Morphology dominated rapid oxidation of framboidal pyrite
Affiliations | Corresponding Author | Cite as | Funding information- Share this article
-
Article views:187Cumulative count of HTML views and PDF downloads.
- Download Citation
- Rights & Permissions
top
Abstract
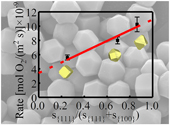
Figures
![]() Figure 1 (a–c) X-ray diffraction patterns of synthesised microscopic pyrite samples. (d–f) Field emission scanning electron microscope images of synthesised pyrite samples. (g–i) Energy dispersive spectrometry results of the pyrite samples. The half-quantitative data shown in the spectra were derived from the average measurements of five points. (j–l) Particle size distributions of the pyrite samples, which were derived from the statistical results of at least 500 grains from the micrographs. | ![]() Figure 2 S 2p (a–f) and Fe 2p3/2 (g–l) X-ray photoelectron spectroscopy spectra of Py-tc (truncated cubic pyrite), Py-co (cubo-octahedral pyrite), and Py-to (truncated octahedral pyrite) surfaces oxidised in air with a relative humidity of 77 % at intervals of 0 hr and 84 hr. The Fe-surf.-I and Fe-surf.-II denote quadruplet and triplet surface states of iron on pyrite, respectively. | ![]() Figure 3 Plots of the concentration ratios of oxidised species to unoxidised species (Coxidised/Cunoxidised) vs. oxidation time (t) from (a) S 2p and (b) Fe 2p3/2. X-ray photoelectron spectroscopy spectra are of Py-tc (truncated cubic pyrite), Py-co (cubo-octahedral pyrite), and Py-to (truncated octahedral pyrite). (c) The estimated oxidation rates of the samples are proportional to the ratio of microscopic pyrite {111} facets to {100} facets. | ![]() Figure 4 (a) Core-shell models of pyrite with different facets under two redox conditions. The red zone is the oxidised shell and the yellow zone is the unoxidised pyrite core. (b,c) Core-shell morphologies of oxidised natural pyrites from the Zhijin phosphorus deposit in Guizhou Province, China. |
Figure 1 | Figure 2 | Figure 3 | Figure 4 |
top
Introduction
Sedimentary sulfides account for more than 95 % of the sulfides on the surface of the Earth and mainly occur as pyrite in sediments (Rickard et al., 2017
Rickard, D., Mussmann, M., Steadman, J.A. (2017) Sedimentary Sulfides. Elements 13, 117–122.
). The formation and oxidative weathering of sedimentary pyrite are core processes in the sulfur cycle and are simultaneously involved in other major element cycles (such as carbon, nitrogen, and oxygen) (Hayakawa et al., 2013Hayakawa, A., Hatakeyama, M., Asano, R., Ishikawa, Y., Hidaka, S. (2013) Nitrate reduction coupled with pyrite oxidation in the surface sediments of a sulfide-rich ecosystem. Journal of Geophysical Research: Biogeosciences 118, 639–649.
; Fike et al., 2015Fike, D.A., Bradley, A.S., Rose, C.V. (2015) Rethinking the Ancient Sulfur Cycle. Annual Review of Earth and Planetary Sciences 43, 593–622.
; Fakhraee et al., 2019Fakhraee, M., Hancisse, O., Canfield, D.E., Crowe, S.A., Katsev, S. (2019) Proterozoic seawater sulfate scarcity and the evolution of ocean–atmosphere chemistry. Nature Geoscience 12, 375–380.
). Thus, these processes have regulated the redox conditions of the Earth’s surface through geological history, especially oceanic redox conditions (Wang et al., 2018Wang, P., Tian, J., Huang, E. (2018) Earth system and evolution. Science Press, Beijing.
). Changes in redox conditions also affect the distribution of elements (Anbar, 2008Anbar, A.D. (2008) Elements and Evolution. Science 322, 1481–1483.
).Framboidal pyrite, which is the main component and dominant pyrite texture of sedimentary pyrite (Wilkin et al., 1996
Wilkin, R.T., Barnes, H.L., Brantley, S.L. (1996) The size distribution of framboidal pyrite in modern sediments: An indicator of redox conditions. Geochimica et Cosmochimica Acta 60, 3897–3912.
; Sawlowicz, 2000Sawlowicz, Z. (2000) Framboids: From their origin to application. Prace Mineralogiczne, Kraków, Poland.
; Rickard, 2012Rickard, D. (2012) Sulfidic Sediments and Sedimentary Rocks. Elsevier, Amsterdam, Netherlands.
), plays a major role in the oxidation of sedimentary pyrite. Billions of pyrite grains are forming in sediments and waterways worldwide every second (Ohfuji and Rickard, 2005Ohfuji, H., Rickard, D. (2005) Experimental syntheses of framboids—a review. Earth-Science Reviews 71, 147–170.
; Rickard, 2015Rickard, D. (2015) Pyrite: A Natural History of Fool’s Gold. Oxford University Press, New York.
, 2019aRickard, D. (2019a) How long does it take a pyrite framboid to form? Earth and Planetary Science Letters 513, 64–68.
,bRickard, D. (2019b) Sedimentary pyrite framboid size-frequency distributions: A meta-analysis. Palaeogeography, Palaeoclimatology, Palaeoecology 522, 62–75.
). The oldest framboidal pyrite may be found in the late Archean (≤2.9 Ga) sediments. (Hallbauer, 1986Hallbauer, D.K. (1986) The mineralogy and geochemistry of Witwatersrand pyrite gold, uranium and carbonaceous matter mineral deposits of South Africa. In: Anhauesser, C.R., Maske, S. (Eds.) Mineral Deposits of Southern Africa. Geological Society of South Africa, South Africa, Vol. 1, 731–752.
; Guy et al., 2010Guy, B.M., Beukes, N.J., Gutzmer, J. (2010) Paleoenvironmental controls on the texture and chemical composition of pyrite from non-conglomeratic sedimentary rocks of the Mesoarchean Witwatersrand Supergroup, South Africa. South African Journal of Geology 113, 195–228.
).Pyrite is easily oxidised when exposed to the Earth’s oxygenic atmosphere and oceans. The pyrite oxidation process has been investigated extensively for decades, particularly the reaction kinetics and oxidation mechanism. Most previous studies mainly focused on powder samples, i.e. fracture surfaces, and ignored crystal facet effects (Zhu et al., 2018
Zhu, J., Xian, H., Lin, X., Tang, H., Du, R., Yang, Y., Zhu, R., Liang, X., Wei, J., Teng, H., He, H. (2018) Surface structure-dependent pyrite oxidation in relatively dry and moist air: Implications for the reaction mechanism and sulfur evolution. Geochimica et Cosmochimica Acta 228, 259–274.
), which may have hindered the accurate determination of the pyrite oxidation mechanism. Moreover, little attention has been paid to the oxidative behaviour of framboidal pyrite, despite its importance in sediments. The oxidation of framboidal pyrite is considered to be fast due to its high specific surface area (SSA) (Pugh et al., 1984Pugh, C.E., Hossner, L.R., Dixon, J.B. (1984) Oxidation rate of iron sulfides as affected by surface area, morphology, oxygen concentration and autotrophic bacteria. Soil Science 137, 309–314.
; Weber et al., 2004Weber, P.A., Stewart, W.A., Skinner, W.M., Weisener, C.G., Thomas, J.E., Smart, R.St.C. (2004) Geochemical effects of oxidation products and framboidal pyrite oxidation in acid mine drainage prediction techniques. Applied Geochemistry 19, 1953–1974.
), and the influence of the crystal shape is often neglected. Several studies have shown that framboidal pyrite grains are mostly euhedral microcrystals with specific shapes (mainly with {100} and {111} facets) (Ohfuji and Rickard, 2005Ohfuji, H., Rickard, D. (2005) Experimental syntheses of framboids—a review. Earth-Science Reviews 71, 147–170.
; Rigby et al., 2006Rigby, P.A., Dobos, S.K., Cook, F.J., Goonetilleke, A. (2006) Role of organic matter in framboidal pyrite oxidation. Science of The Total Environment 367, 847–854.
; Kozina et al., 2018Kozina, N., Reykhard, L., Dara, O., Gordeev, V. (2018) Framboidal pyrite formation in the bottom sediments of the South Caspian Basin under conditions of hydrogen sulfide contamination. Russian Journal of Earth Sciences 18, 1–10.
). Poorly understood issues regarding the rapid oxidation of framboidal pyrite are what controls the oxidation processes; the unique crystal shape or the extremely fine crystal size?Here, we investigated the surface oxidation behaviour of synthesised sub-micron pyrite with predominantly {100} or {111} facets. Our results showed that the specific crystal facet of the microscopic pyrite monocrystals, rather than the grain size, dominated its oxidation. Also, the shape controlled oxidation processes of pyrite as indicators of the local redox conditions of the palaeoenvironment are discussed.
top
Materials and Methods
Highly pure, specifically shaped microscopic pyrite samples were synthesised using a polymer assisted hydrothermal method, see Supplementary Information (SI). To induce oxidation reactions, the prepared pyrite films were exposed to humidity controlled air in airtight cells for 84 hr in total. During the oxidation process, the samples were removed for X-ray photoelectron spectroscopy (XPS) analysis at reaction intervals of 0, 6, 12, 24, 36, 60, and 84 hr. Details of the experimental procedures and characterisation methods are presented in the SI.
top
Results and Discussion
Structural, chemical and morphological analyses. No impurity reflections in the X-ray diffraction (XRD) patterns were identified, thereby confirming the high purity of the pyrite samples (Fig. 1a–c). In sample Py-tc (truncated cubic pyrite), the (111) reflection was relatively weak while the (200) reflection was relatively strong. Compared with those of sample Py-tc, the (111) reflections of samples Py-co (cubo-octahedral pyrite) and Py-to (truncated octahedral pyrite) were stronger, whereas the reflection of (200) was weaker.

Figure 1 (a–c) X-ray diffraction patterns of synthesised microscopic pyrite samples. (d–f) Field emission scanning electron microscope images of synthesised pyrite samples. (g–i) Energy dispersive spectrometry results of the pyrite samples. The half-quantitative data shown in the spectra were derived from the average measurements of five points. (j–l) Particle size distributions of the pyrite samples, which were derived from the statistical results of at least 500 grains from the micrographs.
Figure 1d–f presents the morphology of the three samples. The change in their morphology indicated that they possessed different proportions of cubic {100} and octahedral {111} facets, which was identical to the aforementioned XRD results. The energy dispersive spectrometry (EDS) results for all three samples, shown in Figure 1g–i, confirmed the chemical purity of the pyrite phases. It should be noted that there are weak signals in the range of 1-2 KeV, which may be due to the introduction of impurities such as Al and Si from the environment in the experimental processes, although their concentrations are less than 0.3 wt. %. EDS results also showed that the Py-co and Py-to samples possessed larger atomic ratios of S to Fe than the Py-tc sample. This was because the Py-co and Py-to samples possessed larger proportions of {111} facets, which are sulfur-rich surfaces (Alfonso, 2010
Alfonso, D.R. (2010) Computational Investigation of FeS2 Surfaces and Prediction of Effects of Sulfur Environment on Stabilities. Journal of Physical Chemistry C 114, 8971–8980.
), than the Py-tc sample.Statistical analysis based on field emission scanning electron microscope (FESEM) micrographs showed that the average grain sizes of the Py-tc, Py-co, and Py-to samples were 305.0, 641.5 and 801.4 nm, respectively (Fig. 1j–l). According to the geometric model (see SI), the SSAs were 2.54, 1.96 and 1.83 m2/g for the Py-tc, Py-co and Py-to samples, respectively.
Oxidation rate of microscopic pyrite with different morphologies. Figure 2 shows the S 2p and Fe 2p3/2 XPS spectra of pyrite before and after oxidation. Here, we used the relative content ratio of oxygen-containing and unoxidised species from the XPS spectra to characterise semi-quantitatively the oxidation rate of the microscopic pyrite (Zhu et al., 2018
Zhu, J., Xian, H., Lin, X., Tang, H., Du, R., Yang, Y., Zhu, R., Liang, X., Wei, J., Teng, H., He, H. (2018) Surface structure-dependent pyrite oxidation in relatively dry and moist air: Implications for the reaction mechanism and sulfur evolution. Geochimica et Cosmochimica Acta 228, 259–274.
). The oxygen-containing species from the S 2p XPS spectra included sulfate (SO42−), and the unoxidised species include bulk sulfur (S22−-bulk) and surface sulfur (S22−-surface). The oxygen-containing species from the Fe 2p XPS spectra are iron oxides (Fe-O), and the unoxidised species included bulk iron (Fe-bulk) and surface iron (Fe-surf.-I and Fe-surf.-II). The fitting details of S 2p and Fe 2p3/2 XPS spectra are given in the SI.
Figure 2 S 2p (a–f) and Fe 2p3/2 (g–l) X-ray photoelectron spectroscopy spectra of Py-tc (truncated cubic pyrite), Py-co (cubo-octahedral pyrite), and Py-to (truncated octahedral pyrite) surfaces oxidised in air with a relative humidity of 77 % at intervals of 0 hr and 84 hr. The Fe-surf.-I and Fe-surf.-II denote quadruplet and triplet surface states of iron on pyrite, respectively.
In addition, the XPS fitting results (Table S-1) showed that thiosulfate was only present at the initial stage of pyrite oxidation, thereby indicating that thiosulfate rapidly converts to sulfate. It is possible that some of these intermediates can be stabilised by impurities in natural pyrites, and thus modify oxidation rates in natural systems. The S/Fe ratio at different oxidation intervals (Table S-2) showed an increasing trend over oxidation time. Because the typical altered product of pyrite is an Fe-sulfate, the S/Fe ratio should be the same. Changes in this ratio may have been caused by concentration or structural changes, or even changes in the newly formed solid phases.
Figure 3a,b shows the kinetic results of the relative content ratios of oxygen-containing species to unoxidised species. The kinetic data from the S 2p and Fe 2p3/2 XPS spectra showed the order of the slope (k) for the three samples, i.e. kPy-to >kPy-co > kPy-tc. Because k is proportional to the oxidation rate of pyrite (Zhu et al., 2018
Zhu, J., Xian, H., Lin, X., Tang, H., Du, R., Yang, Y., Zhu, R., Liang, X., Wei, J., Teng, H., He, H. (2018) Surface structure-dependent pyrite oxidation in relatively dry and moist air: Implications for the reaction mechanism and sulfur evolution. Geochimica et Cosmochimica Acta 228, 259–274.
), the oxidation rates of the three pyrite samples were in the order rPy-to > rPy-co > rPy-tc (Table S-3). The normalised data (Fig. S-3) also showed the same rate order for the three samples.
Figure 3 Plots of the concentration ratios of oxidised species to unoxidised species (Coxidised/Cunoxidised) vs. oxidation time (t) from (a) S 2p and (b) Fe 2p3/2. X-ray photoelectron spectroscopy spectra are of Py-tc (truncated cubic pyrite), Py-co (cubo-octahedral pyrite), and Py-to (truncated octahedral pyrite). (c) The estimated oxidation rates of the samples are proportional to the ratio of microscopic pyrite {111} facets to {100} facets.
The order of the oxidation rate was opposite to that of the SSA [i.e. SSA(Py-tc) > SSA(Py-co) > SSA(Py-to)] for the three samples, thereby suggesting that the crystal morphology (i.e. surface structure), rather than their fine grain-induced large SSA, controls the reactivity. Therefore, the crystal morphology should be considered in the oxidation process of framboidal pyrite. The estimated oxidation rates of the samples were proportional to the ratio of microscopic pyrite {111} facets to {100} facets (Fig. 3c). Therefore, the oxidation rate of microscopic pyrite {111} facets is greater than that of {100} facets, which is caused by the difference in surface properties between the {100} and {111} facets (Zhu et al., 2018
Zhu, J., Xian, H., Lin, X., Tang, H., Du, R., Yang, Y., Zhu, R., Liang, X., Wei, J., Teng, H., He, H. (2018) Surface structure-dependent pyrite oxidation in relatively dry and moist air: Implications for the reaction mechanism and sulfur evolution. Geochimica et Cosmochimica Acta 228, 259–274.
; Xian et al., 2019Xian, H., He, H., Zhu, J., Du, R., Wu, X., Tang, H., Tan, W., Liang, X., Zhu, R., Teng, H. (2019) Crystal habit-directed gold deposition on pyrite: Surface chemical interpretation of the pyrite morphology indicative of gold enrichment. Geochimica et Cosmochimica Acta 264, 191–204.
). Regardless of grain size, the exposed crystal facet controls the reactivity of the framboidal pyrite microcrystals.Implications for indicating the local redox conditions of palaeoenvironments. The redox conditions of ancient sediments control the enrichment of biological and redox sensitive trace elements. Pyrite morphology and the size distribution of the framboids are used to indicate palaeoredox conditions (Wilkin and Barnes, 1997a
Wilkin, R.T., Barnes, H.L. (1997a) Pyrite formation in an anoxic estuarine basin. American Journal of Science 297, 620–650.
; Wignall et al., 2005Wignall, P.B., Newton, R., Brookfield, M.E. (2005) Pyrite framboid evidence for oxygen-poor deposition during the Permian–Triassic crisis in Kashmir. Palaeogeography, Palaeoclimatology, Palaeoecology 216, 183–188.
; Huang et al., 2016Huang, Y., Chen, Z.-Q., Wignall, P.B., Zhao, L. (2016) Latest Permian to Middle Triassic redox condition variations in ramp settings, South China: Pyrite framboid evidence. Geological Society of America Bulletin 129, 15.
; Liu et al., 2019Liu, Z., Chen, D., Zhang, J., Lü, X., Wang, Z., Liao, W., Shi, X., Tang, J., Xie, G. (2019) Pyrite Morphology as an Indicator of Paleoredox Conditions and Shale Gas Content of the Longmaxi and Wufeng Shales in the Middle Yangtze Area, South China. Minerals 9, 428.
). Such methods are applicable where there is no oxidative weathering. However, oxidative weathering is always present in ancient sediments. The oxidative products of pyrite, such as magnetite, hematite, limonite, magnesite and goethite, may be the result of pseudomorphism of pyrite (Luther et al., 1982Luther, G.W., Giblin, A., Howarth, R.W., Ryans, R.A. (1982) Pyrite and Oxidized Iron Mineral Phases Formed from Pyrite Oxidation in Salt-Marsh and Estuarine Sediments. Geochimica et Cosmochimica Acta 46, 2665–2669.
; Wilkin and Barnes, 1997bWilkin, R.T., Barnes, H.L. (1997b) Formation processes of framboidal pyrite. Geochimica et Cosmochimica Acta 61, 323–339.
). Incompletely oxidised pyrite will have a core-shell structure (Courtin-Nomade et al., 2010Courtin-Nomade, A., Bril, H., Bény, J.-M., Kunz, M., Tamura, N. (2010) Sulfide oxidation observed using micro-Raman spectroscopy and micro-X-ray diffraction: The importance of water/rock ratios and pH conditions. American Mineralogist 95, 582–591.
; Gu et al., 2020Gu, X., Heaney, P.J., Reis, F.D.A.A., Brantley, S.L. (2020) Deep abiotic weathering of pyrite. Science 370, doi: 10.1126/science.abb8092.
). Based on the results of this study, we propose that such a core-shell structure could indicate the relative redox conditions of the palaeoenvironment. This could be a potential proxy for interpreting local redox conditions, such as local oxidation before the Great Oxidation Event (Anbar et al., 2007Anbar, A.D., Duan, Y., Lyons, T.W., Arnold, G.L., Kendall, B., Creaser, R.A., Kaufman, A.J., Gordon, G.W., Scott, C., Garvin, J., Buick, R. (2007) A Whiff of Oxygen Before the Great Oxidation Event? Science 317, 1903–1906.
).According to the relationship between the shape of the microcrystalline particles and the oxidation rate (Fig. 3c), different crystal facets of oxidised pyrites may form oxidation species with different thicknesses (Fig. 4a). The relative redox conditions of palaeoenvironments where pyrites were present could therefore be characterised by a redox index p, as follows:

where r{hkl} is the oxidation rate of a specific pyrite facet {hkl} (obtained from Fig. 3c), i.e. r{100} = 3.63 × 10−9 [mol O2/m2 s] and r{111} = 10.79 × 10−9 [mol O2/m2 s], d{hkl} is the thickness of the oxidation shell along the [hkl] direction. It is worth noting that p is a facet-dependent variable. Without consideration of morphology, one may obtain false signals because of the intrinsic oxidation difference between pyrite facets. In practice, a smaller p{hkl} value indicates that the degree of pyrite oxidation is relatively weak, whereas a larger p{hkl} value denotes a stronger oxidation.
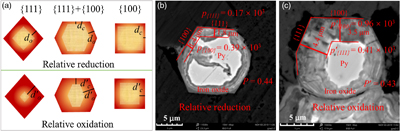
Figure 4 (a) Core-shell models of pyrite with different facets under two redox conditions. The red zone is the oxidised shell and the yellow zone is the unoxidised pyrite core. (b,c) Core-shell morphologies of oxidised natural pyrites from the Zhijin phosphorus deposit in Guizhou Province, China.
Figure 4b,c showed core-shell structures of two pyrites from a phosphorus deposit. The p and p’ are employed to represent the redox indexes of the two pyrites. The estimated redox indexes are in the order of p{111} < p′{111}, indicating that the latter experienced a more oxidising environment or longer duration of oxidation. Based on the aforementioned definition of the redox index, the p value of all [hkl] directions should be the same, e.g., p{100} = p{111}. However, the calculated p values show that p{100} ≠ p{111} and p′{100} ≠ p′{111}, which may be caused by the diffusion controlled reaction with the increase in the thickness of oxidation layers. Meanwhile, we introduce another index P (P = p{111}/p{100} ≤ 1). In the initial stage of pyrite oxidation, the reaction is fully controlled by surface structure, which gives P = 1. With the thickness increase of oxidation products, the reaction is diffusion controlled (the p{111} decreases first), resulting in P < 1. In addition, because d{100} < d{111}, there is a minimum for P, i.e. 1 > P > r{100}/r{111} = 0.34. Therefore, higher P (closer to 1) represent a shorter oxidation duration or lower oxygen fugacity, and thus weaker pyrite oxidation. The index P also works well for the pyrites shown in Figure 4b,c. Moreover, the presence of impurity elements tends to accelerate the oxidation of natural pyrite (Lehner and Savage, 2008
Lehner, S., Savage, K. (2008) The effect of As, Co, and Ni impurities on pyrite oxidation kinetics: Batch and flow-through reactor experiments with synthetic pyrite. Geochimica et Cosmochimica Acta 72, 1788–1800.
), but it will not affect the assessment of local redox conditions of palaeoenvironments if the presence of impurity elements has the same effect on the oxidation of a pyrite crystal in all crystallographic directions.top
Acknowledgements
This research was funded by the National Natural Science Foundation of China (Grant No. 41825003 and 41921003), Natural Science Foundation of Guangdong Province, China (Grant No. 2019A1515011303), China Postdoctoral Science Foundation (Grant Nos. 2018M643220 and 2019T120755), Guangdong Special Support Program (Grant No. 2019TX05L169), Tuguangchi Award for Excellent Young Scholar GIG, CAS, the Science and Technology Planning Project of Guangdong Province, China (Grant No. 2020B1212060055). This is contribution No. IS-2971 from GIG-CAS.
Editor: Satish Myneni
top
References
Alfonso, D.R. (2010) Computational Investigation of FeS2 Surfaces and Prediction of Effects of Sulfur Environment on Stabilities. Journal of Physical Chemistry C 114, 8971–8980.

This was because the Py-co and Py-to samples possessed larger proportions of {111} facets, which are sulfur-rich surfaces (Alfonso, 2010), than the Py-tc sample.
View in article
Anbar, A.D. (2008) Elements and Evolution. Science 322, 1481–1483.

Changes in redox conditions also affect the distribution of elements (Anbar, 2008).
View in article
Anbar, A.D., Duan, Y., Lyons, T.W., Arnold, G.L., Kendall, B., Creaser, R.A., Kaufman, A.J., Gordon, G.W., Scott, C., Garvin, J., Buick, R. (2007) A Whiff of Oxygen Before the Great Oxidation Event? Science 317, 1903–1906.

This could be a potential proxy for interpreting local redox conditions, such as local oxidation before the Great Oxidation Event (Anbar et al., 2007).
View in article
Courtin-Nomade, A., Bril, H., Bény, J.-M., Kunz, M., Tamura, N. (2010) Sulfide oxidation observed using micro-Raman spectroscopy and micro-X-ray diffraction: The importance of water/rock ratios and pH conditions. American Mineralogist 95, 582–591.

Incompletely oxidised pyrite will have a core-shell structure (Courtin-Nomade et al., 2010; Gu et al., 2020).
View in article
Fakhraee, M., Hancisse, O., Canfield, D.E., Crowe, S.A., Katsev, S. (2019) Proterozoic seawater sulfate scarcity and the evolution of ocean–atmosphere chemistry. Nature Geoscience 12, 375–380.

The formation and oxidative weathering of sedimentary pyrite are core processes in the sulfur cycle and are simultaneously involved in other major element cycles (such as carbon, nitrogen, and oxygen) (Hayakawa et al., 2013; Fike et al., 2015; Fakhraee et al., 2019).
View in article
Fike, D.A., Bradley, A.S., Rose, C.V. (2015) Rethinking the Ancient Sulfur Cycle. Annual Review of Earth and Planetary Sciences 43, 593–622.

The formation and oxidative weathering of sedimentary pyrite are core processes in the sulfur cycle and are simultaneously involved in other major element cycles (such as carbon, nitrogen, and oxygen) (Hayakawa et al., 2013; Fike et al., 2015; Fakhraee et al., 2019).
View in article
Gu, X., Heaney, P.J., Reis, F.D.A.A., Brantley, S.L. (2020) Deep abiotic weathering of pyrite. Science 370, doi: 10.1126/science.abb8092.

Incompletely oxidised pyrite will have a core-shell structure (Courtin-Nomade et al., 2010; Gu et al., 2020).
View in article
Guy, B.M., Beukes, N.J., Gutzmer, J. (2010) Paleoenvironmental controls on the texture and chemical composition of pyrite from non-conglomeratic sedimentary rocks of the Mesoarchean Witwatersrand Supergroup, South Africa. South African Journal of Geology 113, 195–228.

The oldest framboidal pyrite may be found in the late Archean (≤2.9 Ga) sediments. (Hallbauer, 1986; Guy et al., 2010).
View in article
Hallbauer, D.K. (1986) The mineralogy and geochemistry of Witwatersrand pyrite gold, uranium and carbonaceous matter mineral deposits of South Africa. In: Anhauesser, C.R., Maske, S. (Eds.) Mineral Deposits of Southern Africa. Geological Society of South Africa, South Africa, Vol. 1, 731–752.

The oldest framboidal pyrite may be found in the late Archean (≤2.9 Ga) sediments. (Hallbauer, 1986; Guy et al., 2010).
View in article
Hayakawa, A., Hatakeyama, M., Asano, R., Ishikawa, Y., Hidaka, S. (2013) Nitrate reduction coupled with pyrite oxidation in the surface sediments of a sulfide-rich ecosystem. Journal of Geophysical Research: Biogeosciences 118, 639–649.

The formation and oxidative weathering of sedimentary pyrite are core processes in the sulfur cycle and are simultaneously involved in other major element cycles (such as carbon, nitrogen, and oxygen) (Hayakawa et al., 2013; Fike et al., 2015; Fakhraee et al., 2019).
View in article
Huang, Y., Chen, Z.-Q., Wignall, P.B., Zhao, L. (2016) Latest Permian to Middle Triassic redox condition variations in ramp settings, South China: Pyrite framboid evidence. Geological Society of America Bulletin 129, 15.

Pyrite morphology and the size distribution of the framboids are used to indicate palaeoredox conditions (Wilkin and Barnes, 1997a; Wignall et al., 2005; Huang et al., 2016; Liu et al., 2019).
View in article
Kozina, N., Reykhard, L., Dara, O., Gordeev, V. (2018) Framboidal pyrite formation in the bottom sediments of the South Caspian Basin under conditions of hydrogen sulfide contamination. Russian Journal of Earth Sciences 18, 1–10.

Several studies have shown that framboidal pyrite grains are mostly euhedral microcrystals with specific shapes (mainly with {100} and {111} facets) (Ohfuji and Rickard, 2005; Rigby et al., 2006; Kozina et al., 2018).
View in article
Lehner, S., Savage, K. (2008) The effect of As, Co, and Ni impurities on pyrite oxidation kinetics: Batch and flow-through reactor experiments with synthetic pyrite. Geochimica et Cosmochimica Acta 72, 1788–1800.

Moreover, the presence of impurity elements tends to accelerate the oxidation of natural pyrite (Lehner and Savage, 2008).
View in article
Liu, Z., Chen, D., Zhang, J., Lü, X., Wang, Z., Liao, W., Shi, X., Tang, J., Xie, G. (2019) Pyrite Morphology as an Indicator of Paleoredox Conditions and Shale Gas Content of the Longmaxi and Wufeng Shales in the Middle Yangtze Area, South China. Minerals 9, 428.

Pyrite morphology and the size distribution of the framboids are used to indicate palaeoredox conditions (Wilkin and Barnes, 1997a; Wignall et al., 2005; Huang et al., 2016; Liu et al., 2019).
View in article
Luther, G.W., Giblin, A., Howarth, R.W., Ryans, R.A. (1982) Pyrite and Oxidized Iron Mineral Phases Formed from Pyrite Oxidation in Salt-Marsh and Estuarine Sediments. Geochimica et Cosmochimica Acta 46, 2665–2669.

The oxidative products of pyrite, such as magnetite, hematite, limonite, magnesite and goethite, may be the result of pseudomorphism of pyrite (Luther et al., 1982; Wilkin and Barnes, 1997b).
View in article
Ohfuji, H., Rickard, D. (2005) Experimental syntheses of framboids—a review. Earth-Science Reviews 71, 147–170.

Billions of pyrite grains are forming in sediments and waterways worldwide every second (Ohfuji and Rickard, 2005; Rickard, 2015, 2019a,b).
View in article
Several studies have shown that framboidal pyrite grains are mostly euhedral microcrystals with specific shapes (mainly with {100} and {111} facets) (Ohfuji and Rickard, 2005; Rigby et al., 2006; Kozina et al., 2018).
View in article
Pugh, C.E., Hossner, L.R., Dixon, J.B. (1984) Oxidation rate of iron sulfides as affected by surface area, morphology, oxygen concentration and autotrophic bacteria. Soil Science 137, 309–314.

The oxidation of framboidal pyrite is considered to be fast due to its high specific surface area (SSA) (Pugh et al., 1984; Weber et al., 2004), and the influence of the crystal shape is often neglected.
View in article
Rickard, D. (2012) Sulfidic Sediments and Sedimentary Rocks. Elsevier, Amsterdam, Netherlands.

Framboidal pyrite, which is the main component and dominant pyrite texture of sedimentary pyrite (Wilkin et al., 1996; Sawlowicz, 2000; Rickard, 2012), plays a major role in the oxidation of sedimentary pyrite.
View in article
Rickard, D. (2015) Pyrite: A Natural History of Fool’s Gold. Oxford University Press, New York.

Billions of pyrite grains are forming in sediments and waterways worldwide every second (Ohfuji and Rickard, 2005; Rickard, 2015, 2019a,b).
View in article
Rickard, D. (2019a) How long does it take a pyrite framboid to form? Earth and Planetary Science Letters 513, 64–68.

Billions of pyrite grains are forming in sediments and waterways worldwide every second (Ohfuji and Rickard, 2005; Rickard, 2015, 2019a,b).
View in article
Rickard, D. (2019b) Sedimentary pyrite framboid size-frequency distributions: A meta-analysis. Palaeogeography, Palaeoclimatology, Palaeoecology 522, 62–75.

Billions of pyrite grains are forming in sediments and waterways worldwide every second (Ohfuji and Rickard, 2005; Rickard, 2015, 2019a,b).
View in article
Rickard, D., Mussmann, M., Steadman, J.A. (2017) Sedimentary Sulfides. Elements 13, 117–122.

Sedimentary sulfides account for more than 95 % of the sulfides on the surface of the Earth and mainly occur as pyrite in sediments (Rickard et al., 2017).
View in article
Rigby, P.A., Dobos, S.K., Cook, F.J., Goonetilleke, A. (2006) Role of organic matter in framboidal pyrite oxidation. Science of The Total Environment 367, 847–854.

Several studies have shown that framboidal pyrite grains are mostly euhedral microcrystals with specific shapes (mainly with {100} and {111} facets) (Ohfuji and Rickard, 2005; Rigby et al., 2006; Kozina et al., 2018).
View in article
Sawlowicz, Z. (2000) Framboids: From their origin to application. Prace Mineralogiczne, Kraków, Poland.

Framboidal pyrite, which is the main component and dominant pyrite texture of sedimentary pyrite (Wilkin et al., 1996; Sawlowicz, 2000; Rickard, 2012), plays a major role in the oxidation of sedimentary pyrite.
View in article
Wang, P., Tian, J., Huang, E. (2018) Earth system and evolution. Science Press, Beijing.

Thus, these processes have regulated the redox conditions of the Earth’s surface through geological history, especially oceanic redox conditions (Wang et al., 2018).
View in article
Weber, P.A., Stewart, W.A., Skinner, W.M., Weisener, C.G., Thomas, J.E., Smart, R.St.C. (2004) Geochemical effects of oxidation products and framboidal pyrite oxidation in acid mine drainage prediction techniques. Applied Geochemistry 19, 1953–1974.

The oxidation of framboidal pyrite is considered to be fast due to its high specific surface area (SSA) (Pugh et al., 1984; Weber et al., 2004), and the influence of the crystal shape is often neglected.
View in article
Wignall, P.B., Newton, R., Brookfield, M.E. (2005) Pyrite framboid evidence for oxygen-poor deposition during the Permian–Triassic crisis in Kashmir. Palaeogeography, Palaeoclimatology, Palaeoecology 216, 183–188.

Pyrite morphology and the size distribution of the framboids are used to indicate palaeoredox conditions (Wilkin and Barnes, 1997a; Wignall et al., 2005; Huang et al., 2016; Liu et al., 2019).
View in article
Wilkin, R.T., Barnes, H.L. (1997a) Pyrite formation in an anoxic estuarine basin. American Journal of Science 297, 620–650.

Pyrite morphology and the size distribution of the framboids are used to indicate palaeoredox conditions (Wilkin and Barnes, 1997a; Wignall et al., 2005; Huang et al., 2016; Liu et al., 2019).
View in article
Wilkin, R.T., Barnes, H.L. (1997b) Formation processes of framboidal pyrite. Geochimica et Cosmochimica Acta 61, 323–339.

The oxidative products of pyrite, such as magnetite, hematite, limonite, magnesite and goethite, may be the result of pseudomorphism of pyrite (Luther et al., 1982; Wilkin and Barnes, 1997b).
View in article
Wilkin, R.T., Barnes, H.L., Brantley, S.L. (1996) The size distribution of framboidal pyrite in modern sediments: An indicator of redox conditions. Geochimica et Cosmochimica Acta 60, 3897–3912.

Framboidal pyrite, which is the main component and dominant pyrite texture of sedimentary pyrite (Wilkin et al., 1996; Sawlowicz, 2000; Rickard, 2012), plays a major role in the oxidation of sedimentary pyrite.
View in article
Xian, H., He, H., Zhu, J., Du, R., Wu, X., Tang, H., Tan, W., Liang, X., Zhu, R., Teng, H. (2019) Crystal habit-directed gold deposition on pyrite: Surface chemical interpretation of the pyrite morphology indicative of gold enrichment. Geochimica et Cosmochimica Acta 264, 191–204.

Therefore, the oxidation rate of microscopic pyrite {111} facets is greater than that of {100} facets, which is caused by the difference in surface properties between the {100} and {111} facets (Zhu et al., 2018; Xian et al., 2019).
View in article
Zhu, J., Xian, H., Lin, X., Tang, H., Du, R., Yang, Y., Zhu, R., Liang, X., Wei, J., Teng, H., He, H. (2018) Surface structure-dependent pyrite oxidation in relatively dry and moist air: Implications for the reaction mechanism and sulfur evolution. Geochimica et Cosmochimica Acta 228, 259–274.

Most previous studies mainly focused on powder samples, i.e. fracture surfaces, and ignored crystal facet effects (Zhu et al., 2018), which may have hindered the accurate determination of the pyrite oxidation mechanism.
View in article
Here, we used the relative content ratio of oxygen-containing and unoxidised species from the XPS spectra to characterise semi-quantitatively the oxidation rate of the microscopic pyrite (Zhu et al., 2018).
View in article
Because k is proportional to the oxidation rate of pyrite (Zhu et al., 2018), the oxidation rates of the three pyrite samples were in the order rPy-to > rPy-co > rPy-tc (Table S-3).
View in article
Therefore, the oxidation rate of microscopic pyrite {111} facets is greater than that of {100} facets, which is caused by the difference in surface properties between the {100} and {111} facets (Zhu et al., 2018; Xian et al., 2019).
View in article
top
Supplementary Information
The Supplementary Information includes:
- Materials and Methods
- Supplementary Text: Calculation of specific surface area; surface species on the microscopic pyrite
- Tables S-1 to S-3: S 2p peak parameters and chemical states of oxidised pyrites, S/Fe ratios derived from X-ray photoelectron spectroscopy (XPS) survey spectra at different oxidation intervals, and a comparison of oxidation rates of the samples.
- Figures S-1 to S-3: S 2p spectra and Fe 2p3/2 XPS spectra of oxidised Py-tc, Py-co and Py-to surfaces and the kinetic data normalised by the SSAs of the three samples.
- Supplementary Information References
Download the Supplementary Information (PDF).
Figures
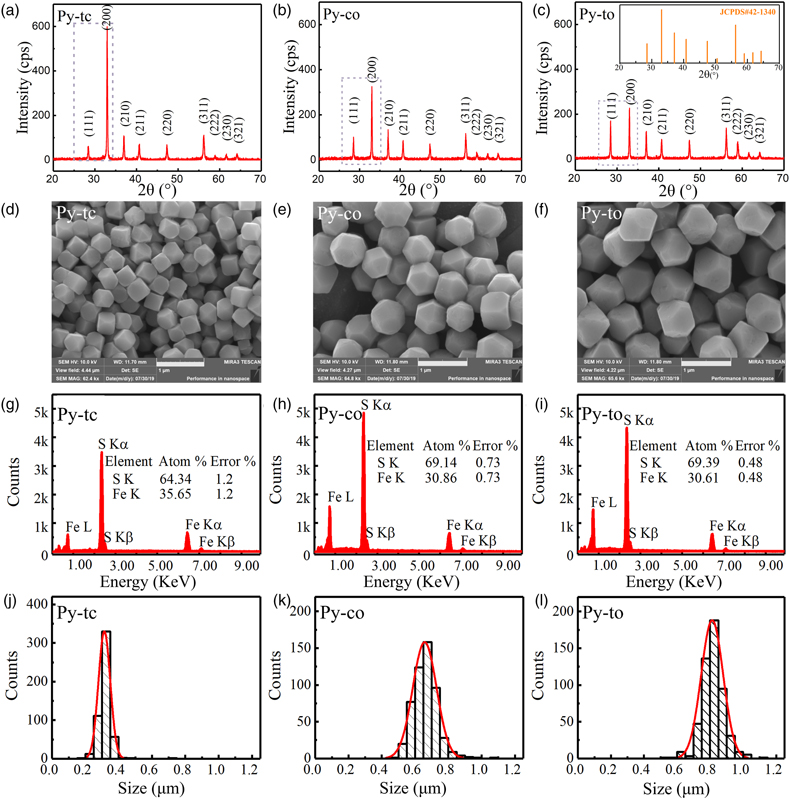
Figure 1 (a–c) X-ray diffraction patterns of synthesised microscopic pyrite samples. (d–f) Field emission scanning electron microscope images of synthesised pyrite samples. (g–i) Energy dispersive spectrometry results of the pyrite samples. The half-quantitative data shown in the spectra were derived from the average measurements of five points. (j–l) Particle size distributions of the pyrite samples, which were derived from the statistical results of at least 500 grains from the micrographs.
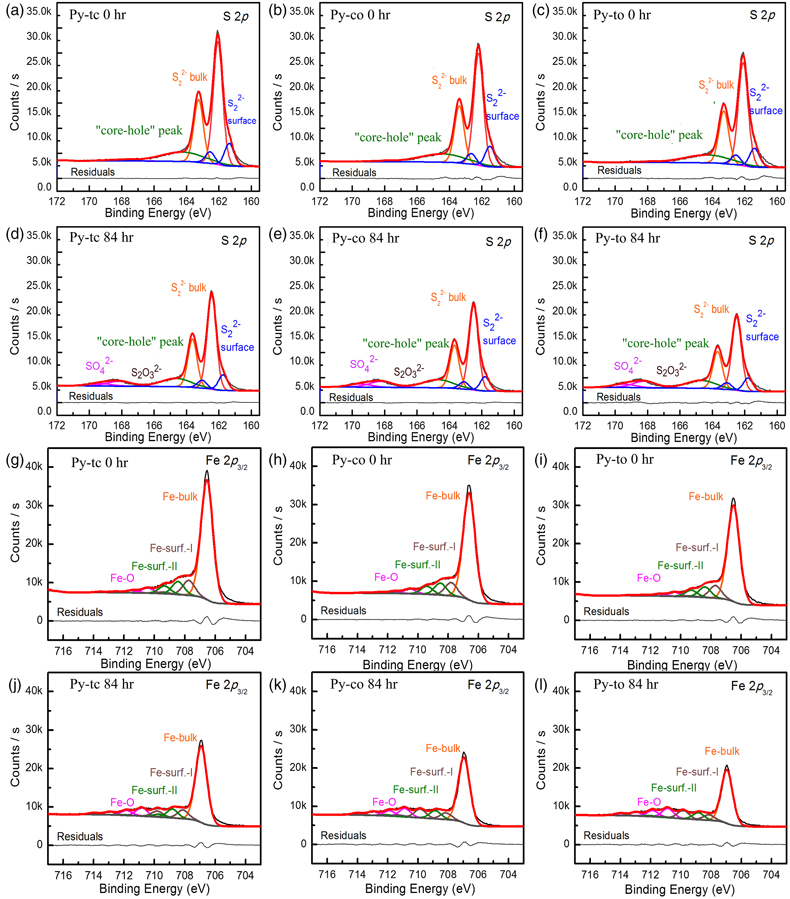
Figure 2 S 2p (a–f) and Fe 2p3/2 (g–l) X-ray photoelectron spectroscopy spectra of Py-tc (truncated cubic pyrite), Py-co (cubo-octahedral pyrite), and Py-to (truncated octahedral pyrite) surfaces oxidised in air with a relative humidity of 77 % at intervals of 0 hr and 84 hr. The Fe-surf.-I and Fe-surf.-II denote quadruplet and triplet surface states of iron on pyrite, respectively.

Figure 3 Plots of the concentration ratios of oxidised species to unoxidised species (Coxidised/Cunoxidised) vs. oxidation time (t) from (a) S 2p and (b) Fe 2p3/2. X-ray photoelectron spectroscopy spectra are of Py-tc (truncated cubic pyrite), Py-co (cubo-octahedral pyrite), and Py-to (truncated octahedral pyrite). (c) The estimated oxidation rates of the samples are proportional to the ratio of microscopic pyrite {111} facets to {100} facets.

Figure 4 (a) Core-shell models of pyrite with different facets under two redox conditions. The red zone is the oxidised shell and the yellow zone is the unoxidised pyrite core. (b,c) Core-shell morphologies of oxidised natural pyrites from the Zhijin phosphorus deposit in Guizhou Province, China.