The pressure-induced local structural change around tungsten in silicate glass
Affiliations | Corresponding Author | Cite as | Funding information- Share this article
-
Article views:190Cumulative count of HTML views and PDF downloads.
- Download Citation
- Rights & Permissions
top
Abstract
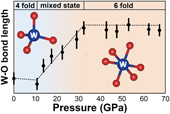
Figures and Tables
![]() Figure 1 EXAFS oscillations of tungsten in a basaltic glass and FT-EXAFS spectra up to 67 GPa. (a) The k3-weighted oscillations, χ(k) × k3, extracted from EXAFS spectra (black curves). The vertical dotted lines highlight the wave numbers k of the first and second maxima of the oscillations at ambient pressure. (b) The radial structural function at the LIII-edge for tungsten, which was Fourier transformed (FT) from k3-weighted EXAFS oscillations (black curves). The vertical dotted line indicates the position of the maximum FT-magnitude at 1 bar. The red curves in (a) and (b) show simulation data of EXAFS spectra using the parameter extracted by FEFF. | ![]() Figure 2 Pressure-induced change in the W-O bond length (open circles) in basaltic glass on compression from the present EXAFS analyses, compared to those in the Si-O bond length. Filled squares (Prescher et al., 2017) and diamonds (Sato and Funamori, 2010) indicate data on SiO2 glass. Open squares are for a molten basalt (Sanloup et al., 2013). All the Si-O bond length data were obtained by XRD measurements. | ![]() Figure 3 Tungsten LIII-edge XANES spectra of a basaltic glass collected up to 67 GPa after background subtraction. (a) Normalised XANES spectra and (b) their second derivatives. In (b), the blue and red dashed lines indicate the position of the minimum of the second derivatives of the XANES spectrum at 1 bar and that of the local minimum that is prominent above 32.3 GPa, respectively. These XANES spectra show a clear difference between fourfold and sixfold coordinated W6+. | ![]() Table 1 The W-O bond length in a basaltic glass at each pressure obtained from the k range of EXAFS spectra provided. Standard deviations are given in parentheses. |
Figure 1 | Figure 2 | Figure 3 | Table 1 |
top
Introduction
The Earth’s mantle is depleted in siderophile (iron-loving) elements because they were preferentially partitioned into metals during core formation. Such depletion can be a record of conditions for core-forming metal segregation from silicate. Among siderophile elements, the metal-silicate partitioning of tungsten has been extensively studied because of its chemical properties and geological significance. Since tungsten is a highly refractory element, its bulk Earth abundance is obtained from the chondritic abundance without correction for volatility (see review by McDonough, 2014
McDonough, W.F. (2014) Compositional model for the Earth’s core. In: Holland, H.D., Turekian, K.K. (Eds.) Treatise on Geochemistry. Second Edition, Elsevier, Oxford, 559–577.
), giving the tungsten content in the core from the known mantle abundance. The distribution of tungsten between the core and the mantle is thought to be key to understanding the conditions for metal-silicate chemical equilibrium during core metal segregation.Previous experiments have explored the effects of pressure, temperature, oxygen fugacity, and the compositions of metallic liquid and silicate melt on the metal-silicate partitioning of tungsten (Walter and Thibault, 1995
Walter, M.J., Thibault, Y. (1995) Partitioning of tungsten and molybdenum between metallic liquid and silicate melt. Science 270, 1186–1189.
; O’Neill et al., 2008O’Neill, H.S.C., Berry, A.J., Eggins, S.M. (2008) The solubility and oxidation state of tungsten in silicate melts: Implications for the comparative chemistry of W and Mo in planetary differentiation processes. Chemical Geology 255, 346–359.
; Cottrell et al., 2009Cottrell, E., Walter, M.J., Walker, D. (2009) Metal–silicate partitioning of tungsten at high pressure and temperature: Implications for equilibrium core formation in Earth. Earth and Planetary Science Letters 281, 275–287.
; Siebert et al., 2011Siebert, J., Corgne, A., Ryerson, F.J. (2011) Systematics of metal-silicate partitioning for many siderophile elements applied to Earth’s core formation. Geochimica et Cosmochimica Acta 75, 1451–1489.
; Rai and van Westrenen, 2014Rai, N., van Westrenen, W. (2014) Lunar core formation: New constraints from metal–silicate partitioning of siderophile elements. Earth and Planetary Science Letters 388, 343–352.
; Jennings et al., 2020Jennings, E.S., Jacobson, S.A., Rubie, D.C., Nakajima, Y., Vogel, A.K., Rose-Weston, L.A., Frost, D.J. (2020) Metal–silicate partitioning of W and Mo and the role of carbon in controlling their abundances in the bulk silicate earth. Geochimica et Cosmochimica Acta 293, 40–69.
). However, partitioning is still not well understood even at relatively low pressures. It has been argued that tungsten becomes more siderophile with increasing pressure to ∼5 GPa and then less siderophile at higher pressures (Cottrell et al., 2009Cottrell, E., Walter, M.J., Walker, D. (2009) Metal–silicate partitioning of tungsten at high pressure and temperature: Implications for equilibrium core formation in Earth. Earth and Planetary Science Letters 281, 275–287.
; Rai and van Westrenen, 2014Rai, N., van Westrenen, W. (2014) Lunar core formation: New constraints from metal–silicate partitioning of siderophile elements. Earth and Planetary Science Letters 388, 343–352.
). This was attributed to the formation of W4+ (Cottrell et al., 2009Cottrell, E., Walter, M.J., Walker, D. (2009) Metal–silicate partitioning of tungsten at high pressure and temperature: Implications for equilibrium core formation in Earth. Earth and Planetary Science Letters 281, 275–287.
) due to the emergence of octahedrally coordinated silicon in silicate melts (Sanloup et al., 2013Sanloup, C., Drewitt, J.W.E., Konopkova, Z., Dalladay-Simpson, P., Morton, D.M., Rai, N., van Westrenen, W., Morgenroth, W. (2013) Structural change in molten basalt at deep mantle conditions. Nature 503, 104–107.
). Sanloup et al. (2011)Sanloup, C., van Westrenen, W., Dasgupta, R., Maynard-Casely, H., Perrillat, J. (2011) Compressibility change in iron-rich melt and implications for core formation models. Earth and Planetary Science Letters 306, 118–122.
also pointed out that a change in the compressibility of liquid iron may affect the metal-silicate partitioning of trace elements including tungsten.Pressure-induced structural changes in silicate melt and glasses have been examined, focusing on a change in the coordination of silicon (e.g., Sanloup et al., 2013
Sanloup, C., Drewitt, J.W.E., Konopkova, Z., Dalladay-Simpson, P., Morton, D.M., Rai, N., van Westrenen, W., Morgenroth, W. (2013) Structural change in molten basalt at deep mantle conditions. Nature 503, 104–107.
; Prescher et al., 2017Prescher, C., Prakapenka, V.B., Stefanski, J., Jahn, S., Skinner, L.B., Wang, Y. (2017) Beyond sixfold coordinated Si in SiO2 glass at ultrahigh pressures. Proceedings of the National Academy of Sciences 114, 10041–10046.
). The local structure around a trace element in silicate melt may be important for its partitioning, in particular for cations with high valences such as W6+, but it has been least explored. Keppler and Rubie (1993)Keppler, H., Rubie, D.C. (1993) Pressure-induced coordination changes of transition-metal ions in silicate melts. Science 364, 54–56.
reported the coordination changes of Ni2+ and Co2+ in silicate melts at high pressures up to 10 GPa based on crystal field spectroscopy on quenched glasses at ambient conditions. The coordination environments of lutetium and xenon in silicate glasses and melts were examined by using in situ high pressure X-ray diffraction (XRD) techniques to 8 GPa (de Grouchy et al., 2017de Grouchy, C.J.L., Sanloup, C., Cochain, B., Drewitt, J.W.E., Kono, Y., Crépisson, C. (2017) Lutetium incorporation in magmas at depth: Changes in melt local environment and the influence on partitioning behaviour. Earth and Planetary Science Letters 464, 155–165.
; Leroy et al., 2018Leroy, C., Sanloup, C., Bureau, H., Schmidt, B.C., Konopkova, Z., Raepsaet, C. (2018) Bonding of xenon to oxygen in magmas at depth. Earth and Planetary Science Letters 484, 103–110.
). As far as we know, there are no experimental studies that investigate the local structure around trace elements under lower mantle conditions so far, because of their low concentrations and disturbance by Compton signals from diamond in XRD measurements at high pressure in a diamond-anvil cell (DAC).Here we examine a change in the coordination structure of tungsten in a basaltic glass with increasing pressure to 67 GPa, based on in situ high pressure X-ray absorption spectroscopy in the fluorescence yield mode, which gives element selective information and is sensitive to trace elements. The extended X-ray absorption fine structure (EXAFS) and X-ray absorption near-edge structure (XANES) spectra demonstrate the pressure-induced coordination change of W6+. We discuss its implications for the partitioning behaviour of tungsten at high pressure.
top
Results and Discussion
Change in the coordination structure of tungsten. We obtained twelve separate tungsten LIII-edge EXAFS spectra of the W-doped basaltic silicate glass in a wide pressure range from 1 bar to 67.2 GPa, whose k3-weighted oscillations are shown in Figure 1a. As can be seen from the change in the oscillation period, the average W-O bond became longer with increasing pressure from 10.7 to 32.3 GPa although it did not change outside of this pressure range (Fig. 2). The W-O bond length (rW-O) was determined at each pressure from the EXAFS analysis (Fig. 1, Table 1).
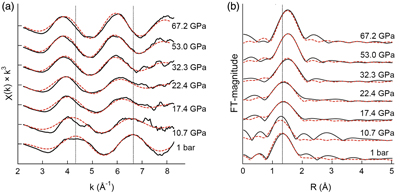
Figure 1 EXAFS oscillations of tungsten in a basaltic glass and FT-EXAFS spectra up to 67 GPa. (a) The k3-weighted oscillations, χ(k) × k3, extracted from EXAFS spectra (black curves). The vertical dotted lines highlight the wave numbers k of the first and second maxima of the oscillations at ambient pressure. (b) The radial structural function at the LIII-edge for tungsten, which was Fourier transformed (FT) from k3-weighted EXAFS oscillations (black curves). The vertical dotted line indicates the position of the maximum FT-magnitude at 1 bar. The red curves in (a) and (b) show simulation data of EXAFS spectra using the parameter extracted by FEFF.

Figure 2 Pressure-induced change in the W-O bond length (open circles) in basaltic glass on compression from the present EXAFS analyses, compared to those in the Si-O bond length. Filled squares (Prescher et al., 2017
Prescher, C., Prakapenka, V.B., Stefanski, J., Jahn, S., Skinner, L.B., Wang, Y. (2017) Beyond sixfold coordinated Si in SiO2 glass at ultrahigh pressures. Proceedings of the National Academy of Sciences 114, 10041–10046.
) and diamonds (Sato and Funamori, 2010Sato, T., Funamori, N. (2010) High-pressure structural transformation of SiO2 glass up to 100 GPa. Physical Review B 82, 184102.
) indicate data on SiO2 glass. Open squares are for a molten basalt (Sanloup et al., 2013Sanloup, C., Drewitt, J.W.E., Konopkova, Z., Dalladay-Simpson, P., Morton, D.M., Rai, N., van Westrenen, W., Morgenroth, W. (2013) Structural change in molten basalt at deep mantle conditions. Nature 503, 104–107.
). All the Si-O bond length data were obtained by XRD measurements.Table 1 The W-O bond length in a basaltic glass at each pressure obtained from the k range of EXAFS spectra provided. Standard deviations are given in parentheses.
Pressure (GPa) | k range (Å−1) | W-O bond length (Å) |
0 | 2.3–8.2 | 1.771(18) |
10.7 | 2.3–8.2 | 1.753(19) |
13.7 | 2.3–8.2 | 1.814(21) |
17.4 | 2.3–8.2 | 1.845(23) |
22.4 | 2.3–8.2 | 1.858(23) |
29.0 | 2.3–7.0 | 1.900(29) |
32.3 | 2.3–8.2 | 1.939(21) |
42.7 | 2.3–7.0 | 1.932(28) |
47.5 | 2.3–8.2 | 1.928(21) |
53.0 | 2.3–8.2 | 1.950(20) |
62.3 | 2.3–8.2 | 1.934(19) |
67.2 | 2.3–8.2 | 1.931(19) |
At ambient pressure, we obtained rW-O = 1.77 ± 0.02 Å in the basaltic glass, similar to rW-O = 1.78 Å in WO42− solution reported by Kashiwabara et al. (2013)
Kashiwabara, T., Takahashi, Y., Marcus, M.A., Uruga, T., Tanida, H., Terada, Y., Usui, A. (2013) Tungsten species in natural ferromanganese oxides related to its different behavior from molybdenum in oxic ocean. Geochimica et Cosmochimica Acta 106, 364–378.
. This indicates that W6+ was predominantly tetrahedrally coordinated at 1 bar in our glass sample in agreement with O’Neill et al. (2008)O’Neill, H.S.C., Berry, A.J., Eggins, S.M. (2008) The solubility and oxidation state of tungsten in silicate melts: Implications for the comparative chemistry of W and Mo in planetary differentiation processes. Chemical Geology 255, 346–359.
. At 10.7 GPa, we found rW-O = 1.75 ± 0.02 Å, which is close to the average W-O bond length at ambient pressure. Nevertheless, the XANES spectra and their second derivatives, which are sensitive to the local structure around an element of interest, show a difference between the two pressures (Fig. 3), possibly suggesting that the WO4 tetrahedral symmetry was slightly changed by compression.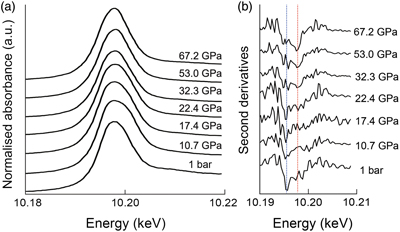
Figure 3 Tungsten LIII-edge XANES spectra of a basaltic glass collected up to 67 GPa after background subtraction. (a) Normalised XANES spectra and (b) their second derivatives. In (b), the blue and red dashed lines indicate the position of the minimum of the second derivatives of the XANES spectrum at 1 bar and that of the local minimum that is prominent above 32.3 GPa, respectively. These XANES spectra show a clear difference between fourfold and sixfold coordinated W6+.
A rapid increase in the average W-O bond length in a pressure range from 10.7 to 32.3 GPa can be attributed to a change in the mean coordination number from four to six (Fig. 2). rW-O = 1.94 Å at 32.3 GPa is longer by 0.17 Å than rW-O = 1.77 Å at 1 bar. This difference in the average W-O bond length is similar to the difference in the ionic radii between 0.42 and 0.60 Å for fourfold and sixfold coordinated W6+, respectively (Shannon, 1976
Shannon, R. (1976) Revised effective ionic radii and systematic studies of interatomic distances in halides and chalcogenides. Acta Crystallographica Section A 32, 751–767.
). Above 32.3 GPa, the average W-O bond length remained similar; neither the period of EXAFS oscillations nor the XANES spectra changed significantly (Figs. 1, 3). This could be because the effect of compression was compensated by the effect of the small continuous increase in the mean coordination number of W6+ to >6.Coordination changes of tungsten and silicon at similar pressure range. These changes in the average W-O bond length and the tungsten coordination number take place at a pressure range very similar to that in which silicon changes its coordination from four to six in silicate glass and melt (see a comparison in Fig. 2). Previous XRD measurements have examined the pressure effect on the average Si-O bond length and the coordination number of silicon in SiO2 glass up to a megabar pressure (Prescher et al., 2017
Prescher, C., Prakapenka, V.B., Stefanski, J., Jahn, S., Skinner, L.B., Wang, Y. (2017) Beyond sixfold coordinated Si in SiO2 glass at ultrahigh pressures. Proceedings of the National Academy of Sciences 114, 10041–10046.
; Sato and Funamori, 2010Sato, T., Funamori, N. (2010) High-pressure structural transformation of SiO2 glass up to 100 GPa. Physical Review B 82, 184102.
). The most recent experiments performed by Prescher et al. (2017)Prescher, C., Prakapenka, V.B., Stefanski, J., Jahn, S., Skinner, L.B., Wang, Y. (2017) Beyond sixfold coordinated Si in SiO2 glass at ultrahigh pressures. Proceedings of the National Academy of Sciences 114, 10041–10046.
demonstrated that i) below 10 GPa, the Si-O bond length was constant at ∼1.62 Å, while volume was reduced because of the collapse of void space (topological change), ii) between 10 and 40 GPa, the Si-O bond length increased to ∼1.69 Å as a consequence of the increase in the mean silicon coordination number from four to six, and iii) above 40 GPa, the Si-O bond length slightly decreased with pressure, as illustrated in Figure 2. Furthermore, the XRD study on a basaltic melt by Sanloup et al. (2013)Sanloup, C., Drewitt, J.W.E., Konopkova, Z., Dalladay-Simpson, P., Morton, D.M., Rai, N., van Westrenen, W., Morgenroth, W. (2013) Structural change in molten basalt at deep mantle conditions. Nature 503, 104–107.
showed that the Si-O bond length rapidly increased from <10 to ∼35 GPa (Fig. 2), consistent with the observations in SiO2 glass. The ab initio simulations of a model basalt by Bajgain et al. (2015)Bajgain, S., Ghosh, D.B., Karki, B.B. (2015) Structure and density of basaltic melts at mantle conditions from first-principles simulations. Nature Communications 6, 8578.
reported that the mean silicon coordination number started to increase from 5.3 GPa at 2,200 K. They additionally calculated the mean coordination numbers of other major cations at high pressures, demonstrating that those of network modifier cations of Na+, Ca2+, Mg2+, and Fe2+ increase with initial compression to 20 GPa, in contrast to those of network former cations of Si4+ and Al3+.These suggest that the coordination increase in tungsten may be induced by that of silicon which determines the overall networking structure in silicate. Below 10 GPa, W6+ is predominantly fourfold coordinated and therefore cannot be a network former in a silicate glass (Farges et al., 2006
Farges, F., Linnen, R.L., Brown, G.E. Jr. (2006) Redox and speciation of tin in hydrous silicate glasses: A comparison with Nb, Ta, Mo and W. The Canadian Mineralogist 44, 775.
). This is explained by the bond valence theory; oxygen cannot connect fourfold coordinated W6+ and Si4+ ions because the sum of the valence units combining W6+-O (VI/4 = 1.5) and Si4+-O bonds (IV/4 = 1.0) exceeds the valence (= 2) of an oxygen ion (Pauling, 1929Pauling, L. (1929) The principles determining the structure of complex ionic crystals. Journal of American Chemical Society 51, 1010–1026.
). Therefore, the WO4 tetrahedra are positioned in a region that is rich in the network modifier cations (e.g., Na+, Ca2+, Mg2+, and Fe2+), and are disconnected from the framework of SiO2 tetrahedra. This could be the reason why tungsten is much less siderophile during metal-silicate partitioning when silicate melt exhibits higher NBO/T (the ratio of non-bridging oxygens per tetrahedrally coordinated cations, a measure of the depolymerisation) (Walter and Thibault, 1995Walter, M.J., Thibault, Y. (1995) Partitioning of tungsten and molybdenum between metallic liquid and silicate melt. Science 270, 1186–1189.
) or contains more alkali metals and alkaline earth metals, in particular CaO (Jennings et al., 2020Jennings, E.S., Jacobson, S.A., Rubie, D.C., Nakajima, Y., Vogel, A.K., Rose-Weston, L.A., Frost, D.J. (2020) Metal–silicate partitioning of W and Mo and the role of carbon in controlling their abundances in the bulk silicate earth. Geochimica et Cosmochimica Acta 293, 40–69.
). Above 10 GPa, fourfold coordinated Si4+ starts to change into sixfold coordination, leading to an increase in bridging oxygen at the expense of non-bridging oxygen and possibly the oxygen constituting WO4 tetrahedra. While fourfold coordinated W6+ cannot bind to the bridging oxygen due to its high bond valence, it is possible for sixfold coordinated W6+ to do so because its bond valence units decrease to 1.0 (= VI/6). Such sixfold coordinated W6+ can thus be a network former cation, bonding to the bridging oxygen together with the charge compensating cations such as Na+ in a basaltic glass.Implications for the partitioning of tungsten. The change in the nature of the W6+-O bond will affect the partitioning behaviour of tungsten. Cottrell et al. (2009)
Cottrell, E., Walter, M.J., Walker, D. (2009) Metal–silicate partitioning of tungsten at high pressure and temperature: Implications for equilibrium core formation in Earth. Earth and Planetary Science Letters 281, 275–287.
examined the partitioning of tungsten between liquid iron and basaltic melt and showed positive and negative pressure effects on the metal/silicate partition coefficient below and above 2–6 GPa, respectively. A more recent study by Rai and van Westrenen (2014)Rai, N., van Westrenen, W. (2014) Lunar core formation: New constraints from metal–silicate partitioning of siderophile elements. Earth and Planetary Science Letters 388, 343–352.
also demonstrated the positive pressure effect below 5 GPa before it changes to negative at higher pressures. Cottrell et al. (2009)Cottrell, E., Walter, M.J., Walker, D. (2009) Metal–silicate partitioning of tungsten at high pressure and temperature: Implications for equilibrium core formation in Earth. Earth and Planetary Science Letters 281, 275–287.
argued that such a change in the pressure effect may correspond to the emergence of W4+, but this was not supported by Wade et al. (2012)Wade, J., Wood, B.J., Tuff, J. (2012) Metal–silicate partitioning of Mo and W at high pressures and temperatures: Evidence for late accretion of sulphur to the Earth. Geochimica et Cosmochimica Acta 85, 58–74.
who found that the valence of tungsten remained six in silicate melt at 6 to 24 GPa based on augmented experimental data. This might be attributed to the change in the structure of liquid iron alloy (Sanloup et al., 2011Sanloup, C., van Westrenen, W., Dasgupta, R., Maynard-Casely, H., Perrillat, J. (2011) Compressibility change in iron-rich melt and implications for core formation models. Earth and Planetary Science Letters 306, 118–122.
; Shibazaki et al., 2015Shibazaki, Y., Kono, Y., Fei, Y. (2015) Microscopic structural change in a liquid Fe-C alloy of ∼5 GPa. Geophysical Research Letters 42, 13.
), but the pressure response of the metal-silicate partitioning of tungsten is different from that of nickel and cobalt, which cannot be explained solely by the structural change in liquid metal. Alternatively, it is likely that the change in the pressure effect on the metal/silicate partitioning of tungsten above ∼5 GPa is caused by the onset of the increase in the coordination number of W6+ in a silicate melt (note that this may start in melt at pressures slightly lower than that in glass as predicted for Si by Bajgain et al., 2015Bajgain, S., Ghosh, D.B., Karki, B.B. (2015) Structure and density of basaltic melts at mantle conditions from first-principles simulations. Nature Communications 6, 8578.
). These observations further suggest that the pressure effect on the metal/silicate partitioning of tungsten above 32 GPa (sixfold coordinated W6+ predominant) is likely different from that at <5 GPa (fourfold coordinated W6+ predominant) and 5–32 GPa (mixed state).It has also been argued that the metal-silicate partitioning of tungsten strongly depends on the silicate melt composition represented by such as NBO/T or the CaO content as mentioned above (e.g., Walter and Thibault, 1995
Walter, M.J., Thibault, Y. (1995) Partitioning of tungsten and molybdenum between metallic liquid and silicate melt. Science 270, 1186–1189.
; Jennings et al., 2020Jennings, E.S., Jacobson, S.A., Rubie, D.C., Nakajima, Y., Vogel, A.K., Rose-Weston, L.A., Frost, D.J. (2020) Metal–silicate partitioning of W and Mo and the role of carbon in controlling their abundances in the bulk silicate earth. Geochimica et Cosmochimica Acta 293, 40–69.
). For example, according to the parameterisation by O’Neill et al. (2008)O’Neill, H.S.C., Berry, A.J., Eggins, S.M. (2008) The solubility and oxidation state of tungsten in silicate melts: Implications for the comparative chemistry of W and Mo in planetary differentiation processes. Chemical Geology 255, 346–359.
, the partition coefficient of tungsten between metal and pyrolitic melt is forty times as high as that between metal and basaltic melt below 6 GPa. Such strong compositional dependence may significantly diminish as the coordination structure of tungsten changes. Indeed, the earlier experiments conducted by Siebert et al. (2011)Siebert, J., Corgne, A., Ryerson, F.J. (2011) Systematics of metal-silicate partitioning for many siderophile elements applied to Earth’s core formation. Geochimica et Cosmochimica Acta 75, 1451–1489.
have demonstrated that the effect of NBO/T on metal-silicate partitioning is smaller for sixfold coordinated, high valence cations such as Nb5+ and Ta5+ than for fourfold coordinated W6+ and P5+. We can thus expect that the effect of silicate melt composition on the metal-silicate partitioning of tungsten is smaller above 32 GPa, where sixfold coordinated W6+ is predominant, than previously found below 10 GPa.Moreover, the change in the coordination number of tungsten should affect its isotopic fractionation between melt and crystals at high pressure. In general, heavier isotopes tend to be partitioned into a phase with a lower coordination number (Kashiwabara et al., 2017
Kashiwabara, T., Kubo, S., Tanaka, M., Senda, R., Iizuka, T., Tanimizu, M., Takahashi, Y. (2017) Stable isotope fractionation of tungsten during adsorption on Fe and Mn (oxyhydr)oxides. Geochimica et Cosmochimica Acta 204, 52–67.
). Therefore, the 182W/184W ratio in silicate melt equilibrated with metallic liquid above 32 GPa may be higher than that below 10 GPa, although the temperature for metal-silicate equilibrium at the time of core formation may have been too high to cause a resolvable isotopic fractionation, as is the case for Mo as reported by Hin et al. (2019)Hin, R.C., Burnham, A.D., Gianolio, D., Walter, M.J., Elliot, T. (2019) Molybdenum isotope fractionation between Mo4+ and Mo6+ in silicate liquid and metallic Mo. Chemical Geology 504, 177–189.
.Coordination changes of other trace elements. It is worth emphasising that the pressure range for the coordination change of W6+ observed in this study is quite different from those for Ni2+, Co2+ (Keppler and Rubie, 1993
Keppler, H., Rubie, D.C. (1993) Pressure-induced coordination changes of transition-metal ions in silicate melts. Science 364, 54–56.
), and Lu3+ (de Grouchy et al., 2017de Grouchy, C.J.L., Sanloup, C., Cochain, B., Drewitt, J.W.E., Kono, Y., Crépisson, C. (2017) Lutetium incorporation in magmas at depth: Changes in melt local environment and the influence on partitioning behaviour. Earth and Planetary Science Letters 464, 155–165.
), whose coordination numbers increase rapidly with increasing pressure up to 10 GPa. Such a difference may be related to the field strength (= charge/ionic radius) of these trace elements. The low field strength of Ni2+, Co2+, and Lu3+ suggests that their local structures are similar to those of network modifier cations. On the other hand, we expect that the local structures around high field strength elements such as Mo6+, P5+, and As5+ are close to that of W6+, and therefore their coordination numbers will increase in a manner similar to that of tungsten. Future studies of these high field strength elements besides tungsten will clarify the importance of the field strength on the behaviour of pressure-induced coordination changes in silicate glasses and melts.top
Acknowledgements
We thank H. Sakuma for discussion on the cause of pressure-induced coordination change of tungsten. K. Ohta is acknowledged for his help in DAC experiments. Comments from two anonymous reviewers helped to improve the manuscript. This work was supported by JSPS Kakenhi grants no. 16H06285 and 20J21667. This work was performed with the approval of Photon Factory (proposal no. 2018S1-001 and 2020G670).
Editor: Anat Shahar
top
References
Bajgain, S., Ghosh, D.B., Karki, B.B. (2015) Structure and density of basaltic melts at mantle conditions from first-principles simulations. Nature Communications 6, 8578.

The ab initio simulations of a model basalt by Bajgain et al. (2015) reported that the mean silicon coordination number started to increase from 5.3 GPa at 2,200 K. They additionally calculated the mean coordination numbers of other major cations at high pressures, demonstrating that those of network modifier cations of Na+, Ca2+, Mg2+, and Fe2+ increase with initial compression to 20 GPa, in contrast to those of network former cations of Si4+ and Al3+.
View in article
Alternatively, it is likely that the change in the pressure effect on the metal/silicate partitioning of tungsten above ∼5 GPa is caused by the onset of the increase in the coordination number of W6+ in a silicate melt (note that this may start in melt at pressures slightly lower than that in glass as predicted for Si by Bajgain et al., 2015).
View in article
Cottrell, E., Walter, M.J., Walker, D. (2009) Metal–silicate partitioning of tungsten at high pressure and temperature: Implications for equilibrium core formation in Earth. Earth and Planetary Science Letters 281, 275–287.

Previous experiments have explored the effects of pressure, temperature, oxygen fugacity, and the compositions of metallic liquid and silicate melt on the metal-silicate partitioning of tungsten (Walter and Thibault, 1995; O’Neill et al., 2008; Cottrell et al., 2009; Siebert et al., 2011; Rai and van Westrenen, 2014; Jennings et al., 2020).
View in article
It has been argued that tungsten becomes more siderophile with increasing pressure to ∼5 GPa and then less siderophile at higher pressures (Cottrell et al., 2009; Rai and van Westrenen, 2014).
View in article
This was attributed to the formation of W4+ (Cottrell et al., 2009) due to the emergence of octahedrally coordinated silicon in silicate melts (Sanloup et al., 2013).
View in article
Cottrell et al. (2009) examined the partitioning of tungsten between liquid iron and basaltic melt and showed positive and negative pressure effects on the metal/silicate partition coefficient below and above 2–6 GPa, respectively.
View in article
Cottrell et al. (2009) argued that such a change in the pressure effect may correspond to the emergence of W4+, but this was not supported by Wade et al. (2012) who found that the valence of tungsten remained six in silicate melt at 6 to 24 GPa based on augmented experimental data.
View in article
de Grouchy, C.J.L., Sanloup, C., Cochain, B., Drewitt, J.W.E., Kono, Y., Crépisson, C. (2017) Lutetium incorporation in magmas at depth: Changes in melt local environment and the influence on partitioning behaviour. Earth and Planetary Science Letters 464, 155–165.

The coordination environments of lutetium and xenon in silicate glasses and melts were examined by using in situ high pressure X-ray diffraction (XRD) techniques to 8 GPa (de Grouchy et al., 2017; Leroy et al., 2018).
View in article
It is worth emphasising that the pressure range for the coordination change of W6+ observed in this study is quite different from those for Ni2+, Co2+ (Keppler and Rubie, 1993), and Lu3+ (de Grouchy et al., 2017), whose coordination numbers increase rapidly with increasing pressure up to 10 GPa.
View in article
Farges, F., Linnen, R.L., Brown, G.E. Jr. (2006) Redox and speciation of tin in hydrous silicate glasses: A comparison with Nb, Ta, Mo and W. The Canadian Mineralogist 44, 775.

Below 10 GPa, W6+ is predominantly fourfold coordinated and therefore cannot be a network former in a silicate glass (Farges et al., 2006).
View in article
Hin, R.C., Burnham, A.D., Gianolio, D., Walter, M.J., Elliot, T. (2019) Molybdenum isotope fractionation between Mo4+ and Mo6+ in silicate liquid and metallic Mo. Chemical Geology 504, 177–189.

Therefore, the 182W/184W ratio in silicate melt equilibrated with metallic liquid above 32 GPa may be higher than that below 10 GPa, although the temperature for metal-silicate equilibrium at the time of core formation may have been too high to cause a resolvable isotopic fractionation, as is the case for Mo as reported by Hin et al. (2019).
View in article
Jennings, E.S., Jacobson, S.A., Rubie, D.C., Nakajima, Y., Vogel, A.K., Rose-Weston, L.A., Frost, D.J. (2020) Metal–silicate partitioning of W and Mo and the role of carbon in controlling their abundances in the bulk silicate earth. Geochimica et Cosmochimica Acta 293, 40–69.

Previous experiments have explored the effects of pressure, temperature, oxygen fugacity, and the compositions of metallic liquid and silicate melt on the metal-silicate partitioning of tungsten (Walter and Thibault, 1995; O’Neill et al., 2008; Cottrell et al., 2009; Siebert et al., 2011; Rai and van Westrenen, 2014; Jennings et al., 2020).
View in article
This could be the reason why tungsten is much less siderophile during metal-silicate partitioning when silicate melt exhibits higher NBO/T (the ratio of non-bridging oxygens per tetrahedrally coordinated cations, a measure of the depolymerisation) (Walter and Thibault, 1995) or contains more alkali metals and alkaline earth metals, in particular CaO (Jennings et al., 2020).
View in article
It has also been argued that the metal-silicate partitioning of tungsten strongly depends on the silicate melt composition represented by such as NBO/T or the CaO content as mentioned above (e.g., Walter and Thibault, 1995; Jennings et al., 2020).
View in article
Kashiwabara, T., Takahashi, Y., Marcus, M.A., Uruga, T., Tanida, H., Terada, Y., Usui, A. (2013) Tungsten species in natural ferromanganese oxides related to its different behavior from molybdenum in oxic ocean. Geochimica et Cosmochimica Acta 106, 364–378.

At ambient pressure, we obtained rW-O = 1.77 ± 0.02 Å in the basaltic glass, similar to rW-O = 1.78 Å in WO42− solution reported by Kashiwabara et al. (2013).
View in article
Kashiwabara, T., Kubo, S., Tanaka, M., Senda, R., Iizuka, T., Tanimizu, M., Takahashi, Y. (2017) Stable isotope fractionation of tungsten during adsorption on Fe and Mn (oxyhydr)oxides. Geochimica et Cosmochimica Acta 204, 52–67.

In general, heavier isotopes tend to be partitioned into a phase with a lower coordination number (Kashiwabara et al., 2017).
View in article
Keppler, H., Rubie, D.C. (1993) Pressure-induced coordination changes of transition-metal ions in silicate melts. Science 364, 54–56.

Keppler and Rubie (1993) reported the coordination changes of Ni2+ and Co2+ in silicate melts at high pressures up to 10 GPa based on crystal field spectroscopy on quenched glasses at ambient conditions.
View in article
It is worth emphasising that the pressure range for the coordination change of W6+ observed in this study is quite different from those for Ni2+, Co2+ (Keppler and Rubie, 1993), and Lu3+ (de Grouchy et al., 2017), whose coordination numbers increase rapidly with increasing pressure up to 10 GPa.
View in article
Leroy, C., Sanloup, C., Bureau, H., Schmidt, B.C., Konopkova, Z., Raepsaet, C. (2018) Bonding of xenon to oxygen in magmas at depth. Earth and Planetary Science Letters 484, 103–110.

The coordination environments of lutetium and xenon in silicate glasses and melts were examined by using in situ high pressure X-ray diffraction (XRD) techniques to 8 GPa (de Grouchy et al., 2017; Leroy et al., 2018).
View in article
McDonough, W.F. (2014) Compositional model for the Earth’s core. In: Holland, H.D., Turekian, K.K. (Eds.) Treatise on Geochemistry. Second Edition, Elsevier, Oxford, 559–577.

Since tungsten is a highly refractory element, its bulk Earth abundance is obtained from the chondritic abundance without correction for volatility (see review by McDonough, 2014), giving the tungsten content in the core from the known mantle abundance.
View in article
O’Neill, H.S.C., Berry, A.J., Eggins, S.M. (2008) The solubility and oxidation state of tungsten in silicate melts: Implications for the comparative chemistry of W and Mo in planetary differentiation processes. Chemical Geology 255, 346–359.

Previous experiments have explored the effects of pressure, temperature, oxygen fugacity, and the compositions of metallic liquid and silicate melt on the metal-silicate partitioning of tungsten (Walter and Thibault, 1995; O’Neill et al., 2008; Cottrell et al., 2009; Siebert et al., 2011; Rai and van Westrenen, 2014; Jennings et al., 2020).
View in article
This indicates that W6+ was predominantly tetrahedrally coordinated at 1 bar in our glass sample in agreement with O’Neill et al. (2008).
View in article
For example, according to the parameterisation by O’Neill et al. (2008), the partition coefficient of tungsten between metal and pyrolitic melt is forty times as high as that between metal and basaltic melt below 6 GPa.
View in article
Pauling, L. (1929) The principles determining the structure of complex ionic crystals. Journal of American Chemical Society 51, 1010–1026.

This is explained by the bond valence theory; oxygen cannot connect fourfold coordinated W6+ and Si4+ ions because the sum of the valence units combining W6+-O (VI/4 = 1.5) and Si4+-O bonds (IV/4 = 1.0) exceeds the valence (= 2) of an oxygen ion (Pauling, 1929).
View in article
Prescher, C., Prakapenka, V.B., Stefanski, J., Jahn, S., Skinner, L.B., Wang, Y. (2017) Beyond sixfold coordinated Si in SiO2 glass at ultrahigh pressures. Proceedings of the National Academy of Sciences 114, 10041–10046.

Pressure-induced structural changes in silicate melt and glasses have been examined, focusing on a change in the coordination of silicon (e.g., Sanloup et al., 2013; Prescher et al., 2017).
View in article
Filled squares (Prescher et al., 2017) and diamonds (Sato and Funamori, 2010) indicate data on SiO2 glass.
View in article
Previous XRD measurements have examined the pressure effect on the average Si-O bond length and the coordination number of silicon in SiO2 glass up to a megabar pressure (Prescher et al., 2017; Sato and Funamori, 2010).
View in article
The most recent experiments performed by Prescher et al. (2017) demonstrated that i) below 10 GPa, the Si-O bond length was constant at ∼1.62 Å, while volume was reduced because of the collapse of void space (topological change), ii) between 10 and 40 GPa, the Si-O bond length increased to ∼1.69 Å as a consequence of the increase in the mean silicon coordination number from four to six, and iii) above 40 GPa, the Si-O bond length slightly decreased with pressure, as illustrated in Figure 2.
View in article
Rai, N., van Westrenen, W. (2014) Lunar core formation: New constraints from metal–silicate partitioning of siderophile elements. Earth and Planetary Science Letters 388, 343–352.

Previous experiments have explored the effects of pressure, temperature, oxygen fugacity, and the compositions of metallic liquid and silicate melt on the metal-silicate partitioning of tungsten (Walter and Thibault, 1995; O’Neill et al., 2008; Cottrell et al., 2009; Siebert et al., 2011; Rai and van Westrenen, 2014; Jennings et al., 2020).
View in article
It has been argued that tungsten becomes more siderophile with increasing pressure to ∼5 GPa and then less siderophile at higher pressures (Cottrell et al., 2009; Rai and van Westrenen, 2014).
View in article
A more recent study by Rai and van Westrenen (2014) also demonstrated the positive pressure effect below 5 GPa before it changes to negative at higher pressures.
View in article
Sanloup, C., van Westrenen, W., Dasgupta, R., Maynard-Casely, H., Perrillat, J. (2011) Compressibility change in iron-rich melt and implications for core formation models. Earth and Planetary Science Letters 306, 118–122.

Sanloup et al. (2011) also pointed out that a change in the compressibility of liquid iron may affect the metal-silicate partitioning of trace elements including tungsten.
View in article
This might be attributed to the change in the structure of liquid iron alloy (Sanloup et al., 2011; Shibazaki et al., 2015), but the pressure response of the metal-silicate partitioning of tungsten is different from that of nickel and cobalt, which cannot be explained solely by the structural change in liquid metal.
View in article
Sanloup, C., Drewitt, J.W.E., Konopkova, Z., Dalladay-Simpson, P., Morton, D.M., Rai, N., van Westrenen, W., Morgenroth, W. (2013) Structural change in molten basalt at deep mantle conditions. Nature 503, 104–107.

This was attributed to the formation of W4+ (Cottrell et al., 2009) due to the emergence of octahedrally coordinated silicon in silicate melts (Sanloup et al., 2013).
View in article
Pressure-induced structural changes in silicate melt and glasses have been examined, focusing on a change in the coordination of silicon (e.g., Sanloup et al., 2013; Prescher et al., 2017).
View in article
Open squares are for a molten basalt (Sanloup et al., 2013).
View in article
Furthermore, the XRD study on a basaltic melt by Sanloup et al. (2013) showed that the Si-O bond length rapidly increased from <10 to ∼35 GPa (Fig. 2), consistent with the observations in SiO2 glass.
View in article
Sato, T., Funamori, N. (2010) High-pressure structural transformation of SiO2 glass up to 100 GPa. Physical Review B 82, 184102.

Filled squares (Prescher et al., 2017) and diamonds (Sato and Funamori, 2010) indicate data on SiO2 glass.
View in article
Previous XRD measurements have examined the pressure effect on the average Si-O bond length and the coordination number of silicon in SiO2 glass up to a megabar pressure (Prescher et al., 2017; Sato and Funamori, 2010).
View in article
Shannon, R. (1976) Revised effective ionic radii and systematic studies of interatomic distances in halides and chalcogenides. Acta Crystallographica Section A 32, 751–767.

This difference in the average W-O bond length is similar to the difference in the ionic radii between 0.42 and 0.60 Å for fourfold and sixfold coordinated W6+, respectively (Shannon, 1976).
View in article
Shibazaki, Y., Kono, Y., Fei, Y. (2015) Microscopic structural change in a liquid Fe-C alloy of ∼5 GPa. Geophysical Research Letters 42, 13.

This might be attributed to the change in the structure of liquid iron alloy (Sanloup et al., 2011; Shibazaki et al., 2015), but the pressure response of the metal-silicate partitioning of tungsten is different from that of nickel and cobalt, which cannot be explained solely by the structural change in liquid metal.
View in article
Siebert, J., Corgne, A., Ryerson, F.J. (2011) Systematics of metal-silicate partitioning for many siderophile elements applied to Earth’s core formation. Geochimica et Cosmochimica Acta 75, 1451–1489.

Previous experiments have explored the effects of pressure, temperature, oxygen fugacity, and the compositions of metallic liquid and silicate melt on the metal-silicate partitioning of tungsten (Walter and Thibault, 1995; O’Neill et al., 2008; Cottrell et al., 2009; Siebert et al., 2011; Rai and van Westrenen, 2014; Jennings et al., 2020).
View in article
Indeed, the earlier experiments conducted by Siebert et al. (2011) have demonstrated that the effect of NBO/T on metal-silicate partitioning is smaller for sixfold coordinated, high valence cations such as Nb5+ and Ta5+ than for fourfold coordinated W6+ and P5+.
View in article
Wade, J., Wood, B.J., Tuff, J. (2012) Metal–silicate partitioning of Mo and W at high pressures and temperatures: Evidence for late accretion of sulphur to the Earth. Geochimica et Cosmochimica Acta 85, 58–74.

Cottrell et al. (2009) argued that such a change in the pressure effect may correspond to the emergence of W4+, but this was not supported by Wade et al. (2012) who found that the valence of tungsten remained six in silicate melt at 6 to 24 GPa based on augmented experimental data.
View in article
Walter, M.J., Thibault, Y. (1995) Partitioning of tungsten and molybdenum between metallic liquid and silicate melt. Science 270, 1186–1189.

Previous experiments have explored the effects of pressure, temperature, oxygen fugacity, and the compositions of metallic liquid and silicate melt on the metal-silicate partitioning of tungsten (Walter and Thibault, 1995; O’Neill et al., 2008; Cottrell et al., 2009; Siebert et al., 2011; Rai and van Westrenen, 2014; Jennings et al., 2020).
View in article
This could be the reason why tungsten is much less siderophile during metal-silicate partitioning when silicate melt exhibits higher NBO/T (the ratio of non-bridging oxygens per tetrahedrally coordinated cations, a measure of the depolymerisation) (Walter and Thibault, 1995) or contains more alkali metals and alkaline earth metals, in particular CaO (Jennings et al., 2020).
View in article
It has also been argued that the metal-silicate partitioning of tungsten strongly depends on the silicate melt composition represented by such as NBO/T or the CaO content as mentioned above (e.g., Walter and Thibault, 1995; Jennings et al., 2020).
View in article
top
Supplementary Information
The Supplementary Information includes:
Download the Supplementary Information (PDF).
Figures
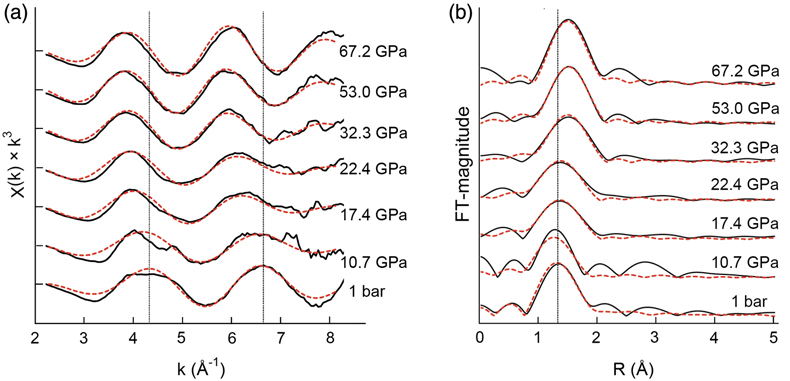
Figure 1 EXAFS oscillations of tungsten in a basaltic glass and FT-EXAFS spectra up to 67 GPa. (a) The k3-weighted oscillations, χ(k) × k3, extracted from EXAFS spectra (black curves). The vertical dotted lines highlight the wave numbers k of the first and second maxima of the oscillations at ambient pressure. (b) The radial structural function at the LIII-edge for tungsten, which was Fourier transformed (FT) from k3-weighted EXAFS oscillations (black curves). The vertical dotted line indicates the position of the maximum FT-magnitude at 1 bar. The red curves in (a) and (b) show simulation data of EXAFS spectra using the parameter extracted by FEFF.

Figure 2 Pressure-induced change in the W-O bond length (open circles) in basaltic glass on compression from the present EXAFS analyses, compared to those in the Si-O bond length. Filled squares (Prescher et al., 2017
Prescher, C., Prakapenka, V.B., Stefanski, J., Jahn, S., Skinner, L.B., Wang, Y. (2017) Beyond sixfold coordinated Si in SiO2 glass at ultrahigh pressures. Proceedings of the National Academy of Sciences 114, 10041–10046.
) and diamonds (Sato and Funamori, 2010Sato, T., Funamori, N. (2010) High-pressure structural transformation of SiO2 glass up to 100 GPa. Physical Review B 82, 184102.
) indicate data on SiO2 glass. Open squares are for a molten basalt (Sanloup et al., 2013Sanloup, C., Drewitt, J.W.E., Konopkova, Z., Dalladay-Simpson, P., Morton, D.M., Rai, N., van Westrenen, W., Morgenroth, W. (2013) Structural change in molten basalt at deep mantle conditions. Nature 503, 104–107.
). All the Si-O bond length data were obtained by XRD measurements.
Figure 3 Tungsten LIII-edge XANES spectra of a basaltic glass collected up to 67 GPa after background subtraction. (a) Normalised XANES spectra and (b) their second derivatives. In (b), the blue and red dashed lines indicate the position of the minimum of the second derivatives of the XANES spectrum at 1 bar and that of the local minimum that is prominent above 32.3 GPa, respectively. These XANES spectra show a clear difference between fourfold and sixfold coordinated W6+.