stabilising hydrothermally sourced Cu in Precambrian seawater. Copper is chosen as a focus, because thermodynamic data are available to model Cu speciation in hydrothermal fluids. Using those data, Sander and Koschinsky (2011)
Sander, S.G., Koschinsky, A. (2011) Metal flux from hydrothermal vents increased by organic complexation. Nature Geoscience 4, 145–150.
showed that modern hydrothermal vents contribute 5–14 % of the ocean’s Cu budget in the form of organic complexes. Here the thermodynamic model is adapted to Precambrian seawater conditions, and subsequently paired with a box model that combines the hydrothermal Cu flux with the riverine flux and the residence of Cu in seawater to derive a global average Cu concentration through time (see Supplementary Information for methods). In brief, the thermodynamic model simulates mixing between a Cu-rich hydrothermal fluid at 300 °C and Cu-depleted seawater at 4 °C up to a ratio of 1∶1000 and allows Cu minerals to precipitate. Organic ligands are included in the hydrothermal fluid (Lh+) and in seawater (Ls+) with empirically-determined stability constants of 10−14 for Cu ligand complexes in both fluids (Sander and Koschinsky, 2011Sander, S.G., Koschinsky, A. (2011) Metal flux from hydrothermal vents increased by organic complexation. Nature Geoscience 4, 145–150.
). These constants are assumed to be applicable for the Precambrian models as well. The Cu concentration remaining in solution after precipitation has ceased is then entered into the box model as an input parameter for the hydrothermal Cu source to the ocean. The riverine source is taken from the literature. Both Archean and Proterozoic seawater are modelled as ferruginous (anoxic with 100 μM of free Fe2+) (Holland, 1984Holland, H.D. (1984) The chemical evolution of the atmosphere and oceans. Princeton University Press, Princeton, NJ.
), but the Archean is defined by having very low concentrations of
Claire, M.W., Catling, D.C., Zahnle, K.J. (2006) Biogeochemical modelling of the rise in atmospheric oxygen. Geobiology 4, 239–269.
) while the Proterozoic is modelled with 3 mM of
top
Results
For the modern ocean, the model produced marine Cu concentrations of 2.7–3.4 nM (Fig. 1a), in good agreement with observations of 1–3 nM in the Pacific Ocean (Whitby et al., 2018
Whitby, H., Posacka, A.M., Maldonado, M.T., van den Berg, C.M. (2018) Copper-binding ligands in the NE Pacific. Marine Chemistry 204, 36–48.
). Hydrothermal fluids contributed 2–20 % of the total Cu (for Lh of 1–10 μM), similar to the 5–14 % found by Sander and Koschinsky (2011)Sander, S.G., Koschinsky, A. (2011) Metal flux from hydrothermal vents increased by organic complexation. Nature Geoscience 4, 145–150.
. At the 1000∶1 mixing ratio, the concentration of ligand-bound Cu (CuLh+ and CuLs+) was of the order of a few nM, while that of free Cu2+ was 10−13 to 10−15 M (Fig. 2), which agrees with measurements from modern seawater (Vraspir and Butler, 2009Vraspir, J.M., Butler, A. (2009) Chemistry of marine ligands and siderophores. Annual Review of Marine Science 1, 43–63.
). The concentration of Cu bound to the seawater ligand (CuLs+) increased from 0.2 nM to 7 nM at the 1000∶1 mixing ratio as the concentration of the hydrothermal ligand Lh+ was raised from 0 to 10 uM (Fig. 2), although Ls+ in seawater stayed constant at 10 nM. This result implies that the hydrothermal ligand prevents some hydrothermally-sourced Cu from precipitating and later passes that Cu on to the seawater ligand as the latter becomes more abundant and thus thermodynamically favourable. Hydrothermal ligands are thus important for transporting Cu into the ocean. Major precipitates in the modern ocean scenario included chalcocite and ferrite-Cu (Fig. S-2).
Figure 1 Total Cu concentration in seawater for different model scenarios. (a) Modern ocean. (b) Fraction of hydrothermal Cu contribution to total marine Cu budget in the modern ocean. Scenarios 3 and 4 are deemed the most realistic according to previous work (Sander and Koschinsky, 2011
Sander, S.G., Koschinsky, A. (2011) Metal flux from hydrothermal vents increased by organic complexation. Nature Geoscience 4, 145–150.
). (c) Proterozoic ocean. (d) Effect of the seawater residence time on CuT for scenario 2 in the Proterozoic (panel c). (e) Archean ocean. (f) Effect of seawater temperature on CuT for scenario 2 in the Archean (panel e).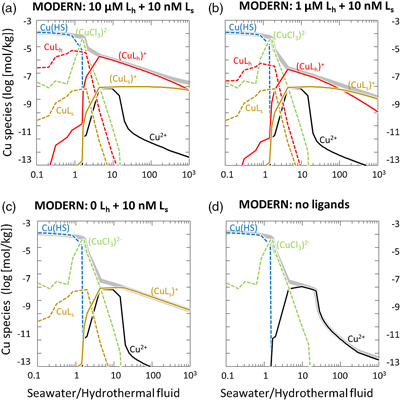
Figure 2 Dissolved copper speciation during mixing between hydrothermal fluid and seawater in the modern ocean under four different model scenarios (see Fig. 1). Note that seawater has an initial CuT concentration of zero; the seawater concentration shown in Figure 1 is calculated after combining the hydrothermal mixing model with the ocean box model (Fig. S-1). Solid lines = Cu2+ species, dashed lines = Cu+ species. The x axis shows the mixing ratio of seawater to hydrothermal fluid. Thick solid grey line = CuT.
In the Archean ocean scenario, even the highest amounts of modern observed ligand concentrations had very little effect on raising total marine Cu concentrations at high mixing ratios with seawater (CuT; Fig. 1e). Most Cu was lost by the precipitation of Cu sulfides proximal to the vent. The modelled CuT concentration was thus always several orders of magnitude below 1 nM. Values closer to the modern range could be obtained if the temperature of seawater was raised to around 50 °C (Fig. 1f), or if the concentration of Ls+ was increased drastically to 1000 μM and the residence time of CuT in the ocean was extended to >100,000 yr (Fig. S-7). Increasing Lh+ did not have nearly the same effect as increasing Ls+ (not shown). In any case, the riverine Cu contribution was less than a few percent (Fig. 1e).
In the Proterozoic, modelled CuT levels with the maximum modern ligand load (10 μM Lh+ and 10 nM Ls+) were still lower than in the modern, but several orders of magnitude above the Archean. The riverine contribution far outweighed the hydrothermal input (Fig. 1c). Similar to the Archean, high concentrations of Ls+ were needed to establish high marine CuT levels similar to the modern. Importantly, lowering the residence time of CuT in seawater, as predicted by the expansion of euxinic waters along Proterozoic ocean margins (Lyons et al., 2014
Lyons, T.W., Reinhard, C.T., Planavsky, N.J. (2014) The rise of oxygen in Earth’s early ocean and atmosphere. Nature 506, 307–315.
), did not push CuT levels far out of the modern range (Fig. 1d). Sensitivity tests of differing Fe or
top
Discussion
In the Archean, only high seawater temperatures (Fig. 1f) or unusually high concentrations of organic ligands in seawater paired with a much longer seawater residence time of CuT may have been able to push CuT concentrations closer to modern values. It is conceivable that the amount of suspended organic matter in seawater was indeed higher in the Precambrian, prior to the rise of filter feeding metazoans and complex algae (Lenton et al., 2014
Lenton, T.M., Boyle, R.A., Poulton, S.W., Shields-Zhou, G.A., Butterfield, N.J. (2014) Co-evolution of eukaryotes and ocean oxygenation in the Neoproterozoic era. Nature Geoscience 7, 257–265.
). For example, a microbially-dominated anoxic lake in Antarctica, which lacks significant input of eukaryotic biomass, reaches DOC levels up to 2000 μM (McKnight et al., 1991McKnight, D.M., Aiken, G.R., Smith, R.L. (1991) Aquatic fulvic acids in microbially based ecosystems: results from two desert lakes in Antarctica. Limnology and Oceanography 36, 998–1006.
). If a high proportion of the organic matter was Cu-binding, it may thus have increased the residence time and concentration of CuT in seawater, which may explain the nearly constant Cu levels in sedimentary rocks through time (Large et al., 2014Large, R.R., Halpin, J.A., Danyushevsky, L.V., Maslennikov, V.V., Bull, S.W., Long, J.A., Gregory, D.D., Lounejeva, E., Lyons, T.W., Sack, P.J., McGoldrick, J.J., Calver, C.R. (2014) Trace element content of sedimentary pyrite as a new proxy for deep-time ocean-atmosphere evolution. Earth and Planetary Science Letters 389, 209–220.
; Chi Fru et al., 2016Chi Fru, E., Rodríguez, N.P., Partin, C.A., Lalonde, S.V., Andersson, P., Weiss, D.J., El Albani, A., Rodushkin, I., Konhauser, K.O. (2016) Cu isotopes in marine black shales record the Great Oxidation Event. Proceedings of the National Academy of Sciences 113, 4941–4946.
). Alternatively, the unexpectedly high Archean Cu enrichments in rocks may reflect higher seawater temperatures (Tartèse et al., 2017Tartèse, R., Chaussidon, M., Gurenko, A., Delarue, F., Robert, F. (2017) Warm Archean oceans reconstructed from oxygen isotope composition of early-life remnants. Geochemical Perspectives Letters 3, 55–65.
), which would have raised the solubility of Cu sulfide minerals.Throughout the Archean, hydrothermal vents were the major source of Cu to the ocean, because the riverine flux was low in the absence of oxidative weathering (Hao et al., 2017
Hao, J., Sverjensky, D.A., Hazen, R.M. (2017) Mobility of nutrients and trace metals during weathering in the late Archean. Earth and Planetary Science Letters 471, 148–159.
). Interestingly, previous workers have pointed out that several prebiotic reactions may have occurred in hydrothermal settings because of the abundance of catalytic metals (Martin et al., 2008Martin, W., Baross, J., Kelley, D., Russell, M.J. (2008) Hydrothermal vents and the origin of life. Nature Reviews Microbiology 6, 805–814.
). The results from this study suggest that CuT concentrations were closest to modern values proximal to hydrothermal vents where the mixing ratio of seawater to vent fluid was lower (Fig. 3). Hence the model supports the view that the origin of metalloenzymes occurred proximal to hydrothermal environments. At the same time, the dispersal of ligand-bound Cu into the global ocean from hydrothermal point sources may be consistent with the idea that prebiotic hydrothermal products may have undergone further modification and evolution in other environmental niches (Stüeken et al., 2013Stüeken, E.E., Anderson, R.E., Bowman, J.S., Brazelton, W.J., Colangelo-Lillis, J., Goldman, A.D., Som, S.M., Baross, J.A. (2013) Did life originate from a global chemical reactor? Geobiology 11, 101–126.
).
Figure 3 Dissolved copper speciation in the Archean (a, b) and Proterozoic (c, d) during mixing between hydrothermal fluid and seawater, showing two different model scenarios for each time bin. As for Figure 2, seawater CuT is set to zero in these simulations and then calculated with the ocean box model in a separate step (Fig. S-1). Colour code and symbols as in Figure 2. In the absence of ligands, aqueous Cu(HS) is the dominant species in both the Archean and Proterozoic ocean (not plotted). Thick solid grey line = CuT.
With the onset of oxidative sulfide weathering in the Proterozoic (the most probable Cu source to rivers), CuT levels were probably within 1–2 orders of magnitude of modern concentrations (Fig. 1d). Throughout the Proterozoic (Fig. 3c,d) and the modern (Fig. 2), and possibly earlier (Fig. 3b), CuT was largely organic-bound, which would explain the difference between these new results and earlier predictions (Saito et al., 2003
Saito, M.A., Sigman, D.M., Morel, F.M.M. (2003) The bioinorganic chemistry of the ancient ocean: the co-evolution of cyanobacterial metal requirements and biogeochemical cycles at the Archean-Proterozoic boundary? Inorganica Chimica Acta 356, 308–318.
). Unlike in the Archean, rivers largely controlled the marine CuT budget of the Proterozoic, but it is important to note that riverine Cu would also have needed to be ligand-bound to be stable in the Proterozoic ocean, as suggested by the seawater end member composition of the thermodynamic model (Fig. 3) and consistent with observations from modern rivers where Cu is overwhelmingly organic-bound (Hoffmann et al., 2007Hoffmann, S.R., Shafer, M.M., Armstrong, D.E. (2007) Strong colloidal and dissolved organic ligands binding copper and zinc in rivers. Environmental Science & Technology 41, 6996–7002.
). Ligand-bound Cu can be bioavailable (Zhao et al., 2016Zhao, C.-M., Campbell, P.G., Wilkinson, K.J. (2016) When are metal complexes bioavailable? Environmental Chemistry 13, 425–433.
), making it unlikely that Cu was bio-limiting in the Proterozoic and that Cu limitation caused large scale production of N2O gas (Buick, 2007Buick, R. (2007) Did the Proterozoic ‘Canfield Ocean’ cause a laughing gas greenhouse? Geobiology 5, 97–100.
). One the other hand, Cu toxicity to cyanobacteria may have arisen earlier than previously thought. It has been shown that free Cu2+ concentrations around 10−11 M can negatively impact both CO2 and N2 fixation (Rueter and Petersen, 1987Rueter, J.G., Petersen, R.R. (1987) Micronutrient effects on cyanobacterial growth and physiology. New Zealand Journal of Marine and Freshwater Research 21, 435–445.
). This Cu sensitivity may be an artifact of a relatively Cu-free setting during the origin of cyanobacteria. If so, then the model of this study would support the idea that cyanobacteria originated in the Archean (Lyons et al., 2014Lyons, T.W., Reinhard, C.T., Planavsky, N.J. (2014) The rise of oxygen in Earth’s early ocean and atmosphere. Nature 506, 307–315.
), prior to the expansion of the marine CuT budget.top
Conclusion
Our understanding of Precambrian ocean chemistry has evolved since the original study by Saito et al. (2003)
Saito, M.A., Sigman, D.M., Morel, F.M.M. (2003) The bioinorganic chemistry of the ancient ocean: the co-evolution of cyanobacterial metal requirements and biogeochemical cycles at the Archean-Proterozoic boundary? Inorganica Chimica Acta 356, 308–318.
that predicted severe Cu limitation in the Archean and Proterozoic due to rapid scavenging of Cu by free H2S. It is now evident that such H2S-rich conditions were spatially limited in the early oceans (Lyons et al., 2014Lyons, T.W., Reinhard, C.T., Planavsky, N.J. (2014) The rise of oxygen in Earth’s early ocean and atmosphere. Nature 506, 307–315.
), warranting a new model of CuT solubility. The results presented here support the inference that Archean CuT levels were probably lower than today (Fig. 4a), unless seawater was warmer or the abundance of organic ligands was markedly higher. Near modern levels of CuT were likely reached in the Proterozoic with the onset of oxidative weathering, despite a potentially shorter residence time in the ocean. In the Archean, hydrothermal vents constituted the major source of Cu (Fig. 4b), which provides additional credence to the idea that key prebiotic processes involving the origin of metalloenzymes occurred in hydrothermal settings. From the Proterozoic onwards, and perhaps earlier, the vast majority of CuT in seawater has been stabilised by organic ligands, which thus probably lifted Cu limitations for the evolving biosphere. While the model presented in this study focused on Cu only, it is predicted that similar mechanisms would apply for Zn and perhaps other base metals.
Figure 4 Compilation of (a) the total marine Cu concentration and (b) the hydrothermal contribution to the marine Cu budget through time. For the Archean, the lower bound is scenario 2 in Figure 1e; the upper bound corresponds to scenario 4 in Figure 1e with a 10-fold longer residence time (Fig. S-7). For the Proterozoic, the lower bound is scenario 2 in Figure 1c with a 10-fold shorter residence time (Fig. 1d) while the upper bound corresponds to scenario 5 in Figure 1c with a modern residence time.
top
Acknowledgements
I thank Rob Raiswell, Kurt Konhauser and one anonymous reviewer for helpful comments that improved the manuscript. Liane Benning is thanked for editorial handling.
Editor: Liane G. Benning
top
References
Buick, R. (2007) Did the Proterozoic ‘Canfield Ocean’ cause a laughing gas greenhouse? Geobiology 5, 97–100.

For example, Zn scarcity has been invoked to explain the late rise of eukaryotes, which have a high Zn demand (Dupont et al., 2006), while Cu limitation has been proposed as a mechanism to build up high levels of N2O in the Proterozoic atmosphere (Buick, 2007).
View in article
Ligand-bound Cu can be bioavailable (Zhao et al., 2016), making it unlikely that Cu was bio-limiting in the Proterozoic and that Cu limitation caused large scale production of N2O gas (Buick, 2007).
View in article
Chi Fru, E., Rodríguez, N.P., Partin, C.A., Lalonde, S.V., Andersson, P., Weiss, D.J., El Albani, A., Rodushkin, I., Konhauser, K.O. (2016) Cu isotopes in marine black shales record the Great Oxidation Event. Proceedings of the National Academy of Sciences 113, 4941–4946.

However, recent studies of marine sedimentary rocks do not support Zn- and Cu-depleted oceans in the Precambrian (Scott et al., 2012; Large et al., 2014; Chi Fru et al., 2016; Robbins et al., 2016).
View in article
If a high proportion of the organic matter was Cu-binding, it may thus have increased the residence time and concentration of CuT in seawater, which may explain the nearly constant Cu levels in sedimentary rocks through time (Large et al., 2014; Chi Fru et al., 2016).
View in article
Claire, M.W., Catling, D.C., Zahnle, K.J. (2006) Biogeochemical modelling of the rise in atmospheric oxygen. Geobiology 4, 239–269.

Both Archean and Proterozoic seawater are modelled as ferruginous (anoxic with 100 μM of free Fe2+) (Holland, 1984), but the Archean is defined by having very low concentrations of (20 μM) and minor amounts of dissolved H2 (Claire et al., 2006) while the Proterozoic is modelled with 3 mM of
in the absence of H2 gas.
View in article
Dupont, C.L., Yang, S., Palenik, B., Bourne, P.E. (2006) Modern proteomes contain putative imprints of ancient shifts in trace metal geochemistry. Proceedings of the National Academy of Sciences 103, 17822–17827.

For example, Zn scarcity has been invoked to explain the late rise of eukaryotes, which have a high Zn demand (Dupont et al., 2006), while Cu limitation has been proposed as a mechanism to build up high levels of N2O in the Proterozoic atmosphere (Buick, 2007).
View in article
Fitzsimmons, J.N., Boyle, E.A., Jenkins, W.J. (2014) Distal transport of dissolved hydrothermal iron in the deep South Pacific Ocean. Proceedings of the National Academy of Sciences 111, 16654–16661.

Indeed, modern hydrothermal vents can disperse metals widely into the open ocean and enhance biological productivity in surface waters (e.g., Fitzsimmons et al., 2014).
View in article
Hao, J., Sverjensky, D.A., Hazen, R.M. (2017) Mobility of nutrients and trace metals during weathering in the late Archean. Earth and Planetary Science Letters 471, 148–159.

Throughout the Archean, hydrothermal vents were the major source of Cu to the ocean, because the riverine flux was low in the absence of oxidative weathering (Hao et al., 2017).
View in article
Hoffmann, S.R., Shafer, M.M., Armstrong, D.E. (2007) Strong colloidal and dissolved organic ligands binding copper and zinc in rivers. Environmental Science & Technology 41, 6996–7002.

Unlike in the Archean, rivers largely controlled the marine CuT budget of the Proterozoic, but it is important to note that riverine Cu would also have needed to be ligand-bound to be stable in the Proterozoic ocean, as suggested by the seawater end member composition of the thermodynamic model (Fig. 3) and consistent with observations from modern rivers where Cu is overwhelmingly organic-bound (Hoffmann et al., 2007).
View in article
Holland, H.D. (1984) The chemical evolution of the atmosphere and oceans. Princeton University Press, Princeton, NJ.

Both Archean and Proterozoic seawater are modelled as ferruginous (anoxic with 100 μM of free Fe2+) (Holland, 1984), but the Archean is defined by having very low concentrations of (20 μM) and minor amounts of dissolved H2 (Claire et al., 2006) while the Proterozoic is modelled with 3 mM of
in the absence of H2 gas.
View in article
Large, R.R., Halpin, J.A., Danyushevsky, L.V., Maslennikov, V.V., Bull, S.W., Long, J.A., Gregory, D.D., Lounejeva, E., Lyons, T.W., Sack, P.J., McGoldrick, J.J., Calver, C.R. (2014) Trace element content of sedimentary pyrite as a new proxy for deep-time ocean-atmosphere evolution. Earth and Planetary Science Letters 389, 209–220.

However, recent studies of marine sedimentary rocks do not support Zn- and Cu-depleted oceans in the Precambrian (Scott et al., 2012; Large et al., 2014; Chi Fru et al., 2016; Robbins et al., 2016).
View in article
If a high proportion of the organic matter was Cu-binding, it may thus have increased the residence time and concentration of CuT in seawater, which may explain the nearly constant Cu levels in sedimentary rocks through time (Large et al., 2014; Chi Fru et al., 2016).
View in article
Lenton, T.M., Boyle, R.A., Poulton, S.W., Shields-Zhou, G.A., Butterfield, N.J. (2014) Co-evolution of eukaryotes and ocean oxygenation in the Neoproterozoic era. Nature Geoscience 7, 257–265.

It is conceivable that the amount of suspended organic matter in seawater was indeed higher in the Precambrian, prior to the rise of filter feeding metazoans and complex algae (Lenton et al., 2014).
View in article
Lyons, T.W., Reinhard, C.T., Planavsky, N.J. (2014) The rise of oxygen in Earth’s early ocean and atmosphere. Nature 506, 307–315.

Importantly, lowering the residence time of CuT in seawater, as predicted by the expansion of euxinic waters along Proterozoic ocean margins (Lyons et al., 2014), did not push CuT levels far out of the modern range (Fig. 1d).
View in article
If so, then the model of this study would support the idea that cyanobacteria originated in the Archean (Lyons et al., 2014), prior to the expansion of the marine CuT budget.
View in article
It is now evident that such H2S-rich conditions were spatially limited in the early oceans (Lyons et al., 2014), warranting a new model of CuT solubility.
View in article
Martin, W., Baross, J., Kelley, D., Russell, M.J. (2008) Hydrothermal vents and the origin of life. Nature Reviews Microbiology 6, 805–814.

Interestingly, previous workers have pointed out that several prebiotic reactions may have occurred in hydrothermal settings because of the abundance of catalytic metals (Martin et al., 2008).
View in article
McKnight, D.M., Aiken, G.R., Smith, R.L. (1991) Aquatic fulvic acids in microbially based ecosystems: results from two desert lakes in Antarctica. Limnology and Oceanography 36, 998–1006.

For example, a microbially-dominated anoxic lake in Antarctica, which lacks significant input of eukaryotic biomass, reaches DOC levels up to 2000 μM (McKnight et al., 1991).
View in article
Robbins, L.J., Lalonde, S.V., Saito, M.A., Planavsky, N.J., Miloszewska, A.M., Pecoits, E., Scott, C., Dupont, C.L., Kappler, A., Konhauser, K. (2013) Authigenic iron oxide proxies for marine zinc over geological time and implications for eukaryotic metallome evolution. Geobiology 11, 295–306.

To explain this discrepancy, some workers have suggested a stronger hydrothermal metal influx into the ocean (Scott et al., 2012; Robbins et al., 2013).
View in article
Robbins, L.J., Lalonde, S.V., Planavsky, N.J., Partin, C.A., Reinhard, C.T., Kendall, B., Scott, C., Hardisty, D.S., Gill, B.C., Alessi, D.S., Dupont, C.L. (2016) Trace elements at the intersection of marine biological and geochemical evolution. Earth-Science Reviews 163, 323–348.

However, recent studies of marine sedimentary rocks do not support Zn- and Cu-depleted oceans in the Precambrian (Scott et al., 2012; Large et al., 2014; Chi Fru et al., 2016; Robbins et al., 2016).
View in article
Rueter, J.G., Petersen, R.R. (1987) Micronutrient effects on cyanobacterial growth and physiology. New Zealand Journal of Marine and Freshwater Research 21, 435–445.

It has been shown that free Cu2+ concentrations around 10−11 M can negatively impact both CO2 and N2 fixation (Rueter and Petersen, 1987).
View in article
Saito, M.A., Sigman, D.M., Morel, F.M.M. (2003) The bioinorganic chemistry of the ancient ocean: the co-evolution of cyanobacterial metal requirements and biogeochemical cycles at the Archean-Proterozoic boundary? Inorganica Chimica Acta 356, 308–318.

Earlier theoretical work (Saito et al., 2003) suggested extremely low concentrations of Cu and Zn in the Archean and Proterozoic ocean, which triggered several hypotheses about metal-limited biogeochemical cycles.
View in article
Throughout the Proterozoic (Fig. 3c,d) and the modern (Fig. 2), and possibly earlier (Fig. 3b), CuT was largely organic-bound, which would explain the difference between these new results and earlier predictions (Saito et al., 2003).
View in article
Our understanding of Precambrian ocean chemistry has evolved since the original study by Saito et al. (2003) that predicted severe Cu limitation in the Archean and Proterozoic due to rapid scavenging of Cu by free H2S.
View in article
Sander, S.G., Koschinsky, A. (2011) Metal flux from hydrothermal vents increased by organic complexation. Nature Geoscience 4, 145–150.

Copper is chosen as a focus, because thermodynamic data are available to model Cu speciation in hydrothermal fluids. Using those data, Sander and Koschinsky (2011) showed that modern hydrothermal vents contribute 5–14 % of the ocean’s Cu budget in the form of organic complexes.
View in article
Organic ligands are included in the hydrothermal fluid (Lh+) and in seawater (Ls+) with empirically-determined stability constants of 10−14 for Cu ligand complexes in both fluids (Sander and Koschinsky, 2011).
View in article
Hydrothermal fluids contributed 2–20 % of the total Cu (for Lh of 1–10 μM), similar to the 5–14 % found by Sander and Koschinsky (2011).
View in article
Scenarios 3 and 4 are deemed the most realistic according to previous work (Sander and Koschinsky, 2011). (c) Proterozoic ocean.
View in article
Scott, C., Planavsky, N.J., Dupont, C.L., Kendall, B., Gill, B.C., Robbins, L.J., Husband, K.F., Arnold, G.L., Wing, B., Poulton, S.W., Bekker, A., Anbar, A., Konhauser, K., Lyons, T.W. (2012) Bioavailability of zinc in marine systems through time. Nature Geoscience 6, 125–128.

However, recent studies of marine sedimentary rocks do not support Zn- and Cu-depleted oceans in the Precambrian (Scott et al., 2012; Large et al., 2014; Chi Fru et al., 2016; Robbins et al., 2016).
View in article
To explain this discrepancy, some workers have suggested a stronger hydrothermal metal influx into the ocean (Scott et al., 2012; Robbins et al., 2013).
View in article
Stüeken, E.E., Anderson, R.E., Bowman, J.S., Brazelton, W.J., Colangelo-Lillis, J., Goldman, A.D., Som, S.M., Baross, J.A. (2013) Did life originate from a global chemical reactor? Geobiology 11, 101–126.

At the same time, the dispersal of ligand-bound Cu into the global ocean from hydrothermal point sources may be consistent with the idea that prebiotic hydrothermal products may have undergone further modification and evolution in other environmental niches (Stüeken et al., 2013).
View in article
Tartèse, R., Chaussidon, M., Gurenko, A., Delarue, F., Robert, F. (2017) Warm Archean oceans reconstructed from oxygen isotope composition of early-life remnants. Geochemical Perspectives Letters 3, 55–65.

Alternatively, the unexpectedly high Archean Cu enrichments in rocks may reflect higher seawater temperatures (Tartèse et al., 2017), which would have raised the solubility of Cu sulfide minerals.
View in article
Viehmann, S., Bau, M., Hoffmann, J.E., Münker, C. (2015) Geochemistry of the Krivoy Rog Banded Iron Formation, Ukraine, and the impact of peak episodes of increased global magmatic activity on the trace element composition of Precambrian seawater. Precambrian Research 270, 165–180.

This hypothesis would be consistent with independent evidence that hydrothermal activity was elevated in the early Precambrian compared to today (Viehmann et al., 2015).
View in article
Vraspir, J.M., Butler, A. (2009) Chemistry of marine ligands and siderophores. Annual Review of Marine Science 1, 43–63.

At the 1000∶1 mixing ratio, the concentration of ligand-bound Cu (CuLh+ and CuLs+) was of the order of a few nM, while that of free Cu2+ was 10−13 to 10−15 M (Fig. 2), which agrees with measurements from modern seawater (Vraspir and Butler, 2009).
View in article
Whitby, H., Posacka, A.M., Maldonado, M.T., van den Berg, C.M. (2018) Copper-binding ligands in the NE Pacific. Marine Chemistry 204, 36–48.

For the modern ocean, the model produced marine Cu concentrations of 2.7–3.4 nM (Fig. 1a), in good agreement with observations of 1–3 nM in the Pacific Ocean (Whitby et al., 2018).
View in article
Zhao, C.-M., Campbell, P.G., Wilkinson, K.J. (2016) When are metal complexes bioavailable? Environmental Chemistry 13, 425–433.

Ligand-bound Cu can be bioavailable (Zhao et al., 2016), making it unlikely that Cu was bio-limiting in the Proterozoic and that Cu limitation caused large scale production of N2O gas (Buick, 2007).
View in article
top
Supplementary Information
The Supplementary Information includes:
- Thermodynamic Model
- Box Model
- Figures S-1 to S-7
- Tables S-1 to S-3
- Supplementary Information References
Download the Supplementary Information (PDF).
Figures

Figure 1 Total Cu concentration in seawater for different model scenarios. (a) Modern ocean. (b) Fraction of hydrothermal Cu contribution to total marine Cu budget in the modern ocean. Scenarios 3 and 4 are deemed the most realistic according to previous work (Sander and Koschinsky, 2011
Sander, S.G., Koschinsky, A. (2011) Metal flux from hydrothermal vents increased by organic complexation. Nature Geoscience 4, 145–150.
). (c) Proterozoic ocean. (d) Effect of the seawater residence time on CuT for scenario 2 in the Proterozoic (panel c). (e) Archean ocean. (f) Effect of seawater temperature on CuT for scenario 2 in the Archean (panel e).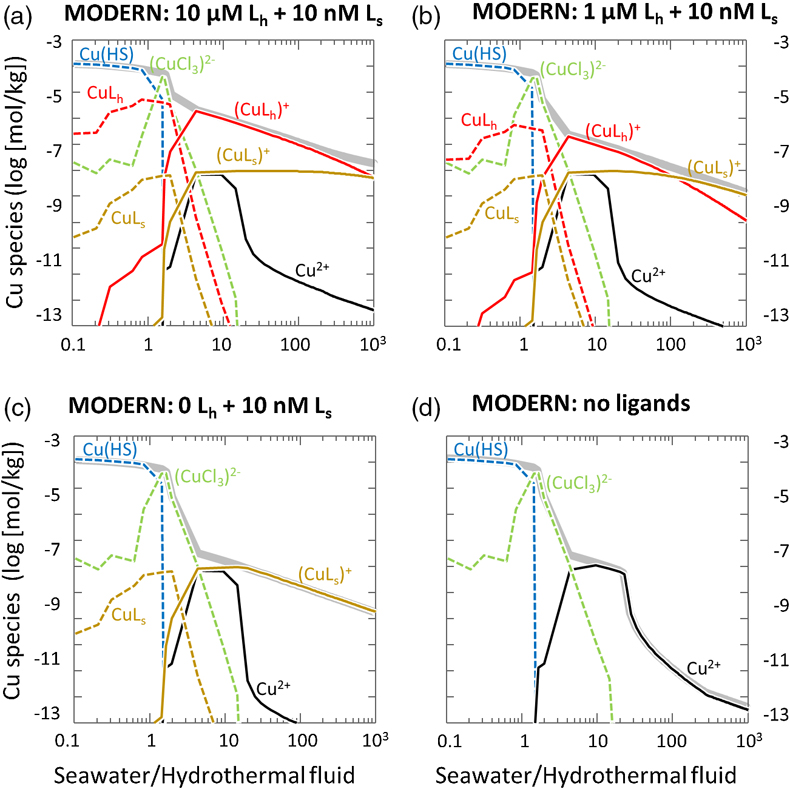
Figure 2 Dissolved copper speciation during mixing between hydrothermal fluid and seawater in the modern ocean under four different model scenarios (see Fig. 1). Note that seawater has an initial CuT concentration of zero; the seawater concentration shown in Figure 1 is calculated after combining the hydrothermal mixing model with the ocean box model (Fig. S-1). Solid lines = Cu2+ species, dashed lines = Cu+ species. The x axis shows the mixing ratio of seawater to hydrothermal fluid. Thick solid grey line = CuT.
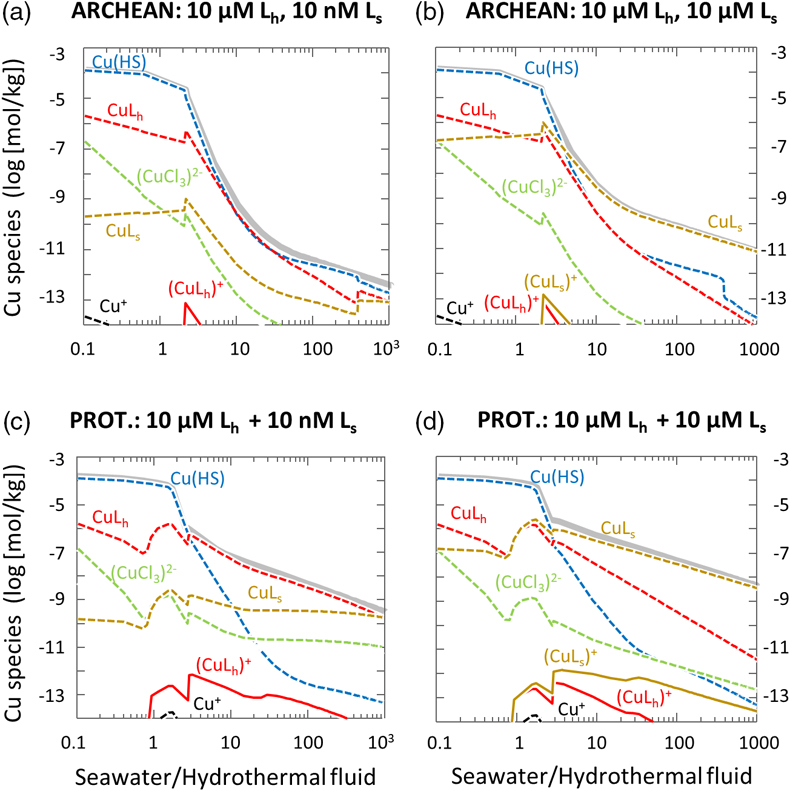
Figure 3 Dissolved copper speciation in the Archean (a, b) and Proterozoic (c, d) during mixing between hydrothermal fluid and seawater, showing two different model scenarios for each time bin. As for Figure 2, seawater CuT is set to zero in these simulations and then calculated with the ocean box model in a separate step (Fig. S-1). Colour code and symbols as in Figure 2. In the absence of ligands, aqueous Cu(HS) is the dominant species in both the Archean and Proterozoic ocean (not plotted). Thick solid grey line = CuT.
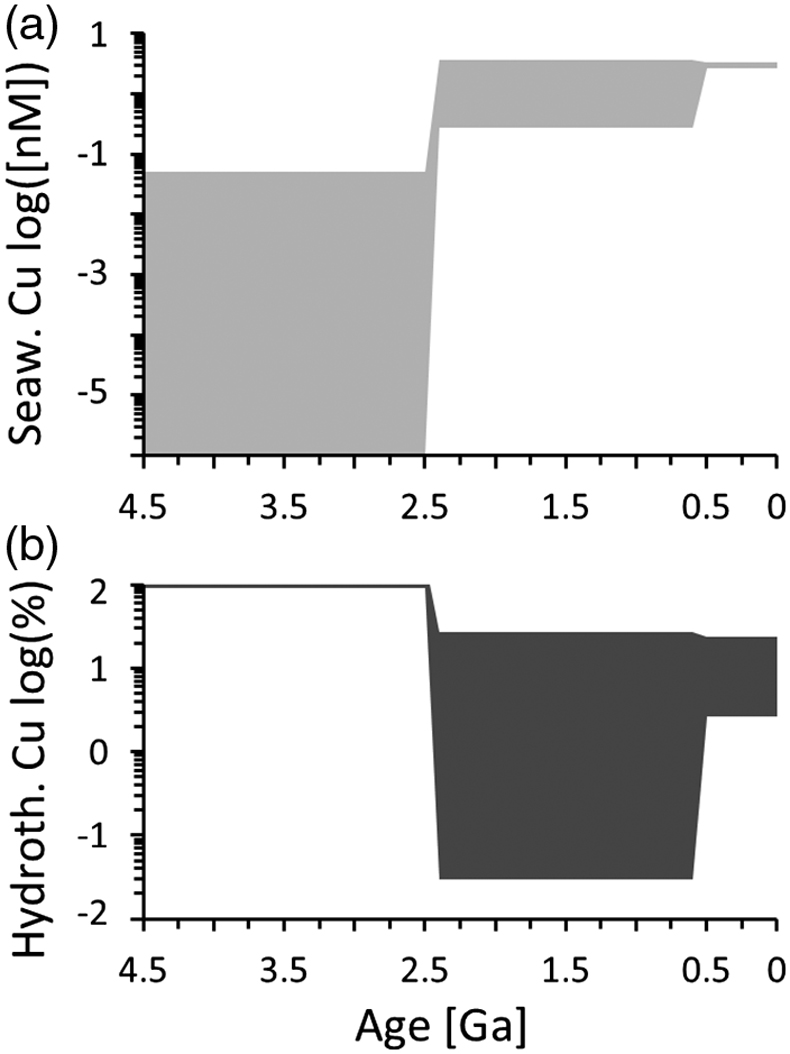
Figure 4 Compilation of (a) the total marine Cu concentration and (b) the hydrothermal contribution to the marine Cu budget through time. For the Archean, the lower bound is scenario 2 in Figure 1e; the upper bound corresponds to scenario 4 in Figure 1e with a 10-fold longer residence time (Fig. S-7). For the Proterozoic, the lower bound is scenario 2 in Figure 1c with a 10-fold shorter residence time (Fig. 1d) while the upper bound corresponds to scenario 5 in Figure 1c with a modern residence time.