Metamorphic olivine records external fluid infiltration during serpentinite dehydration
Affiliations | Corresponding Author | Cite as | Funding information- Share this article
-
Article views:191Cumulative count of HTML views and PDF downloads.
- Download Citation
- Rights & Permissions
top
Abstract
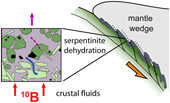
Figures
![]() Figure 1 Metamorphic facies map of the Western Alps (after Bousquet et al., 2012) showing study areas and sample locations. | ![]() Figure 2 δ11B data for olivine and serpentine in our samples. Error bars represent 1s uncertainties and include calibration uncertainties. Also indicated are B isotope compositions of various reservoirs relevant to subduction zones (data sources: Tonarini et al., 2011; De Hoog and Savov, 2018 and references therein; Martin et al., 2020). | ![]() Figure 3 Diagram comparing boron isotope systematics of co-existing metamorphic olivine and serpentine. [B]ol/srp = [B]olivine/[B]serpentine, and Δ11Bol-srp = δ11B of olivine – δ11B of serpentine. Large symbols with 1s error bars indicate averages for each sample, whereas small symbols represent all possible olivine-serpentine pairs for each sample (see Supplementary Information for details). A broadly negative correlation between [B]ol/srp and Δ11Bol-srp is observed. Grey bars indicate equilibrium fractionation of boron and its isotopes at relevant P-T conditions. | ![]() Figure 4 Schematic diagram depicting proposed fluid pathways and setting within the plate interface for serpentinite localities in this study after Gilio et al. (2019), where LCU and CdG indicate positions of Lago di Cignana and Cima di Gagnone HP ophiolites from that publication. |
Figure 1 | Figure 2 | Figure 3 | Figure 4 |
top
Introduction
The recycling of lithosphere-hosted volatile and fluid-mobile elements (e.g., H2O, CO2, B, halogens) back into the convecting mantle via subduction has been crucial for Earth’s evolution. Down-dragged mantle wedge serpentinites and hydrated oceanic mantle are water-rich (up to 13 wt. % H2O), and increasingly recognised as important sources of water and fluid-mobile elements (Deschamps et al., 2013
Deschamps, F., Godard, M., Guillot, S., Hattori, K. (2013) Geochemistry of subduction zone serpentinites: A review. Lithos 178, 96–127.
; Scambelluri et al., 2019Scambelluri, M., Cannaò, E., Gilio, M. (2019) The water and fluid-mobile element cycles during serpentinite subduction. A review. European Journal of Mineralogy 31, 405–428.
). However, many details of serpentinite dehydration in subduction zones are still poorly understood, including the relative roles of mantle wedge vs. ocean floor serpentinites (Martin et al., 2020Martin, C., Flores, K.E., Vitale-Brovarone, A., Angiboust, S., Harlow, G.E. (2020) Deep mantle serpentinization in subduction zones: Insight from in situ B isotopes in slab and mantle wedge serpentinites. Chemical Geology 545, 119637.
) and B isotope fractionation during serpentine breakdown (De Hoog et al., 2014De Hoog, J.C.M., Hattori, K., Jung, H. (2014) Titanium- and water-rich metamorphic olivine in high-pressure serpentinites from the Voltri Massif (Ligurian Alps, Italy): evidence for deep subduction of high-field strength and fluid-mobile elements. Contributions to Mineralogy and Petrology 167.
). Previous work on B behaviour during de-serpentinisation in subduction settings focussed on whole rock geochemistry (Harvey et al., 2014Harvey, J., Garrido, C.J., Savov, I., Agostini, S., Padrón-Navarta, J.A., Marchesi, C., Sánchez-Vizcaíno, V.L., Gómez-Pugnaire, M.T. (2014) 11B-rich fluids in subduction zones: The role of antigorite dehydration in subducting slabs and boron isotope heterogeneity in the mantle. Chemical Geology 376, 20–30.
; Cannaò et al., 2015Cannaò, E., Agostini, S., Scambelluri, M., Tonarini, S., Godard, M. (2015) B, Sr and Pb isotope geochemistry of high-pressure Alpine metaperidotites monitors fluid-mediated element recycling during serpentinite dehydration in subduction mélange (Cima di Gagnone, Swiss Central Alps). Geochimica et Cosmochimica Acta 163, 80–100.
), which obscures the detailed record of fluid-rock interaction preserved in individual mineral phases.Metamorphic olivine is formed during breakdown of brucite or antigorite (high pressure serpentine) at ∼400 °C and ∼650 °C, respectively (Scambelluri et al., 2004
Scambelluri, M., Müntener, O., Ottolini, L., Pettke, T.T., Vannucci, R. (2004) The fate of B, Cl and Li in the subducted oceanic mantle and in the antigorite breakdown fluids. Earth and Planetary Science Letters 222, 217–234.
) and is B-rich (up to 100 μg/g; Scambelluri et al., 2004Scambelluri, M., Müntener, O., Ottolini, L., Pettke, T.T., Vannucci, R. (2004) The fate of B, Cl and Li in the subducted oceanic mantle and in the antigorite breakdown fluids. Earth and Planetary Science Letters 222, 217–234.
; De Hoog et al., 2014De Hoog, J.C.M., Hattori, K., Jung, H. (2014) Titanium- and water-rich metamorphic olivine in high-pressure serpentinites from the Voltri Massif (Ligurian Alps, Italy): evidence for deep subduction of high-field strength and fluid-mobile elements. Contributions to Mineralogy and Petrology 167.
) compared to primary mantle olivine (<0.11 μg/g; Ottolini et al., 2004Ottolini, L., Le Fèvre, B., Vannucci, R. (2004) Direct assessment of mantle boron and lithium contents and distribution by SIMS analyses of peridotite minerals. Earth and Planetary Science Letters 228, 19–36.
). The incorporation of B in metamorphic olivine uniquely records fluid processes during serpentinite dehydration and allows insight into the composition, evolution and origin of these fluids.Here we present in situ δ11B data of co-existing serpentine and metamorphic olivine from high pressure serpentinites, which show significant disequilibrium between the two minerals. We interpret this as evidence for the infiltration of externally derived fluids during serpentinite dehydration, attesting to complex fluid pathways near the slab-wedge interface.
top
Samples and their Geological Setting
To capture the dehydration process, we selected eight serpentinite samples with phase assemblages between the brucite-out and antigorite-out phase transitions with 20–40 % modal olivine from three ophiolites in the Western Alps (Fig. 1). The Monviso Lago Superiore Unit (LSU) is a shear zone which underwent lawsonite-eclogite facies metamorphism and likely represents a serpentinite channel on the interface between the subducting plate and overlying mantle (Guillot et al., 2004
Guillot, S., Schwartz, S., Hattori, K., Auzende, A.L., Lardeaux, J. (2004) The Monviso ophiolitic massif (Western Alps), a section through a serpentinite subduction channel. Journal of the Virtual Explorer 16, 1–17.
). The Zermatt-Saas ophiolite represents relict oceanic lithosphere of the Mesozoic Tethys ocean subducted to ∼60–70 km: samples were obtained from near Zermatt and from Val d’Aosta. The Erro-Tobbio metaperidotites in the Voltri massif were subducted to 2.0–2.5 GPa and 550–600 °C and represent a subduction channel domain. See Supplementary Information for further sample details.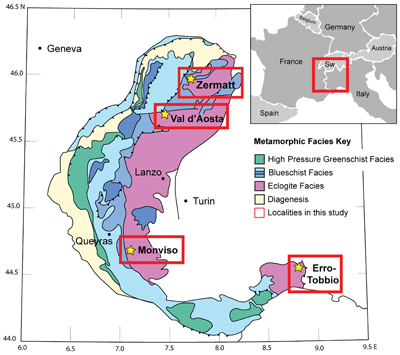
Figure 1 Metamorphic facies map of the Western Alps (after Bousquet et al., 2012
Bousquet, R., Oberhänsli, R., Schmid, S.M., Berger, A., Wiedenkehr, C.R., Möller, A., Rosenberg, C., Zeilinger, G., Molli, G., Koller, F. (2012) Metamorphic framework of the Alps. CCGM-CGMW, Paris.
) showing study areas and sample locations.top
Boron Isotope Systematics of Olivine and Serpentine
Boron isotope ratios and concentrations were measured by SIMS at the Edinburgh Ion Microprobe Facility. Matrix-matched standards including serpentine and olivine with known B isotope compositions were used for calibration. Typical uncertainty for B isotope analyses is ±1.6 ‰ (1s). Further analytical details and the complete dataset can be found in the Supplementary Information.
Olivine and serpentine show high δ11B (+6 to +28 ‰) in all samples (Fig. 2). Minor phases present in some samples include pyroxene, chlorite and clinohumite. Clinohumite has similar [B] as olivine (4.8 to 6.5 μg/g), but very low modal abundance. Pyroxene and chlorite have low [B] (<2 μg/g) compared to olivine and serpentine and can be ignored for the overall B budget.
On average, Monviso metamorphic olivine shows lower δ11B and higher [B] (δ11B = +8 to +14 ‰, [B] ∼16 μg/g) than co-existing serpentine (δ11B = +18 to +21 ‰, [B] ∼6 μg/g). Erro-Tobbio olivine has slightly higher δ11B and [B] (δ11B +18 to +20 ‰, [B] ∼11 μg/g) than co-existing serpentine (δ11B +16 to +17 ‰, [B] ∼10 μg/g). Zermatt olivine shows a wide range of δ11B (+16 to +28 ‰) and is higher on average than serpentine (δ11B = +6 to +21 ‰), but lower [B] (∼4 μg/g) in olivine compared to serpentine (∼8 μg/g). The Val d’Aosta sample has higher [B] in olivine vs. serpentine (22 vs. 10 μg/g) but somewhat lower δ11B (+17 to +19 ‰ in olivine, +16 to +21 ‰ in serpentine).
The most striking aspect of our dataset is the large variation in B isotope fractionation between olivine and serpentine (Δ11Bol-srp; δ11Bol – δ11Bsrp of co-existing olivine and serpentine), which shows that olivine is often not in isotopic equilibrium with serpentine, despite being its daughter product. Our dataset also shows a clear covariation between Δ11Bol-srp and B-enrichment in olivine ([B]ol/srp: [B]ol/[B]srp): Zermatt metamorphic olivine is depleted in B compared to co-existing serpentine but has heavier δ11B (Fig. 3). Monviso and Val d’Aosta olivine, however, is enriched in B compared to the co-existing serpentine but has lower δ11B. Erro-Tobbio olivine lies between these two extremes with roughly similar [B] and δ11B in olivine and serpentine. As the measured olivine is metamorphic and grew upon serpentinite dehydration, protolith composition and heterogeneity have no bearing on [B]ol/srp and Δ11Bol-srp, therefore processes operating during olivine growth are needed to explain these observations.
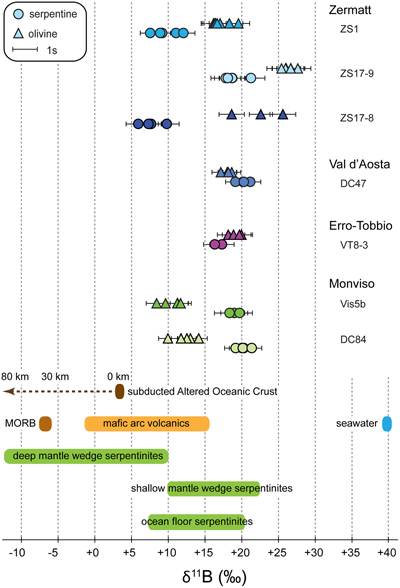
Figure 2 δ11B data for olivine and serpentine in our samples. Error bars represent 1s uncertainties and include calibration uncertainties. Also indicated are B isotope compositions of various reservoirs relevant to subduction zones (data sources: Tonarini et al., 2011
Tonarini, S., Leeman, W.P., Leat, P.T. (2011) Subduction erosion of forearc mantle wedge implicated in the genesis of the South Sandwich Island (SSI) arc: Evidence from boron isotope systematics. Earth and Planetary Science Letters 301, 275–284.
; De Hoog and Savov, 2018De Hoog, J.C.M., Savov, I.P. (2018) Boron Isotopes as a Tracer of Subduction Zone Processes. In: Marschall, H., Foster, G. (Eds.) Boron Isotopes: The Fifth Element. Advances in Isotope Geochemistry. Springer International Publishing, Cham, 217–247.
and references therein; Martin et al., 2020Martin, C., Flores, K.E., Vitale-Brovarone, A., Angiboust, S., Harlow, G.E. (2020) Deep mantle serpentinization in subduction zones: Insight from in situ B isotopes in slab and mantle wedge serpentinites. Chemical Geology 545, 119637.
).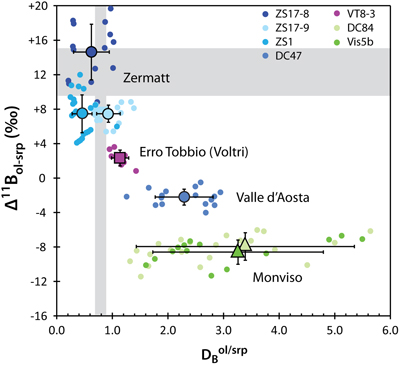
Figure 3 Diagram comparing boron isotope systematics of co-existing metamorphic olivine and serpentine. [B]ol/srp = [B]olivine/[B]serpentine, and Δ11Bol-srp = δ11B of olivine – δ11B of serpentine. Large symbols with 1s error bars indicate averages for each sample, whereas small symbols represent all possible olivine-serpentine pairs for each sample (see Supplementary Information for details). A broadly negative correlation between [B]ol/srp and Δ11Bol-srp is observed. Grey bars indicate equilibrium fractionation of boron and its isotopes at relevant P-T conditions.
top
Fluid Infiltration during Serpentine Dehydration
No partitioning data for B between serpentine and olivine (DBol/srp) is available, but a value of 3–5 for DBfluid/residue was recorded during serpentinite dehydration experiments with olivine as the main reservoir for B in the residue (Tenthorey and Hermann, 2004
Tenthorey, E., Hermann, J. (2004) Composition of fluids during serpentinite breakdown in subduction zones: Evidence for limited boron mobility. Geology 32, 865–868.
). Given this, mass balance dictates that DBol/srp ∼ 0.6–0.9 (see Supplementary Information), and higher values indicate excess B in olivine. Samples from Zermatt show [B]ol/srp 0.4–0.9, close to the expected equilibrium value. This is accompanied by Δ11Bol-srp of +7.4 to +14.6 ‰ (Fig. 3). In most silicates, including serpentine, B3+ substitutes for Si4+ or Al3+ in tetrahedral (IV) coordination (Hervig et al., 2002Hervig, R.L., Moore, G.M., Williams, L.B., Peacock, S.M., Holloway, J.R., Roggensack, K. (2002) Isotopic and elemental partitioning of boron between hydrous fluid and silicate melt. American Mineralogist 87, 769–774.
; Pabst et al., 2011Pabst, S., Zack, T., Savov, I.P., Ludwig, T., Rost, D., Vicenzi, E.P. (2011) Evidence for boron incorporation into the serpentine crystal structure. American Mineralogist 96, 1112–1119.
). In mantle silicates such as clinopyroxene and olivine, B also substitutes for Si4+ but occurs in trigonal (III) coordination as a BO3 group (Hålenius et al., 2010Hålenius, U., Skogby, H., Eden, M., Nazzareni, S., Kristiansson, P., Resmark, J. (2010) Coordination of boron in nominally boron-free rock forming silicates: Evidence for incorporation of BO3 groups in clinopyroxene. Geochimica et Cosmochimica Acta 74, 5672–5679.
; Ingrin et al., 2014Ingrin, J., Kovacs, I., Deloule, E., Balan, E., Blanchard, M., Kohn, S.C., Hermann, J. (2014) Identification of hydrogen defects linked to boron substitution in synthetic forsterite and natural oliyine. American Mineralogist 99, 2138–2141.
). As 11B preferentially resides in trigonal and 10B in tetrahedral coordination (Kowalski et al., 2013Kowalski, P.M., Wunder, B., Jahn, S. (2013) Ab initio prediction of equilibrium boron isotope fractionation between minerals and aqueous fluids at high P and T. Geochimica et Cosmochimica Acta 101, 285–301.
), olivine with trigonal B will show a heavier B isotope signature than co-existing serpentine with tetrahedral B. For the Zermatt samples, Δ11Bol-srp is close to the experimentally determined equilibrium B isotope fractionation between III and IV phases, which decreases from Δ11Bol-srp = +12.5 to +8.5 ‰ with T increasing from 450 to 650 °C (Kowalski et al., 2013Kowalski, P.M., Wunder, B., Jahn, S. (2013) Ab initio prediction of equilibrium boron isotope fractionation between minerals and aqueous fluids at high P and T. Geochimica et Cosmochimica Acta 101, 285–301.
).Therefore, we conclude that Zermatt metamorphic olivine was in equilibrium with internal fluids released during dehydration of serpentine, with no evidence for contamination by external fluids. This conclusion is supported by REE and Sr isotope data (Gilio et al., 2019
Gilio, M., Scambelluri, M., Agostini, S., Godard, M., Peters, D., Pettke, T. (2019) Petrology and geochemistry of serpentinites associated with the ultra-high pressure Lago di Cignana Unit (Italian Western Alps). Journal of Petrology 60, 1229–1262.
) and consistent with the geological setting of the Zermatt samples, which come from a thick (∼2 km) coherent section of subducted ocean lithosphere. The range in Δ11Bol-srp of +7.4 to +14.6 ‰ is probably related to metamorphic olivine forming at different temperatures during antigorite-out and brucite-out reactions, respectively.The majority of metamorphic olivines from localities other than Zermatt have [B]ol/srp > 1 and Δ11Bol-srp < +5 ‰, i.e. more B and 10B in olivine than is expected for growth during equilibrium dehydration of serpentine (Fig. 3). The most likely source of excess B is external fluid, as no other B-rich phases that dehydrate at 450–650 °C were present. Scarce clinohumite is B-rich but stable at the prevailing P–T conditions (Shen et al., 2015
Shen, T.T., Hermann, J., Zhang, L.F., Lu, Z., PadrÓn-Navarta, J.A., Xia, B., Bader, T. (2015) UHP metamorphism documented in Ti-chondrodite- and Ti-clinohumite-bearing serpentinized ultramafic rocks from Chinese Southwestern Tianshan. Journal of Petrology 56, 1425–1457.
) and its breakdown products (ilmenite, rutile) were not observed. Thus, we envisage a model where fluid from serpentine dehydration mixed with infiltrating external fluid, and metamorphic olivine grew in equilibrium with this mixed fluid. Serpentine did not re-equilibrate with this fluid due to sluggish kinetics at these low temperatures (450–650 °C) (e.g., Lafay et al., 2019Lafay, R., Baumgartner, L.P., Putlitz, B., Siron, G. (2019) Oxygen isotope disequilibrium during serpentinite dehydration. Terra Nova 31, 94–101.
). Mass balance calculations indicate that these external fluids had δ11B of +6 ‰ (Monviso) to +15 ‰ (Erro-Tobbio) depending on the B concentration of the external fluids (Fig. S-6) and assuming mildly alkaline pH conditions for both internal and external fluids (Galvez et al., 2016Galvez, M.E., Connolly, J.A.D., Manning, C.E. (2016) Implications for metal and volatile cycles from the pH of subduction zone fluids. Nature 539, 420–424.
; Debret and Sverjensky, 2017Debret, B., Sverjensky, D.A. (2017) Highly oxidising fluids generated during serpentinite breakdown in subduction zones. Scientific Reports 7, 10351.
). This matches the composition of fluids derived from a mixture of altered oceanic crust and sediments from 50–80 km depth (Tonarini et al., 2011Tonarini, S., Leeman, W.P., Leat, P.T. (2011) Subduction erosion of forearc mantle wedge implicated in the genesis of the South Sandwich Island (SSI) arc: Evidence from boron isotope systematics. Earth and Planetary Science Letters 301, 275–284.
; Yamada et al., 2019Yamada, C., Tsujimori, T., Chang, Q., Kimura, J.I. (2019) Boron isotope variations of Franciscan serpentinites, northern California. Lithos 334, 180–189.
), consistent with the depth of the serpentinites in the former subduction zone. The external fluids comprise at least ca. 15–45 % of the total fluid budget of the dehydrating serpentinites, which are minimum estimates, as any partial re-equilibration of serpentine would lead to underestimating the amount of external fluid.top
Metasomatism near the Subduction Interface
External fluids may permeate dehydrating serpentinites without the need for large scale faulting, as seen in Erro-Tobbio, which only shows metre scale vein networks and deformation. During serpentine dehydration there is a rapid increase in porosity and rock permeability allowing fluid to drain from the rock without the need for brittle failures and opening of fault pathways (Tenthorey and Cox, 2003
Tenthorey, E., Cox, S.F. (2003) Reaction-enhanced permeability during serpentinite dehydration. Geology 31, 921–924.
; Plümper et al., 2017Plümper, O., John, T., Podladchikov, Y.Y., Vrijmoed, J.C., Scambelluri, M. (2017) Fluid escape from subduction zones controlled by channel-forming reactive porosity. Nature Geoscience 10, 150–156.
). The creation of such porosity also provides pathways for significant volumes of B-rich external fluids to infiltrate.The trend towards higher [B]ol/srp with lower Δ11Bol-srp indicates an increasing influence of B-rich external fluids from Zermatt to Erro-Tobbio to Valle d’Aosta to Monviso. This trend correlates with the position of the serpentinite bodies relative to the slab-mantle interface during peak metamorphic conditions (Fig. 4). The Monviso ophiolite and Erro-Tobbio massif are thought to represent serpentinite domains between the subducting slab and the mantle wedge (Guillot et al., 2004
Guillot, S., Schwartz, S., Hattori, K., Auzende, A.L., Lardeaux, J. (2004) The Monviso ophiolitic massif (Western Alps), a section through a serpentinite subduction channel. Journal of the Virtual Explorer 16, 1–17.
; Scambelluri and Tonarini, 2012Scambelluri, M., Tonarini, S. (2012) Boron isotope evidence for shallow fluid transfer across subduction zones by serpentinized mantle. Geology 40, 907–910.
); a prime location to be infiltrated by fluids derived from the subducted slab. Shear zone associated faulting provides many additional pathways for fluid flow throughout the Monviso ophiolite, leaving the serpentinites exposed to extensive interaction with external fluids (Angiboust et al., 2014Angiboust, S., Pettke, T., De Hoog, J.C.M., Caron, B., Oncken, O. (2014) Channelized fluid flow and eclogite-facies metasomatism along the subduction shear zone. Journal of Petrology 55, 883–916.
; Gilio et al., 2020Gilio, M., Scambelluri, M., Agostini, S., Godard, M., Pettke, T., Agard, P., Locatelli, M., Angiboust, S. (2020) Fingerprinting and relocating tectonic slices along the plate interface: Evidence from the Lago Superiore unit at Monviso (Western Alps). Lithos 352, 105308
). The Val d’Aosta sample is considered part of the Zermatt-Saas ophiolite but at Val d’Aosta the serpentinite is thinner (∼0.5 km) and surrounded by crustal lithologies (metasediments and metabasalts). This sample shows elevated [B]ol/srp and lower Δ11Bol-srp compared to the other Zermatt samples, likely related to its proximity to other lithologies and increased exposure to dehydration fluids.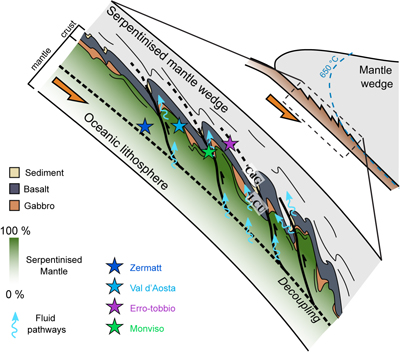
Figure 4 Schematic diagram depicting proposed fluid pathways and setting within the plate interface for serpentinite localities in this study after Gilio et al. (2019
Gilio, M., Scambelluri, M., Agostini, S., Godard, M., Peters, D., Pettke, T. (2019) Petrology and geochemistry of serpentinites associated with the ultra-high pressure Lago di Cignana Unit (Italian Western Alps). Journal of Petrology 60, 1229–1262.
), where LCU and CdG indicate positions of Lago di Cignana and Cima di Gagnone HP ophiolites from that publication.The micro-scale process where metamorphic olivine grows from a mixture of internal and external fluids would not be detected using whole rock δ11B data, as serpentinites in this study have positive whole rock δ11B (e.g., Scambelluri and Tonarini, 2012
Scambelluri, M., Tonarini, S. (2012) Boron isotope evidence for shallow fluid transfer across subduction zones by serpentinized mantle. Geology 40, 907–910.
) whilst being variably affected by external fluid infiltration. In contrast, subduction mélange serpentinites and metaperidotites with negative δ11B suffered more extensive contamination and extreme δ11B overprinting consistent with their close association with large proportions of crustal rocks and sediments (Cannaò et al., 2015Cannaò, E., Agostini, S., Scambelluri, M., Tonarini, S., Godard, M. (2015) B, Sr and Pb isotope geochemistry of high-pressure Alpine metaperidotites monitors fluid-mediated element recycling during serpentinite dehydration in subduction mélange (Cima di Gagnone, Swiss Central Alps). Geochimica et Cosmochimica Acta 163, 80–100.
; Martin et al., 2020Martin, C., Flores, K.E., Vitale-Brovarone, A., Angiboust, S., Harlow, G.E. (2020) Deep mantle serpentinization in subduction zones: Insight from in situ B isotopes in slab and mantle wedge serpentinites. Chemical Geology 545, 119637.
).top
Conclusions
Metamorphic olivine is a sensitive recorder of the fluid composition from which it grew, and recorded extensive external fluid infiltration during subduction dehydration of Alpine serpentinites, which are sinks and carriers of crust-derived fluid mobile elements. External fluid infiltration was most prominent in serpentinites close to the subduction interface and amounted to up to 45 % of the total dehydration fluid budget. The study of B isotope systematics of texturally co-existing olivine and serpentine provides a powerful tool to study fluid flow deep in subduction zones, and reveals processes not visible in whole rock δ11B compositions.
top
Acknowledgements
The authors would like to thank Lukas Baumgartner for his generous help during field work, Marco Scambelluri and Tatsuki Tsujimori for constructive reviews, Horst Marschall for editorial handling, Samuele Agostini, Ray Burgess and Katy Evans for helpful comments on earlier versions of this manuscript, and the NERC ‘Deep Volatiles’ consortium (NE/M000427/1), NERC-IMF (IMF571/1015; IMF595/0516) and E3-DTP for funding.
Editor: Horst R. Marschall
top
References
Angiboust, S., Pettke, T., De Hoog, J.C.M., Caron, B., Oncken, O. (2014) Channelized fluid flow and eclogite-facies metasomatism along the subduction shear zone. Journal of Petrology 55, 883–916.

Shear zone associated faulting provides many additional pathways for fluid flow throughout the Monviso ophiolite, leaving the serpentinites exposed to extensive interaction with external fluids (Angiboust et al., 2014; Gilio et al., 2020).
View in article
Bousquet, R., Oberhänsli, R., Schmid, S.M., Berger, A., Wiedenkehr, C.R., Möller, A., Rosenberg, C., Zeilinger, G., Molli, G., Koller, F. (2012) Metamorphic framework of the Alps. CCGM-CGMW, Paris.

Metamorphic facies map of the Western Alps (after Bousquet et al., 2012) showing study areas and sample locations.
View in article
Cannaò, E., Agostini, S., Scambelluri, M., Tonarini, S., Godard, M. (2015) B, Sr and Pb isotope geochemistry of high-pressure Alpine metaperidotites monitors fluid-mediated element recycling during serpentinite dehydration in subduction mélange (Cima di Gagnone, Swiss Central Alps). Geochimica et Cosmochimica Acta 163, 80–100.

Previous work on B behaviour during de-serpentinisation in subduction settings focussed on whole rock geochemistry (Harvey et al., 2014; Cannaò et al., 2015), which obscures the detailed record of fluid-rock interaction preserved in individual mineral phases.
View in article
In contrast, subduction mélange serpentinites and metaperidotites with negative δ11B suffered more extensive contamination and extreme δ11B overprinting consistent with their close association with large proportions of crustal rocks and sediments (Cannaò et al., 2015; Martin et al., 2020).
View in article
De Hoog, J.C.M., Savov, I.P. (2018) Boron Isotopes as a Tracer of Subduction Zone Processes. In: Marschall, H., Foster, G. (Eds.) Boron Isotopes: The Fifth Element. Advances in Isotope Geochemistry. Springer International Publishing, Cham, 217–247.

Also indicated are B isotope compositions of various reservoirs relevant to subduction zones (data sources: Tonarini et al., 2011; De Hoog and Savov, 2018 and references therein; Martin et al., 2020).
View in article
De Hoog, J.C.M., Hattori, K., Jung, H. (2014) Titanium- and water-rich metamorphic olivine in high-pressure serpentinites from the Voltri Massif (Ligurian Alps, Italy): evidence for deep subduction of high-field strength and fluid-mobile elements. Contributions to Mineralogy and Petrology 167.

However, many details of serpentinite dehydration in subduction zones are still poorly understood, including the relative roles of mantle wedge vs. ocean floor serpentinites (Martin et al., 2020) and B isotope fractionation during serpentine breakdown (De Hoog et al., 2014).
View in article
Metamorphic olivine is formed during breakdown of brucite or antigorite (high pressure serpentine) at ∼400 °C and ∼650 °C, respectively (Scambelluri et al., 2004) and is B-rich (up to 100 μg/g; Scambelluri et al., 2004; De Hoog et al., 2014) compared to primary mantle olivine (<0.11 μg/g; Ottolini et al., 2004).
View in article
Debret, B., Sverjensky, D.A. (2017) Highly oxidising fluids generated during serpentinite breakdown in subduction zones. Scientific Reports 7, 10351.

Mass balance calculations indicate that these external fluids had δ11B of +6 ‰ (Monviso) to +15 ‰ (Erro-Tobbio) depending on the B concentration of the external fluids (Fig. S-6) and assuming mildly alkaline pH conditions for both internal and external fluids (Galvez et al., 2016; Debret and Sverjensky, 2017).
View in article
Deschamps, F., Godard, M., Guillot, S., Hattori, K. (2013) Geochemistry of subduction zone serpentinites: A review. Lithos 178, 96–127.

Down-dragged mantle wedge serpentinites and hydrated oceanic mantle are water-rich (up to 13 wt. % H2O), and increasingly recognised as important sources of water and fluid-mobile elements (Deschamps et al., 2013; Scambelluri et al., 2019).
View in article
Galvez, M.E., Connolly, J.A.D., Manning, C.E. (2016) Implications for metal and volatile cycles from the pH of subduction zone fluids. Nature 539, 420–424.

Mass balance calculations indicate that these external fluids had δ11B of +6 ‰ (Monviso) to +15 ‰ (Erro-Tobbio) depending on the B concentration of the external fluids (Fig. S-6) and assuming mildly alkaline pH conditions for both internal and external fluids (Galvez et al., 2016; Debret and Sverjensky, 2017).
View in article
Gilio, M., Scambelluri, M., Agostini, S., Godard, M., Peters, D., Pettke, T. (2019) Petrology and geochemistry of serpentinites associated with the ultra-high pressure Lago di Cignana Unit (Italian Western Alps). Journal of Petrology 60, 1229–1262.

This conclusion is supported by REE and Sr isotope data (Gilio et al., 2019) and consistent with the geological setting of the Zermatt samples, which come from a thick (∼2 km) coherent section of subducted ocean lithosphere. The range in Δ11Bol-srp of +7.4 to +14.6 ‰ is probably related to metamorphic olivine forming at different temperatures during antigorite-out and brucite-out reactions, respectively.
View in article
Schematic diagram depicting proposed fluid pathways and setting within the plate interface for serpentinite localities in this study after Gilio et al. (2019), where LCU and CdG indicate positions of Lago di Cignana and Cima di Gagnone HP ophiolites from that publication.
View in article
Gilio, M., Scambelluri, M., Agostini, S., Godard, M., Pettke, T., Agard, P., Locatelli, M., Angiboust, S. (2020) Fingerprinting and relocating tectonic slices along the plate interface: Evidence from the Lago Superiore unit at Monviso (Western Alps). Lithos 352, 105308.

Shear zone associated faulting provides many additional pathways for fluid flow throughout the Monviso ophiolite, leaving the serpentinites exposed to extensive interaction with external fluids (Angiboust et al., 2014; Gilio et al., 2020).
View in article
Guillot, S., Schwartz, S., Hattori, K., Auzende, A.L., Lardeaux, J. (2004) The Monviso ophiolitic massif (Western Alps), a section through a serpentinite subduction channel. Journal of the Virtual Explorer 16, 1–17.

The Monviso Lago Superiore Unit (LSU) is a shear zone which underwent lawsonite-eclogite facies metamorphism and likely represents a serpentinite channel on the interface between the subducting plate and overlying mantle (Guillot et al., 2004).
View in article
The Monviso ophiolite and Erro-Tobbio massif are thought to represent serpentinite domains between the subducting slab and the mantle wedge (Guillot et al., 2004; Scambelluri and Tonarini, 2012); a prime location to be infiltrated by fluids derived from the subducted slab.
View in article
Hålenius, U., Skogby, H., Eden, M., Nazzareni, S., Kristiansson, P., Resmark, J. (2010) Coordination of boron in nominally boron-free rock forming silicates: Evidence for incorporation of BO3 groups in clinopyroxene. Geochimica et Cosmochimica Acta 74, 5672–5679.

In mantle silicates such as clinopyroxene and olivine, B also substitutes for Si4+ but occurs in trigonal (III) coordination as a BO3 group (Hålenius et al., 2010; Ingrin et al., 2014).
View in article
Harvey, J., Garrido, C.J., Savov, I., Agostini, S., Padrón-Navarta, J.A., Marchesi, C., Sánchez-Vizcaíno, V.L., Gómez-Pugnaire, M.T. (2014) 11B-rich fluids in subduction zones: The role of antigorite dehydration in subducting slabs and boron isotope heterogeneity in the mantle. Chemical Geology 376, 20–30.

Previous work on B behaviour during de-serpentinisation in subduction settings focussed on whole rock geochemistry (Harvey et al., 2014; Cannaò et al., 2015), which obscures the detailed record of fluid-rock interaction preserved in individual mineral phases.
View in article
Hervig, R.L., Moore, G.M., Williams, L.B., Peacock, S.M., Holloway, J.R., Roggensack, K. (2002) Isotopic and elemental partitioning of boron between hydrous fluid and silicate melt. American Mineralogist 87, 769–774.

In most silicates, including serpentine, B3+ substitutes for Si4+ or Al3+ in tetrahedral (IV) coordination (Hervig et al., 2002; Pabst et al., 2011).
View in article
Ingrin, J., Kovacs, I., Deloule, E., Balan, E., Blanchard, M., Kohn, S.C., Hermann, J. (2014) Identification of hydrogen defects linked to boron substitution in synthetic forsterite and natural oliyine. American Mineralogist 99, 2138–2141.

In mantle silicates such as clinopyroxene and olivine, B also substitutes for Si4+ but occurs in trigonal (III) coordination as a BO3 group (Hålenius et al., 2010; Ingrin et al., 2014).
View in article
Kowalski, P.M., Wunder, B., Jahn, S. (2013) Ab initio prediction of equilibrium boron isotope fractionation between minerals and aqueous fluids at high P and T. Geochimica et Cosmochimica Acta 101, 285–301.

As 11B preferentially resides in trigonal and 10B in tetrahedral coordination (Kowalski et al., 2013), olivine with trigonal B will show a heavier B isotope signature than co-existing serpentine with tetrahedral B.
View in article
For the Zermatt samples, Δ11Bol-srp is close to the experimentally determined equilibrium B isotope fractionation between III and IV phases, which decreases from Δ11Bol-srp = +12.5 to +8.5 ‰ with T increasing from 450 to 650 °C (Kowalski et al., 2013).
View in article
Lafay, R., Baumgartner, L.P., Putlitz, B., Siron, G. (2019) Oxygen isotope disequilibrium during serpentinite dehydration. Terra Nova 31, 94–101.

Serpentine did not re-equilibrate with this fluid due to sluggish kinetics at these low temperatures (450–650 °C) (e.g., Lafay et al., 2019).
View in article
Martin, C., Flores, K.E., Vitale-Brovarone, A., Angiboust, S., Harlow, G.E. (2020) Deep mantle serpentinization in subduction zones: Insight from in situ B isotopes in slab and mantle wedge serpentinites. Chemical Geology 545, 119637.

However, many details of serpentinite dehydration in subduction zones are still poorly understood, including the relative roles of mantle wedge vs. ocean floor serpentinites (Martin et al., 2020) and B isotope fractionation during serpentine breakdown (De Hoog et al., 2014).
View in article
In contrast, subduction mélange serpentinites and metaperidotites with negative δ11B suffered more extensive contamination and extreme δ11B overprinting consistent with their close association with large proportions of crustal rocks and sediments (Cannaò et al., 2015; Martin et al., 2020).
View in article
Also indicated are B isotope compositions of various reservoirs relevant to subduction zones (data sources: Tonarini et al., 2011; De Hoog and Savov, 2018 and references therein; Martin et al., 2020).
View in article
Ottolini, L., Le Fèvre, B., Vannucci, R. (2004) Direct assessment of mantle boron and lithium contents and distribution by SIMS analyses of peridotite minerals. Earth and Planetary Science Letters 228, 19–36.

Metamorphic olivine is formed during breakdown of brucite or antigorite (high pressure serpentine) at ∼400 °C and ∼650 °C, respectively (Scambelluri et al., 2004) and is B-rich (up to 100 μg/g; Scambelluri et al., 2004; De Hoog et al., 2014) compared to primary mantle olivine (<0.11 μg/g; Ottolini et al., 2004).
View in article
Pabst, S., Zack, T., Savov, I.P., Ludwig, T., Rost, D., Vicenzi, E.P. (2011) Evidence for boron incorporation into the serpentine crystal structure. American Mineralogist 96, 1112–1119.

In most silicates, including serpentine, B3+ substitutes for Si4+ or Al3+ in tetrahedral (IV) coordination (Hervig et al., 2002; Pabst et al., 2011).
View in article
Plümper, O., John, T., Podladchikov, Y.Y., Vrijmoed, J.C., Scambelluri, M. (2017) Fluid escape from subduction zones controlled by channel-forming reactive porosity. Nature Geoscience 10, 150–156.

During serpentine dehydration there is a rapid increase in porosity and rock permeability allowing fluid to drain from the rock without the need for brittle failures and opening of fault pathways (Tenthorey and Cox, 2003; Plümper et al., 2017).
View in article
Scambelluri, M., Tonarini, S. (2012) Boron isotope evidence for shallow fluid transfer across subduction zones by serpentinized mantle. Geology 40, 907–910.

The Monviso ophiolite and Erro-Tobbio massif are thought to represent serpentinite domains between the subducting slab and the mantle wedge (Guillot et al., 2004; Scambelluri and Tonarini, 2012); a prime location to be infiltrated by fluids derived from the subducted slab.
View in article
The micro-scale process where metamorphic olivine grows from a mixture of internal and external fluids would not be detected using whole rock δ11B data, as serpentinites in this study have positive whole rock δ11B (e.g., Scambelluri and Tonarini, 2012) whilst being variably affected by external fluid infiltration.
View in article
Scambelluri, M., Müntener, O., Ottolini, L., Pettke, T.T., Vannucci, R. (2004) The fate of B, Cl and Li in the subducted oceanic mantle and in the antigorite breakdown fluids. Earth and Planetary Science Letters 222, 217–234.

Metamorphic olivine is formed during breakdown of brucite or antigorite (high pressure serpentine) at ∼400 °C and ∼650 °C, respectively (Scambelluri et al., 2004) and is B-rich (up to 100 μg/g; Scambelluri et al., 2004; De Hoog et al., 2014) compared to primary mantle olivine (<0.11 μg/g; Ottolini et al., 2004).
View in article
Scambelluri, M., Cannaò, E., Gilio, M. (2019) The water and fluid-mobile element cycles during serpentinite subduction. A review. European Journal of Mineralogy 31, 405–428.

Down-dragged mantle wedge serpentinites and hydrated oceanic mantle are water-rich (up to 13 wt. % H2O), and increasingly recognised as important sources of water and fluid-mobile elements (Deschamps et al., 2013; Scambelluri et al., 2019).
View in article
Shen, T.T., Hermann, J., Zhang, L.F., Lu, Z., PadrÓn-Navarta, J.A., Xia, B., Bader, T. (2015) UHP metamorphism documented in Ti-chondrodite- and Ti-clinohumite-bearing serpentinized ultramafic rocks from Chinese Southwestern Tianshan. Journal of Petrology 56, 1425–1457.

Scarce clinohumite is B-rich but stable at the prevailing P–T conditions (Shen et al., 2015) and its breakdown products (ilmenite, rutile) were not observed.
View in article
Tenthorey, E., Cox, S.F. (2003) Reaction-enhanced permeability during serpentinite dehydration. Geology 31, 921–924.

During serpentine dehydration there is a rapid increase in porosity and rock permeability allowing fluid to drain from the rock without the need for brittle failures and opening of fault pathways (Tenthorey and Cox, 2003; Plümper et al., 2017).
View in article
Tenthorey, E., Hermann, J. (2004) Composition of fluids during serpentinite breakdown in subduction zones: Evidence for limited boron mobility. Geology 32, 865–868.

No partitioning data for B between serpentine and olivine (DBol/srp) is available, but a value of 3–5 for DBfluid/residue was recorded during serpentinite dehydration experiments with olivine as the main reservoir for B in the residue (Tenthorey and Hermann, 2004).
View in article
Tonarini, S., Leeman, W.P., Leat, P.T. (2011) Subduction erosion of forearc mantle wedge implicated in the genesis of the South Sandwich Island (SSI) arc: Evidence from boron isotope systematics. Earth and Planetary Science Letters 301, 275–284.

Also indicated are B isotope compositions of various reservoirs relevant to subduction zones (data sources: Tonarini et al., 2011; De Hoog and Savov, 2018 and references therein; Martin et al., 2020).
View in article
This matches the composition of fluids derived from a mixture of altered oceanic crust and sediments from 50–80 km depth (Tonarini et al., 2011; Yamada et al., 2019), consistent with the depth of the serpentinites in the former subduction zone.
View in article
Yamada, C., Tsujimori, T., Chang, Q., Kimura, J.I. (2019) Boron isotope variations of Franciscan serpentinites, northern California. Lithos 334, 180–189.

This matches the composition of fluids derived from a mixture of altered oceanic crust and sediments from 50–80 km depth (Tonarini et al., 2011; Yamada et al., 2019), consistent with the depth of the serpentinites in the former subduction zone.
View in article
top
Supplementary Information
The Supplementary Information includes:
- 1. Analytical Methods
- 2. Geological Context of Samples
- 3. Sample Descriptions
- 4. Background Geochemical Data
- 5. Average [B]ol/srp and Δ11Bol-srp Calculations
- 6. Estimate of Equilibrium DBol/srp
- 7. Modelling of External Fluid Composition
- Figures S-1 to S-6
- Tables S-1 to S-6
- Supplementary Information References
Download the Supplementary Information (PDF).
Figures
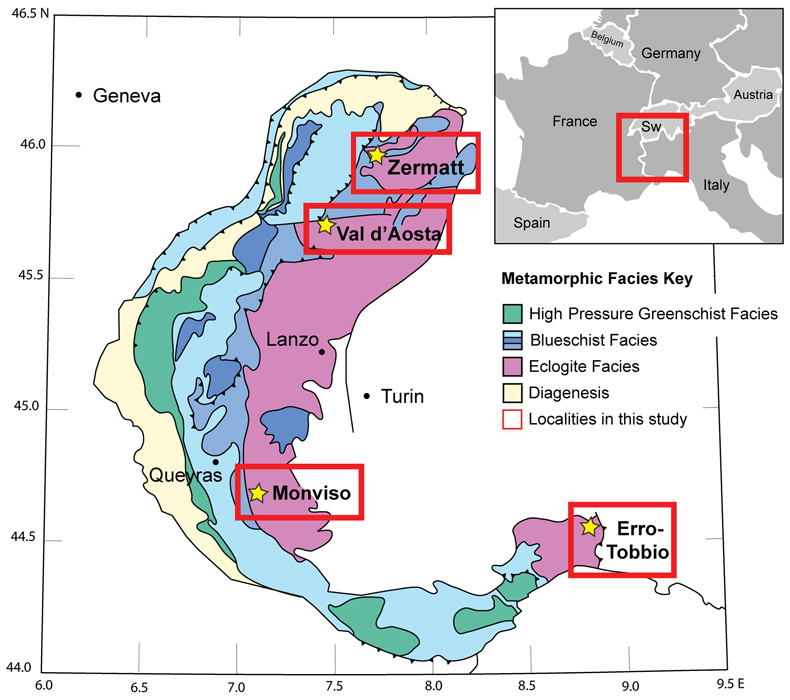
Figure 1 Metamorphic facies map of the Western Alps (after Bousquet et al., 2012
Bousquet, R., Oberhänsli, R., Schmid, S.M., Berger, A., Wiedenkehr, C.R., Möller, A., Rosenberg, C., Zeilinger, G., Molli, G., Koller, F. (2012) Metamorphic framework of the Alps. CCGM-CGMW, Paris.
) showing study areas and sample locations.
Figure 2 δ11B data for olivine and serpentine in our samples. Error bars represent 1s uncertainties and include calibration uncertainties. Also indicated are B isotope compositions of various reservoirs relevant to subduction zones (data sources: Tonarini et al., 2011
Tonarini, S., Leeman, W.P., Leat, P.T. (2011) Subduction erosion of forearc mantle wedge implicated in the genesis of the South Sandwich Island (SSI) arc: Evidence from boron isotope systematics. Earth and Planetary Science Letters 301, 275–284.
; De Hoog and Savov, 2018De Hoog, J.C.M., Savov, I.P. (2018) Boron Isotopes as a Tracer of Subduction Zone Processes. In: Marschall, H., Foster, G. (Eds.) Boron Isotopes: The Fifth Element. Advances in Isotope Geochemistry. Springer International Publishing, Cham, 217–247.
and references therein; Martin et al., 2020Martin, C., Flores, K.E., Vitale-Brovarone, A., Angiboust, S., Harlow, G.E. (2020) Deep mantle serpentinization in subduction zones: Insight from in situ B isotopes in slab and mantle wedge serpentinites. Chemical Geology 545, 119637.
).
Figure 3 Diagram comparing boron isotope systematics of co-existing metamorphic olivine and serpentine. [B]ol/srp = [B]olivine/[B]serpentine, and Δ11Bol-srp = δ11B of olivine – δ11B of serpentine. Large symbols with 1s error bars indicate averages for each sample, whereas small symbols represent all possible olivine-serpentine pairs for each sample (see Supplementary Information for details). A broadly negative correlation between [B]ol/srp and Δ11Bol-srp is observed. Grey bars indicate equilibrium fractionation of boron and its isotopes at relevant P-T conditions.

Figure 4 Schematic diagram depicting proposed fluid pathways and setting within the plate interface for serpentinite localities in this study after Gilio et al. (2019
Gilio, M., Scambelluri, M., Agostini, S., Godard, M., Peters, D., Pettke, T. (2019) Petrology and geochemistry of serpentinites associated with the ultra-high pressure Lago di Cignana Unit (Italian Western Alps). Journal of Petrology 60, 1229–1262.
), where LCU and CdG indicate positions of Lago di Cignana and Cima di Gagnone HP ophiolites from that publication.