Direct evidence of CO2 drawdown through enhanced weathering in soils
Affiliations | Corresponding Author | Cite as | Funding information- Share this article
Article views:740Cumulative count of HTML views and PDF downloads.
- Download Citation
- Rights & Permissions
top
Abstract

Figures
![]() Figure 1 (a) Photograph of the studied soil system. The dark layers correspond to tephra originating from historic volcanic eruptions. (b) Schematic illustration of the soil profile with depths and ages (in years before present) of identified tephra layers shown in yellow for better visibility, the Landnám Layer that occurred around the time of the Icelandic settlement could not be clearly identified because of alteration. Plant remnants are visible in the lower part of the profile, indicating high organic content. | ![]() Figure 2 Measured soil water concentrations determined in the present study in all samples collected from May to November 2018, as a function of depth. The pH values are normalised to a 7 °C reference temperature and Eh values to a Standard Hydrogen Electrode. The black squares represent measured water concentrations, whereas the blue squares show the composition of rainwater. The error bars correspond to a ±5 % uncertainty on the measured concentrations; error bars do not appear if the uncertainty is smaller than the symbol size. The red curves in the figure show 2nd order polynomial fits of all the measured concentrations with the corresponding R2 values next to each curve. | ![]() Figure 3 Comparison of pH and alkalinity of soil waters collected from our studied soil with similarly composed, but volcanic dust-free soils reported in other studies. The black symbols correspond to alkalinity values reported in the literature for Histosols from bogs, poor or rich fens located in non-volcanic regions and in the absence of carbonate bedrock. The black boxes around the black symbols represent the commonly reported pH-alkalinity ranges of bogs and fens respectively. The red, orange, green and blue symbols represent soil water samples measured in the present study at the depths indicated in the figure. The purple symbol shows the composition of rainwater at our field site. The sources and location of the literature data are provided in Table S-3 of the Supplementary Information. | ![]() Figure 4 Schematic illustration of the processes drawing down CO2 at our field site. The site receives ∼1250 ± 200 kg m−2 yr−1 of rainfall. Of this rainfall 16 % is estimated to evaporate and 10 % is estimated to be lost to surface runoff. As the remaining 925 ± 150 kg m−2 yr−1 of water passes through the soil, its alkalinity increases on average from 0 to 2.59 ± 0.34 meq kg−1 at depth. Once these waters equilibrate with the atmosphere, this fluid oxidises and some CO2 is released such that the alkalinity decreases to 1.53 ± 0.2 meq kg−1 resulting in an annual export of 17 ± 3.6 g C per m2 soil surface area. At the same time 26–52 g m−2 yr−1 of C is drawn down from the atmosphere by organic carbon production and stored in the soil. |
Figure 1 | Figure 2 | Figure 3 | Figure 4 |
top
Introduction
The natural weathering of basaltic and ultramafic rocks has been demonstrated to have a relatively large role in the drawdown of CO2 from the atmosphere (Dessert et al., 2003
Dessert, C., Dupré, B., Gaillardet, J., François, L.M., Allègre, C.J. (2003) Basalt weathering laws and the impact of basalt weathering on the global carbon cycle. Chemical Geology 202, 257–273. https://doi.org/10.1016/j.chemgeo.2002.10.001
; Gislason et al., 2009Gislason, S.R., Oelkers, E.H., Eiriksdottir, E.S., Kardjilov, M.I., Gisladottir, G., Sigfusson, B., Snorrason, A., Elefsen, S., Hardardottir, J., Torssander, P., Oskarsson, N. (2009) Direct evidence of the feedback between climate and weathering. Earth and Planetary Science Letters 277, 213–222. https://doi.org/10.1016/j.epsl.2008.10.018
; Taylor et al., 2021Taylor, L.L., Driscoll, C.T., Groffman, P.M., Rau, G.H., Blum, J.D., Beerling, D.J. (2021) Increased carbon capture by a silicate-treated forested watershed affected by acid deposition. Biogeosciences 18, 169–188. https://doi.org/10.5194/bg-18-169-2021
). Such observations have motivated several proposals to use these rocks to remove CO2 directly from the atmosphere through a process called Enhanced Weathering (EW) (Moosdorf et al., 2014Moosdorf, N., Renforth, P., Hartmann, J. (2014) Carbon dioxide efficiency of terrestrial enhanced weathering. Environmental Science & Technology 48, 4809–4816. https://doi.org/10.1021/es4052022
; IPPC, 2018IPCC (2018) Global warming of 1.5°C. An IPCC Special Report on the impacts of global warming of 1.5°C above pre-industrial levels and related global greenhouse gas emission pathways, in the context of strengthening the global response to the threat of climate change, sustainable development, and efforts to eradicate poverty. Masson-Delmotte, V., Zhai, P., Pörtner, H.O., Roberts, D., Skea, J., Shukla, P.R., Pirani, A., Moufouma-Okia, W., Péan, C., Pidcock, R., Connors, S., Matthews, J.B.R., Chen, Y., Zhou, X., Gomis, M.I., Lonnoy, E., Maycock, T., Tignor, M., Waterfield, T. (Eds.) World Meteorological Organization, Geneva, Switzerland. https://www.ipcc.ch/site/assets/uploads/sites/2/2019/06/SR15_Full_Report_High_Res.pdf
; Beerling et al., 2020Beerling, D.J., Kantzas, E.P., Lomas, M.R., Wade, P., Eufrasio, R.M., Renforth, P., Sarkar, B., Andrews, M.G., James, R.H., Pearce, C.R., Mercure, J.-F., Pollitt, H., Holden, P.B., Edwards, N.R., Khanna, M., Koh, L., Quegan, S., Pidgeon, N.F., Janssens, I.A., Hansen, J., Banwart, S.A. (2020) Potential for large-scale CO2 removal via enhanced rock weathering with croplands. Nature 583, 242–248. https://doi.org/10.1038/s41586-020-2448-9
). Enhanced weathering involves amending soils with crushed fine grained, fast reacting Ca-Mg silicate rocks and minerals such as basalts and peridotites (Strefler et al., 2018Strefler, J., Amann, T., Bauer, N., Kriegler, E., Hartmann, J. (2018) Potential and costs of carbon dioxide removal by enhanced weathering of rocks. Environmental Research Letters 13, 034010. https://doi.org/10.1088/1748-9326/aaa9c4
). To date, enhanced weathering field experiments have demonstrated improved crop vigour, organic and inorganic carbon storage and decreased N2O degassing (Haque et al., 2019aHaque, F., Santos, R.M., Dutta, A., Thimmanagari, M., Chiang, Y.W. (2019a) Co-Benefits of Wollastonite Weathering in Agriculture: CO2 Sequestration and Promoted Plant Growth. ACS Omega 4, 1425–1433. https://doi.org/10.1021/acsomega.8b02477
, 2020Haque, F., Santos, R.M., Chiang, Y.W. (2020) CO2 sequestration by wollastonite-amended agricultural soils – An Ontario field study. International Journal of Greenhouse Gas Control 97, 103017. https://doi.org/10.1016/j.ijggc.2020.103017
; Beerling et al., 2020Beerling, D.J., Kantzas, E.P., Lomas, M.R., Wade, P., Eufrasio, R.M., Renforth, P., Sarkar, B., Andrews, M.G., James, R.H., Pearce, C.R., Mercure, J.-F., Pollitt, H., Holden, P.B., Edwards, N.R., Khanna, M., Koh, L., Quegan, S., Pidgeon, N.F., Janssens, I.A., Hansen, J., Banwart, S.A. (2020) Potential for large-scale CO2 removal via enhanced rock weathering with croplands. Nature 583, 242–248. https://doi.org/10.1038/s41586-020-2448-9
). One of the goals of EW is to increase the alkalinity export of waters that drain from soils and enter rivers and streams. The quantification of carbon drawdown by enhanced weathering has been challenging to identify or quantify due to the large number of processes that occur in soils and the short duration of existing field studies.One approach to investigate the long term behaviour and consequences of EW is via natural analogues. Enhanced weathering experiments to date have tested the addition of up to 400 t ha−1 yr−1 of crushed Ca-Mg silicate rocks to agricultural soils (Gillman et al., 2002
Gillman, G.P., Burkett, D.C., Coventry, R.J. (2002) Amending highly weathered soils with finely ground basalt rock. Applied Geochemistry 17, 987–1001. https://doi.org/10.1016/S0883-2927(02)00078-1
; Amann et al., 2018Amann, T., Hartmann, J., Struyf, E., de Oliveira Garcia, W., Fischer, E.K., Janssens, I., Meire, P., Schoelynck, J. (2018) Constraints on Enhanced Weathering and related carbon sequestration – a cropland mesocosm approach. Biogeosciences Discussions 1–21. https://doi.org/10.5194/bg-2018-398
; Haque et al., 2019bHaque, F., Chiang, Y., Santos, R. (2019b) Alkaline Mineral Soil Amendment: A Climate Change ‘Stabilization Wedge’? Energies 12, 2299. https://doi.org/10.3390/en12122299
). This crushed rock flux is orders of magnitude higher than the average global desert dust deposition on Earth, which is estimated to be 0.5 t ha−1 yr−1 (Mahowald et al., 2005Mahowald, N.M., Baker, A.R., Bergametti, G., Brooks, N., Duce, R.A., Jickells, T.D., Kubilay, N., Prospero, J.M., Tegen, I. (2005) Atmospheric global dust cycle and iron inputs to the ocean. Global Biogeochemical Cycles 19, GB4025. https://doi.org/10.1029/2004GB002402
). In the vicinity of the dust “hot spots”, such as South Iceland, however, the mass of deposited fine grained basaltic dust can be as high as 8 t ha−1 yr−1 (Arnalds, 2010Arnalds, O. (2010) Dust sources and deposition of aeolian materials in Iceland. Icelandic Agricultural Sciences 23, 3–21.
; Arnalds et al., 2014Arnalds, O., Olafsson, H., Dagsson-Waldhauserova, P. (2014) Quantification of iron-rich volcanogenic dust emissions and deposition over the ocean from Icelandic dust sources. Biogeosciences 11, 6623–6632. https://doi.org/10.5194/bg-11-6623-2014
, 2016Arnalds, O., Dagsson-Waldhauserova, P., Olafsson, H. (2016) The Icelandic volcanic aeolian environment: Processes and impacts — A review. Aeolian Research 20, 176–195. https://doi.org/10.1016/j.aeolia.2016.01.004
). Although this natural mass flux of basalt is less than that of current EW efforts, 1) this basaltic dust flux has been added continuously to the soil of this region over at least the past 3300 years, such that in total over 16,500 t ha−1 of basaltic dust has been added over this time, and 2) the specific surface area of natural basaltic dust is likely higher than that added to soils in current EW experiments due to its finer grain size. The grain size of the crushed rocks used in EW applications, if reported, is commonly less than 150 μm (Haque et al., 2019aHaque, F., Santos, R.M., Dutta, A., Thimmanagari, M., Chiang, Y.W. (2019a) Co-Benefits of Wollastonite Weathering in Agriculture: CO2 Sequestration and Promoted Plant Growth. ACS Omega 4, 1425–1433. https://doi.org/10.1021/acsomega.8b02477
; Gillman et al., 2002Gillman, G.P., Burkett, D.C., Coventry, R.J. (2002) Amending highly weathered soils with finely ground basalt rock. Applied Geochemistry 17, 987–1001. https://doi.org/10.1016/S0883-2927(02)00078-1
). In contrast, the average size of basaltic Icelandic dust ranges from 10 to 62 μm (Arnalds et al., 2014Arnalds, O., Olafsson, H., Dagsson-Waldhauserova, P. (2014) Quantification of iron-rich volcanogenic dust emissions and deposition over the ocean from Icelandic dust sources. Biogeosciences 11, 6623–6632. https://doi.org/10.5194/bg-11-6623-2014
; Liu et al., 2014Liu, E.J., Cashman, K.V., Beckett, F.M., Witham, C.S., Leadbetter, S.J., Hort, M.C., Gudmudsson, S. (2014) Ash mists and brown snow: Remobilization of volcanic ash from recent Icelandic eruptions. Journal of Geophysical Research: Atmospheres 119, 9463–9480. https://doi.org/10.1002/2014JD021598
). For these reasons, the mineral rich Histic/Gleyic Andosols (Arnalds, 2015Arnalds, O. (2015) The Soils of Iceland. World Soils Book Series, Springer, Dordrecht. https://doi.org/10.1007/978-94-017-9621-7
) considered in this study located in South Iceland provide an insightful natural analogue to illuminate the long term effect of EW applications performed under similar climate, vegetation, and soil conditions. The studied soil receives large amounts of air borne volcanic material during 1) explosive volcanic eruptions in the form of glassy volcanic ash fallout, and 2) dust storms (Shoji et al., 1995Shoji, S., Nanzyo, M., Dahlgren, R. (1995) Volcanic Ash Soils: Genesis, Properties and Utilization. Developments in Soil Science, Elsevier, Amsterdam.
; Arnalds et al., 2016Arnalds, O., Dagsson-Waldhauserova, P., Olafsson, H. (2016) The Icelandic volcanic aeolian environment: Processes and impacts — A review. Aeolian Research 20, 176–195. https://doi.org/10.1016/j.aeolia.2016.01.004
). Explosive eruptions lead to evident tephra horizons that can be used to date these soils. The more frequently deposited windblown dust is finer grained than the tephra and intermingled with the soil organic carbon (see “Soil classification and soil evolution in Iceland” in Supplementary Information). Based on palaeoecologic research (Gísladóttir et al., 2008Gísladóttir, G., Erlendsson, E., Lal, R., Bigham, J. (2008) Erosional Effects on Terrestrial Resources over the last Millennium in Reykjanes, Southwest Iceland. Quaternary Research 73, 20–32. https://doi.org/10.1016/j.yqres.2009.09.007
; Arnalds, 2015Arnalds, O. (2015) The Soils of Iceland. World Soils Book Series, Springer, Dordrecht. https://doi.org/10.1007/978-94-017-9621-7
; Möckel et al., 2017Möckel, S.C., Erlendsson, E., Gísladóttir, G. (2017) Holocene environmental change and development of the nutrient budget of histosols in North Iceland. Plant and Soil 418, 437–457. https://doi.org/10.1007/s11104-017-3305-y
), in the absence of volcanic dust input, the Histic/Gleyic Andosols of Southern Iceland would have developed into Histosols (Arnalds, 2008Arnalds, O. (2008) Soils of Iceland. Jökull 58, 409–421. https://doi.org/10.33799/jokull2008.58.409
). Consequently, the comparison of the behaviour of South Icelandic Histic/Gleyic Andosols with that of volcanic dust-free Histosols, located in similar climatic zones provides insight into the consequences of adding fine grained basaltic material to soils as part of enhanced weathering efforts.One of our motivations to focus on the addition of volcanic material to Histosols/peat soils to drawdown CO2 from the atmosphere stems from the role of these soils in the global carbon cycle. Although peatlands cover only about 3 % of the continents (Xu et al., 2018
Xu, J., Morris, P.J., Liu, J., Holden, J. (2018) PEATMAP: Refining estimates of global peatland distribution based on a meta-analysis. Catena 160, 134–140. https://doi.org/10.1016/j.catena.2017.09.010
), they store ∼10 % of all non-glacial freshwater and roughly 30 % of the land-based organic carbon (Mitra et al., 2005Mitra, S., Wassmann, R., Vlek, P.L.G. (2005) An appraisal of global wetland area and its organic carbon stock. Current Science 88, 25–35. http://www.jstor.org/stable/24110090
; Bragazza et al., 2013Bragazza, L., Parisod, J., Buttler, A., Bardgett, R.D. (2013) Biogeochemical plant-soil microbe feedback in response to climate warming in peatlands. Nature Climate Change 3, 273–277. https://doi.org/10.1038/nclimate1781
). Man made drainage and burning of peat areas worldwide releases 0.5–0.8 Gt C yr−1, which is equivalent to 5–8 % of global anthropogenic carbon emissions (Hooijer et al., 2006Hooijer, A., Silvius, M., Wösten, H., Page, S. (2006) PEAT-CO2, Assessment of CO2 emissions from drained peatlands in SE Asia. Delft Hydraulic Report Q3943.
; Parish et al., 2008Parish, F., Sirin, A., Charman, D., Joosten, H., Minayeva, T., Silvius, M., Stringer, L. (2008) Assessment on Peatlands, Biodiversity and Climate Change: Main Report. Global Environment Centre, Kuala Lumpur and Wetlands International, Wageningen.
). Carbon dioxide emission from the drainage of peat areas is estimated to be the largest anthropogenic source of CO2 emissions in Iceland (Keller et al., 2020Keller, N., Stefani, M., Einarsdóttir, S., Helgadóttir, A., Gudmundsson, J., Snorrason, A., Thorsson, J., Tinganelli, L. (2020) National Inventory Report - Emissions of greenhouse gases in Iceland from 1990 to 2018. The Environment Agency of Iceland, Reykjavík.
). The addition of reactive silicate rock dust to peat soils might help increase carbon storage being otherwise lost due to peatland draining.This manuscript is one of two exploring the long term efficiency and consequences of enhanced rock weathering efforts through the study of a South Iceland Gleyic/Histic Andosol. The first manuscript (Linke et al., 2024
Linke, T., Oelkers, E.H., Dideriksen, K., Möckel, S.C., Nilabh, S., Grandia, F., Gislason, S.R. (2024) The geochemical evolution of basalt Enhanced Rock Weathering systems quantified from a natural analogue. Geochimica et Cosmochimica Acta 370, 66–77. https://doi.org/10.1016/j.gca.2024.02.005
) reports the composition of fluids and solids collected over two field seasons to 1) quantify the saturation state of the primary and secondary mineral phases with respect to the soil solutions, 2) determine the processes controlling the mobility of heavy metals, and 3) assess the rate at which basalt dissolved in the soils. In this manuscript we present a comparison of the alkalinity export from this soil with corresponding results from volcanic dust-free Histosols to quantify the ability of enhanced weathering efforts to drawdown CO2 from the atmosphere. Results are then used to estimate the efficiency of enhanced weathering at a larger scale. The purpose of this paper is to present the results of this study and use the results to gain insight into the consequences of current and future enhanced weathering efforts.top
Field Site Description
The field site is located above the source of the Rauðalækur (“Red creek”) river at 63° 53' 42.5" N, 20° 21' 15.9" W, which is approximately 7 km north of the town of Hella in South Iceland. This site consists of an upper Gleyic Andosol and a lower Histic Andosol (see Supplementary Information for further details). The study site receives an annual aeolian dust flux of 5–8 t ha−1 yr−1, consisting of mostly basaltic glass (Arnalds et al., 2016
Arnalds, O., Dagsson-Waldhauserova, P., Olafsson, H. (2016) The Icelandic volcanic aeolian environment: Processes and impacts — A review. Aeolian Research 20, 176–195. https://doi.org/10.1016/j.aeolia.2016.01.004
). Additional basaltic material is added during irregular volcanic events. During the past several decades, drainage trenches have been cut into the nearby soils; the closest drainage ditch is located more than 150 metres from the study site. The studied soil contains several prominent horizontal tephra layers, with thicknesses ranging from a few mm to few cm and deposited during the past 3300 years. An image and schematic illustration of the system is provided in Figure 1 and a detailed description of the field site is provided in the Supplementary Information and Table S-1 therein.
Figure 1 (a) Photograph of the studied soil system. The dark layers correspond to tephra originating from historic volcanic eruptions. (b) Schematic illustration of the soil profile with depths and ages (in years before present) of identified tephra layers shown in yellow for better visibility, the Landnám Layer that occurred around the time of the Icelandic settlement could not be clearly identified because of alteration. Plant remnants are visible in the lower part of the profile, indicating high organic content.
top
Results
Fluid compositions. Soil fluid samples were collected using suction cup samplers from May to November 2018. The compositions of all fluid samples are provided in Table S-2 (Supplementary Information) and selected dissolved constituents are shown as a function of depth in Figure 2. The pH of the samples was recalculated using PHREEQC (Parkhurst and Appelo, 1999
Parkhurst, D.L., Appelo, C.A.J. (1999) User’s guide to PHREEQC (Version 2): A computer program for speciation, batch-reaction, one-dimensional transport, and inverse geochemical calculations. Water-Resources Investigations Report 99-4259, US Geological Survey, Denver. https://doi.org/10.3133/wri994259
) to the in situ soil temperature of 7 °C. This is the average soil temperature at 76–260 cm depth during the summer months (Petersen and Gerber, 2018)Petersen, G.N., Berber, D. (2018) Soil temperature measurements in Iceland, status and future outlook vision (in Icelandic: Jarðvegshitamælingar á Íslandi. Staða núverandi kerfis og framtíðarsýn). Report of the Icelandic Meteorological Office (Skýrsla Veðurstofu Íslands), VÍ 2018-009, Reykjavík, Iceland. https://www.vedur.is/media/vedurstofan-utgafa-2018/VI_2018_009_rs.pdf
. The concentrations of major elements increase continuously with depth suggesting the continuous dissolution of the basaltic dust in the soil. The soil waters become increasingly anoxic with depth as indicated by the Eh values shown in Figure 2b.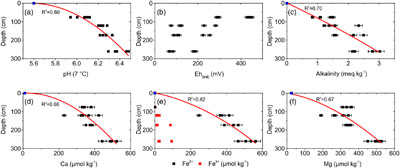
Figure 2 Measured soil water concentrations determined in the present study in all samples collected from May to November 2018, as a function of depth. The pH values are normalised to a 7 °C reference temperature and Eh values to a Standard Hydrogen Electrode. The black squares represent measured water concentrations, whereas the blue squares show the composition of rainwater. The error bars correspond to a ±5 % uncertainty on the measured concentrations; error bars do not appear if the uncertainty is smaller than the symbol size. The red curves in the figure show 2nd order polynomial fits of all the measured concentrations with the corresponding R2 values next to each curve.
The alkalinity of the soil waters increases from 0 to 3 meq kg−1 with depth. Once these waters exit the soils, they will equilibrate with the O2 and CO2 in the atmosphere. PHREEQC calculations indicate that the alkalinity of the soil waters will decrease on average to 1.53 ± 0.2 meq kg−1 due to iron oxidation/precipitation reactions when they come in contact with the atmosphere as they flow into local rivers (further details of this calculation are provided in the Supplementary Information).
The alkalinity of soil waters in our studied dust-rich soil are compared to the corresponding alkalinities of basalt dust-free Histosols located in non-carbonate terrains in Figure 3. Our field site, mostly fed by rainwater, shows considerably higher alkalinity and pH values than observed in corresponding basalt dust-free Histosols. Histosols located in carbonate terrains are not included in this comparison as the presence of carbonate minerals leads to a pH and alkalinity increase due to carbonate dissolution, a process which has no long term net effect on atmospheric carbon drawdown. The comparison in Figure 3 shows that the addition of volcanic dust to our soil increased substantially the alkalinity in its soil waters, most notably deep in the soil column. This observation confirms the ability of enhanced weathering by the addition of basaltic dust to soils to drawdown CO2 from the atmosphere.
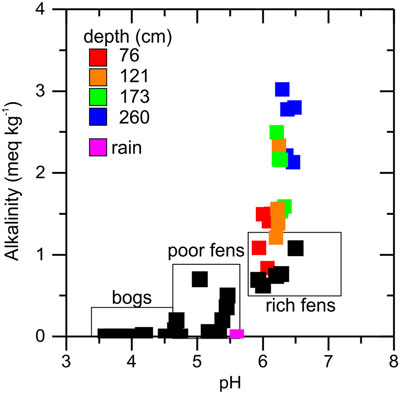
Figure 3 Comparison of pH and alkalinity of soil waters collected from our studied soil with similarly composed, but volcanic dust-free soils reported in other studies. The black symbols correspond to alkalinity values reported in the literature for Histosols from bogs, poor or rich fens located in non-volcanic regions and in the absence of carbonate bedrock. The black boxes around the black symbols represent the commonly reported pH-alkalinity ranges of bogs and fens respectively. The red, orange, green and blue symbols represent soil water samples measured in the present study at the depths indicated in the figure. The purple symbol shows the composition of rainwater at our field site. The sources and location of the literature data are provided in Table S-3 of the Supplementary Information.
A noteworthy observation is that the basaltic dust in the studied soil column persists and is reactive throughout the soil column, despite the fact that some of this dust has been present in the soil for 3300 years. This observation is consistent with mass balance estimates of the import and export of metals to the soil column. The study site receives an average annual dust flux of 500–800 g m−2 yr−1. This basalt flux adds 0.96–1.54 mol Ca and 0.72–1.16 mol Mg per m2 yr−1 to the soil. In contrast, the average Ca and Mg concentration of the deep soil water is 5 × 10−4 and 4 × 10−4 mol kg−1 for Ca and Mg, respectively. Taking account of the estimated 925 ± 150 kg m−2 yr−1 of water that flows through, and is exported annually by our studied soil (see Supplementary Information for details of this water flow estimate), we estimate that 0.47 ± 0.07 and 0.37 ± 0.06 mol yr−1 of Ca and Mg, respectively, are removed from the soil per square metre of soil surface area at present. The input of Ca and Mg by volcanic dust addition is, therefore, approximately 2–3 times more than that removed by soil water export. The results of this comparison are consistent with the persistence of the reactive dust throughout the soil column and suggest the long term viability of enhanced weathering efforts.
Carbon Storage via alkalinity export by the addition of basaltic material to soils. The rate of carbon drawdown due to alkalinity export by enhanced weathering in our studied field site can be estimated by combining the annual water flux through the soil and the measured alkalinity, as demonstrated in Figure 4. By taking account of the rainfall, evaporation, and surface runoff it is estimated that 925 ± 150 kg m−2 yr−1 of water pass through and are exported from the studied soil annually. Multiplying this number by the 1.53 ± 0.2 meq kg−1 average alkalinity of the deepest water samples of our study area, after its equilibration with the atmosphere, yields an estimated alkalinity export from our soils of 1.43 ± 0.3 meq m−2 yr−1. Multiplying this number by the atomic weight of carbon yields an annual carbon addition to our river water of 17 ± 3.6 g m−2 yr−1, which equals 0.17 ± 0.036 t ha−1 yr−1 of C. The degree to which this carbon drawdown rate is influenced by the rate of basalt input to the soil and its surface area has yet to be quantified. Although, dissolution rates are commonly thought to be proportional to the fluid-mineral surface area, these rates are also influenced by fluid compositions, including approach to equilibrium and fluid flow paths in the soil column (Schott et al. 2009
Schott, J., Pokrovsky, O.S., Oelkers, E.H. (2009) The link between mineral dissolution/precipitation kinetics and solution chemistry. Reviews in Mineralogy 70, 207–258. https://doi.org/10.2138/rmg.2009.70.6
; Linke et al., 2024Linke, T., Oelkers, E.H., Dideriksen, K., Möckel, S.C., Nilabh, S., Grandia, F., Gislason, S.R. (2024) The geochemical evolution of basalt Enhanced Rock Weathering systems quantified from a natural analogue. Geochimica et Cosmochimica Acta 370, 66–77. https://doi.org/10.1016/j.gca.2024.02.005
).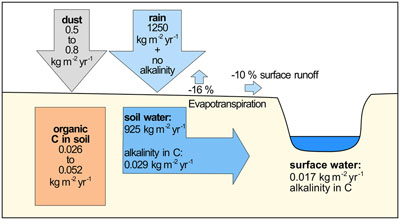
Figure 4 Schematic illustration of the processes drawing down CO2 at our field site. The site receives ∼1250 ± 200 kg m−2 yr−1 of rainfall. Of this rainfall 16 % is estimated to evaporate and 10 % is estimated to be lost to surface runoff. As the remaining 925 ± 150 kg m−2 yr−1 of water passes through the soil, its alkalinity increases on average from 0 to 2.59 ± 0.34 meq kg−1 at depth. Once these waters equilibrate with the atmosphere, this fluid oxidises and some CO2 is released such that the alkalinity decreases to 1.53 ± 0.2 meq kg−1 resulting in an annual export of 17 ± 3.6 g C per m2 soil surface area. At the same time 26–52 g m−2 yr−1 of C is drawn down from the atmosphere by organic carbon production and stored in the soil.
It is insightful to extrapolate this annual rate of carbon drawdown to a larger scale. If the results of our studied field site are representative, the removal of 1 Gt yr−1 CO2 from the atmosphere through alkalinity production would require a total of 16 million km2 of surface. This is larger than the total surface area of the United States. Moreover, the mass of basaltic dust required to provoke this rate of carbon removal may be unrealistically large. The average annual flux of basaltic dust into the studied South Iceland soils is 5–8 t ha−1 yr−1. Adding this mass of basalt over 16 million km2 of surface would require 8 to 13 Gt of finely ground basalt annually. This mass of ground basalt is larger than the world’s annual cement production of 4.3 Gt in 2020 (https://iea.org/reports/cement). This conclusion, based on the alkalinity export from our studied soil, which contains more organic matter (12 % to >20 % C) than most soils globally (<5 % C; e.g., Stockmann et al., 2015
Stockmann, U., Padarian, J., McBratney, A., Minasny, B., de Brogniez, D., Montanarella, L., Hong, S.Y., Rawlins, B.G., Field, D.J. (2015) Global soil organic carbon assessment. Global Food Security 6, 9–16 https://doi.org/10.1016/j.gfs.2015.07.001
), is nevertheless supported by other recent enhanced rock weathering studies. Our result of 0.17 ± 0.036 t ha−1 yr−1 of carbon drawdown from alkalinity export by enhanced rock weathering is within the range of the handful of large scale cropland EW studies of 0.0005–0.5 t C ha−1 yr−1 (Haque et al., 2020Haque, F., Santos, R.M., Chiang, Y.W. (2020) CO2 sequestration by wollastonite-amended agricultural soils – An Ontario field study. International Journal of Greenhouse Gas Control 97, 103017. https://doi.org/10.1016/j.ijggc.2020.103017
; Taylor et al., 2021Taylor, L.L., Driscoll, C.T., Groffman, P.M., Rau, G.H., Blum, J.D., Beerling, D.J. (2021) Increased carbon capture by a silicate-treated forested watershed affected by acid deposition. Biogeosciences 18, 169–188. https://doi.org/10.5194/bg-18-169-2021
; Larkin et al., 2022Larkin, C.S., Andrews, M.G., Pearce, C.R., Yeong, K.L., Beerling, D.J., Bellamy, J., Benedick, S., Freckleton, R.P., Goring-Harford, H., Sadekar, S., James, R.H. (2022) Quantification of CO2 removal in a large-scale enhanced weathering field trial on an oil palm plantation in Sabah, Malaysia. Frontiers in Climate 4, 959229. https://doi.org/10.3389/fclim.2022.959229
). It should be noted, however, that the alkalinity generated in our studied Histic/Gleyic Andosol was the consequence of the dissolution of the basalt added to this soil annually over the past 3300 years. This annual addition has led to a buildup of basaltic material over time. The results shown in Figure 2 indicate that the presence of older basaltic dust, located deep in the soil profile is an important contributor to alkalinity production. As such, it seems likely that substantially more than 5–8 t ha−1 yr−1 would need to be added to soils near-term as part of enhanced weathering efforts to provoke a similar rate of alkalinity production as observed in our study area.Carbon drawdown by alkalinity production versus soil organic carbon. The total mass of organic carbon in our studied soil is estimated to be 86–172 kg C m−2 with average net annual rate of carbon drawdown estimated to be 26–52 g C m−2 yr−1 (see Supplementary Information). This rate of CO2 drawdown is substantially larger than the corresponding 17 ± 3.6 g C m−2 yr−1 drawdown due to alkalinity export in our studied soils. These estimates are in agreement with previous studies (Taylor et al., 2021
Taylor, L.L., Driscoll, C.T., Groffman, P.M., Rau, G.H., Blum, J.D., Beerling, D.J. (2021) Increased carbon capture by a silicate-treated forested watershed affected by acid deposition. Biogeosciences 18, 169–188. https://doi.org/10.5194/bg-18-169-2021
). These estimates also suggest that the amount of CO2 removed by the addition of basaltic dust to the soil in one year by alkalinity export is more than 3 orders of magnitude less than the total CO2 stored as organic carbon in the soil. This latter observation should serve as a warning to those attempting atmospheric CO2 drawdown by enhanced weathering in soils. If the addition of basaltic dust to soil leads to the accelerated decomposition of organic material in soils, the latter process could readily dominate leading to a net increase of CO2 released to the atmosphere due to enhanced weathering efforts.top
Conclusions
The results of this study confirm the ability of fine grained basaltic rock added to soils to enhance CO2 drawdown directly from the atmosphere due to alkalinity production. It is estimated that 17 ± 3.6 g C m−2 yr−1 is currently drawn down and added to rivers by alkalinity production from our South Iceland field site. The enhanced alkalinity production of our soils was produced by the addition of approximately 1.7–2.6 t m−2 of basaltic dust to this soil over 3300 years. Upscaling of this process to address even a small fraction of the mass of anthropogenic CO2 emissions to the atmosphere, however, may be challenging because 1) this enhanced weathering process is slow and would require more land than is available for a sizeable drawdown of CO2 through alkalinity production, and 2) the currently unquantified effect of adding basalt powder to soils on soil organic matter. So, although this study demonstrates the potential of enhanced weathering efforts to contribute to attenuating atmospheric CO2 concentrations, the degree to which this approach will prove successful at a larger scale remains unclear.
top
Acknowledgements
The authors thank Knud Dideriksen for his comments and discussions during the field work. We thank the Icelandic meteorological office (Veðurstofa Íslands) for providing rainwater data, Liane Benning and the interface geochemistry group at the Geological research centre GFZ Potsdam for the DOC measurements, Þorsteinn Jónsson for assisting in the field and designing coring equipment and Egill Erlendsson and Guðrún Gísladóttir at University of Iceland for helping with site selection. We thank Bryndís Róbertsdóttir at the National Energy Authority Iceland for her help with identifying the tephra layers and Eirikur Benjaminsson for access to his property. We thank Susan Stipp and Dominique Tobler, the Metal-Aid coordinators, as well as the network members for their pleasant company during this study. This project was funded by the European Union’s Horizon 2020 research and innovation programme under the Marie Skłodowska-Curie grant agreement No 675219, from Landsvirkjun under the project number 2456, and the Icelandic Center for Research (Rannís) on behalf of the Doctoral Student Fund of the Ministry for the Environment and Natural Resources under the grant No 218929-051. Additional financial support was received from the Research Fund of the University of Iceland and the Travel grant for doctoral students at the University of Iceland. This research was partly supported by research grant CRG9 2020 KAUST-UI. SRG and EHO would like to thank Hussein Hoteit and Abdulkader M. Alafifi at KAUST University, Saudi Arabia for their hospitality and support.
Editor: Satish Myneni
top
References
Amann, T., Hartmann, J., Struyf, E., de Oliveira Garcia, W., Fischer, E.K., Janssens, I., Meire, P., Schoelynck, J. (2018) Constraints on Enhanced Weathering and related carbon sequestration – a cropland mesocosm approach. Biogeosciences Discussions 1–21. https://doi.org/10.5194/bg-2018-398

Enhanced weathering experiments to date have tested the addition of up to 400 t ha−1 yr−1 of crushed Ca-Mg silicate rocks to agricultural soils (Gillman et al., 2002; Amann et al., 2018; Haque et al., 2019b).
View in article
Arnalds, O. (2008) Soils of Iceland. Jökull 58, 409–421. https://doi.org/10.33799/jokull2008.58.409

The more frequently deposited windblown dust is finer grained than the tephra and intermingled with the soil organic carbon (see “Soil classification and soil evolution in Iceland” in Supplementary Information). Based on palaeoecologic research (Gísladóttir et al., 2008; Arnalds, 2015; Möckel et al., 2017), in the absence of volcanic dust input, the Histic/Gleyic Andosols of Southern Iceland would have developed into Histosols (Arnalds, 2008).
View in article
Arnalds, O. (2010) Dust sources and deposition of aeolian materials in Iceland. Icelandic Agricultural Sciences 23, 3–21.

In the vicinity of the dust “hot spots”, such as South Iceland, however, the mass of deposited fine grained basaltic dust can be as high as 8 t ha−1 yr−1 (Arnalds, 2010; Arnalds et al., 2014, 2016).
View in article
Arnalds, O. (2015) The Soils of Iceland. World Soils Book Series, Springer, Dordrecht. https://doi.org/10.1007/978-94-017-9621-7

In the vicinity of the dust “hot spots”, such as South Iceland, however, the mass of deposited fine grained basaltic dust can be as high as 8 t ha−1 yr−1 (Arnalds, 2010; Arnalds et al., 2014, 2016).
View in article
In contrast, the average size of basaltic Icelandic dust ranges from 10 to 62 μm (Arnalds et al., 2014; Liu et al., 2014).
View in article
Arnalds, O., Olafsson, H., Dagsson-Waldhauserova, P. (2014) Quantification of iron-rich volcanogenic dust emissions and deposition over the ocean from Icelandic dust sources. Biogeosciences 11, 6623–6632. https://doi.org/10.5194/bg-11-6623-2014

For these reasons, the mineral rich Histic/Gleyic Andosols (Arnalds, 2015) considered in this study located in South Iceland provide an insightful natural analogue to illuminate the long term effect of EW applications performed under similar climate, vegetation, and soil conditions.
View in article
The more frequently deposited windblown dust is finer grained than the tephra and intermingled with the soil organic carbon (see “Soil classification and soil evolution in Iceland” in Supplementary Information). Based on palaeoecologic research (Gísladóttir et al., 2008; Arnalds, 2015; Möckel et al., 2017), in the absence of volcanic dust input, the Histic/Gleyic Andosols of Southern Iceland would have developed into Histosols (Arnalds, 2008).
View in article
Arnalds, O., Dagsson-Waldhauserova, P., Olafsson, H. (2016) The Icelandic volcanic aeolian environment: Processes and impacts — A review. Aeolian Research 20, 176–195. https://doi.org/10.1016/j.aeolia.2016.01.004

In the vicinity of the dust “hot spots”, such as South Iceland, however, the mass of deposited fine grained basaltic dust can be as high as 8 t ha−1 yr−1 (Arnalds, 2010; Arnalds et al., 2014, 2016).
View in article
The studied soil receives large amounts of air borne volcanic material during 1) explosive volcanic eruptions in the form of glassy volcanic ash fallout, and 2) dust storms (Shoji et al., 1995; Arnalds et al., 2016).
View in article
The study site receives an annual aeolian dust flux of 5–8 t ha−1 yr−1, consisting of mostly basaltic glass (Arnalds et al., 2016).
View in article
Beerling, D.J., Kantzas, E.P., Lomas, M.R., Wade, P., Eufrasio, R.M., Renforth, P., Sarkar, B., Andrews, M.G., James, R.H., Pearce, C.R., Mercure, J.-F., Pollitt, H., Holden, P.B., Edwards, N.R., Khanna, M., Koh, L., Quegan, S., Pidgeon, N.F., Janssens, I.A., Hansen, J., Banwart, S.A. (2020) Potential for large-scale CO2 removal via enhanced rock weathering with croplands. Nature 583, 242–248. https://doi.org/10.1038/s41586-020-2448-9

Such observations have motivated several proposals to use these rocks to remove CO2 directly from the atmosphere through a process called Enhanced Weathering (EW) (Moosdorf et al., 2014; IPPC, 2018; Beerling et al., 2020).
View in article
To date, enhanced weathering field experiments have demonstrated improved crop vigour, organic and inorganic carbon storage and decreased N2O degassing (Haque et al., 2019a, 2020; Beerling et al., 2020).
View in article
Bragazza, L., Parisod, J., Buttler, A., Bardgett, R.D. (2013) Biogeochemical plant-soil microbe feedback in response to climate warming in peatlands. Nature Climate Change 3, 273–277. https://doi.org/10.1038/nclimate1781

Although peatlands cover only about 3 % of the continents (Xu et al., 2018), they store ∼10 % of all non-glacial freshwater and roughly 30 % of the land-based organic carbon (Mitra et al., 2005; Bragazza et al., 2013).
View in article
Dessert, C., Dupré, B., Gaillardet, J., François, L.M., Allègre, C.J. (2003) Basalt weathering laws and the impact of basalt weathering on the global carbon cycle. Chemical Geology 202, 257–273. https://doi.org/10.1016/j.chemgeo.2002.10.001

The natural weathering of basaltic and ultramafic rocks has been demonstrated to have a relatively large role in the drawdown of CO2 from the atmosphere (Dessert et al., 2003; Gislason et al., 2009; Taylor et al., 2021).
View in article
Gillman, G.P., Burkett, D.C., Coventry, R.J. (2002) Amending highly weathered soils with finely ground basalt rock. Applied Geochemistry 17, 987–1001. https://doi.org/10.1016/S0883-2927(02)00078-1

Enhanced weathering experiments to date have tested the addition of up to 400 t ha−1 yr−1 of crushed Ca-Mg silicate rocks to agricultural soils (Gillman et al., 2002; Amann et al., 2018; Haque et al., 2019b).
View in article
The grain size of the crushed rocks used in EW applications, if reported, is commonly less than 150 μm (Haque et al., 2019a; Gillman et al., 2002).
View in article
Gísladóttir, G., Erlendsson, E., Lal, R., Bigham, J. (2008) Erosional Effects on Terrestrial Resources over the last Millennium in Reykjanes, Southwest Iceland. Quaternary Research 73, 20–32. https://doi.org/10.1016/j.yqres.2009.09.007

The more frequently deposited windblown dust is finer grained than the tephra and intermingled with the soil organic carbon (see “Soil classification and soil evolution in Iceland” in Supplementary Information). Based on palaeoecologic research (Gísladóttir et al., 2008; Arnalds, 2015; Möckel et al., 2017), in the absence of volcanic dust input, the Histic/Gleyic Andosols of Southern Iceland would have developed into Histosols (Arnalds, 2008).
View in article
Gislason, S.R., Oelkers, E.H., Eiriksdottir, E.S., Kardjilov, M.I., Gisladottir, G., Sigfusson, B., Snorrason, A., Elefsen, S., Hardardottir, J., Torssander, P., Oskarsson, N. (2009) Direct evidence of the feedback between climate and weathering. Earth and Planetary Science Letters 277, 213–222. https://doi.org/10.1016/j.epsl.2008.10.018

The natural weathering of basaltic and ultramafic rocks has been demonstrated to have a relatively large role in the drawdown of CO2 from the atmosphere (Dessert et al., 2003; Gislason et al., 2009; Taylor et al., 2021).
View in article
Haque, F., Santos, R.M., Dutta, A., Thimmanagari, M., Chiang, Y.W. (2019a) Co-Benefits of Wollastonite Weathering in Agriculture: CO2 Sequestration and Promoted Plant Growth. ACS Omega 4, 1425–1433. https://doi.org/10.1021/acsomega.8b02477

To date, enhanced weathering field experiments have demonstrated improved crop vigour, organic and inorganic carbon storage and decreased N2O degassing (Haque et al., 2019a, 2020; Beerling et al., 2020).
View in article
The grain size of the crushed rocks used in EW applications, if reported, is commonly less than 150 μm (Haque et al., 2019a; Gillman et al., 2002).
View in article
Haque, F., Chiang, Y., Santos, R. (2019b) Alkaline Mineral Soil Amendment: A Climate Change ‘Stabilization Wedge’? Energies 12, 2299. https://doi.org/10.3390/en12122299

Enhanced weathering experiments to date have tested the addition of up to 400 t ha−1 yr−1 of crushed Ca-Mg silicate rocks to agricultural soils (Gillman et al., 2002; Amann et al., 2018; Haque et al., 2019b).
View in article
Haque, F., Santos, R.M., Chiang, Y.W. (2020) CO2 sequestration by wollastonite-amended agricultural soils – An Ontario field study. International Journal of Greenhouse Gas Control 97, 103017. https://doi.org/10.1016/j.ijggc.2020.103017

To date, enhanced weathering field experiments have demonstrated improved crop vigour, organic and inorganic carbon storage and decreased N2O degassing (Haque et al., 2019a, 2020; Beerling et al., 2020).
View in article
Our result of 0.17 ± 0.036 t ha−1 yr−1 of carbon drawdown from alkalinity export by enhanced rock weathering is within the range of the handful of large scale cropland EW studies of 0.0005–0.5 t C ha−1 yr−1 (Haque et al., 2020; Taylor et al., 2021; Larkin et al., 2022).
View in article
Hooijer, A., Silvius, M., Wösten, H., Page, S. (2006) PEAT-CO2, Assessment of CO2 emissions from drained peatlands in SE Asia. Delft Hydraulic Report Q3943.

Man made drainage and burning of peat areas worldwide releases 0.5–0.8 Gt C yr−1, which is equivalent to 5–8 % of global anthropogenic carbon emissions (Hooijer et al., 2006; Parish et al., 2008).
View in article
IPCC (2018) Global warming of 1.5°C. An IPCC Special Report on the impacts of global warming of 1.5°C above pre-industrial levels and related global greenhouse gas emission pathways, in the context of strengthening the global response to the threat of climate change, sustainable development, and efforts to eradicate poverty. Masson-Delmotte, V., Zhai, P., Pörtner, H.O., Roberts, D., Skea, J., Shukla, P.R., Pirani, A., Moufouma-Okia, W., Péan, C., Pidcock, R., Connors, S., Matthews, J.B.R., Chen, Y., Zhou, X., Gomis, M.I., Lonnoy, E., Maycock, T., Tignor, M., Waterfield, T. (Eds.) World Meteorological Organization, Geneva, Switzerland. https://www.ipcc.ch/site/assets/uploads/sites/2/2019/06/SR15_Full_Report_High_Res.pdf

Such observations have motivated several proposals to use these rocks to remove CO2 directly from the atmosphere through a process called Enhanced Weathering (EW) (Moosdorf et al., 2014; IPPC, 2018; Beerling et al., 2020).
View in article
Keller, N., Stefani, M., Einarsdóttir, S., Helgadóttir, A., Gudmundsson, J., Snorrason, A., Thorsson, J., Tinganelli, L. (2020) National Inventory Report - Emissions of greenhouse gases in Iceland from 1990 to 2018. The Environment Agency of Iceland, Reykjavík.

Carbon dioxide emission from the drainage of peat areas is estimated to be the largest anthropogenic source of CO2 emissions in Iceland (Keller et al., 2020).
View in article
Larkin, C.S., Andrews, M.G., Pearce, C.R., Yeong, K.L., Beerling, D.J., Bellamy, J., Benedick, S., Freckleton, R.P., Goring-Harford, H., Sadekar, S., James, R.H. (2022) Quantification of CO2 removal in a large-scale enhanced weathering field trial on an oil palm plantation in Sabah, Malaysia. Frontiers in Climate 4, 959229. https://doi.org/10.3389/fclim.2022.959229

Our result of 0.17 ± 0.036 t ha−1 yr−1 of carbon drawdown from alkalinity export by enhanced rock weathering is within the range of the handful of large scale cropland EW studies of 0.0005–0.5 t C ha−1 yr−1 (Haque et al., 2020; Taylor et al., 2021; Larkin et al., 2022).
View in article
Linke, T., Oelkers, E.H., Dideriksen, K., Möckel, S.C., Nilabh, S., Grandia, F., Gislason, S.R. (2024) The geochemical evolution of basalt Enhanced Rock Weathering systems quantified from a natural analogue. Geochimica et Cosmochimica Acta 370, 66–77. https://doi.org/10.1016/j.gca.2024.02.005

The first manuscript (Linke et al., 2024) reports the composition of fluids and solids collected over two field seasons to 1) quantify the saturation state of the primary and secondary mineral phases with respect to the soil solutions, 2) determine the processes controlling the mobility of heavy metals, and 3) assess the rate at which basalt dissolved in the soils.
View in article
Although, dissolution rates are commonly thought to be proportional to the fluid-mineral surface area, these rates are also influenced by fluid compositions, including approach to equilibrium and fluid flow paths in the soil column (Schott et al. 2009; Linke et al., 2024).
View in article
Liu, E.J., Cashman, K.V., Beckett, F.M., Witham, C.S., Leadbetter, S.J., Hort, M.C., Gudmudsson, S. (2014) Ash mists and brown snow: Remobilization of volcanic ash from recent Icelandic eruptions. Journal of Geophysical Research: Atmospheres 119, 9463–9480. https://doi.org/10.1002/2014JD021598

In contrast, the average size of basaltic Icelandic dust ranges from 10 to 62 μm (Arnalds et al., 2014; Liu et al., 2014).
View in article
Mahowald, N.M., Baker, A.R., Bergametti, G., Brooks, N., Duce, R.A., Jickells, T.D., Kubilay, N., Prospero, J.M., Tegen, I. (2005) Atmospheric global dust cycle and iron inputs to the ocean. Global Biogeochemical Cycles 19, GB4025. https://doi.org/10.1029/2004GB002402

This crushed rock flux is orders of magnitude higher than the average global desert dust deposition on Earth, which is estimated to be 0.5 t ha−1 yr−1 (Mahowald et al., 2005).
View in article
Mitra, S., Wassmann, R., Vlek, P.L.G. (2005) An appraisal of global wetland area and its organic carbon stock. Current Science 88, 25–35. http://www.jstor.org/stable/24110090

Although peatlands cover only about 3 % of the continents (Xu et al., 2018), they store ∼10 % of all non-glacial freshwater and roughly 30 % of the land-based organic carbon (Mitra et al., 2005; Bragazza et al., 2013).
View in article
Möckel, S.C., Erlendsson, E., Gísladóttir, G. (2017) Holocene environmental change and development of the nutrient budget of histosols in North Iceland. Plant and Soil 418, 437–457. https://doi.org/10.1007/s11104-017-3305-y

The more frequently deposited windblown dust is finer grained than the tephra and intermingled with the soil organic carbon (see “Soil classification and soil evolution in Iceland” in Supplementary Information). Based on palaeoecologic research (Gísladóttir et al., 2008; Arnalds, 2015; Möckel et al., 2017), in the absence of volcanic dust input, the Histic/Gleyic Andosols of Southern Iceland would have developed into Histosols (Arnalds, 2008).
View in article
Moosdorf, N., Renforth, P., Hartmann, J. (2014) Carbon dioxide efficiency of terrestrial enhanced weathering. Environmental Science & Technology 48, 4809–4816. https://doi.org/10.1021/es4052022

Such observations have motivated several proposals to use these rocks to remove CO2 directly from the atmosphere through a process called Enhanced Weathering (EW) (Moosdorf et al., 2014; IPPC, 2018; Beerling et al., 2020).
View in article
Parish, F., Sirin, A., Charman, D., Joosten, H., Minayeva, T., Silvius, M., Stringer, L. (2008) Assessment on Peatlands, Biodiversity and Climate Change: Main Report. Global Environment Centre, Kuala Lumpur and Wetlands International, Wageningen.

Man made drainage and burning of peat areas worldwide releases 0.5–0.8 Gt C yr−1, which is equivalent to 5–8 % of global anthropogenic carbon emissions (Hooijer et al., 2006; Parish et al., 2008).
View in article
Parkhurst, D.L., Appelo, C.A.J. (1999) User’s guide to PHREEQC (Version 2): A computer program for speciation, batch-reaction, one-dimensional transport, and inverse geochemical calculations. Water-Resources Investigations Report 99-4259, US Geological Survey, Denver. https://doi.org/10.3133/wri994259

The compositions of all fluid samples are provided in Table S-2 (Supplementary Information) and selected dissolved constituents are shown as a function of depth in Figure 2. The pH of the samples was recalculated using PHREEQC (Parkhurst and Appelo, 1999) to the in situ soil temperature of 7 °C.
View in article
Petersen, G.N., Berber, D. (2018) Soil temperature measurements in Iceland, status and future outlook vision (in Icelandic: Jarðvegshitamælingar á Íslandi. Staða núverandi kerfis og framtíðarsýn). Report of the Icelandic Meteorological Office (Skýrsla Veðurstofu Íslands), VÍ 2018-009, Reykjavík, Iceland. https://www.vedur.is/media/vedurstofan-utgafa-2018/VI_2018_009_rs.pdf

This is the average soil temperature at 76–260 cm depth during the summer months (Petersen and Gerber, 2018).
View in article
Schott, J., Pokrovsky, O.S., Oelkers, E.H. (2009) The link between mineral dissolution/precipitation kinetics and solution chemistry. Reviews in Mineralogy 70, 207–258. https://doi.org/10.2138/rmg.2009.70.6

Although, dissolution rates are commonly thought to be proportional to the fluid-mineral surface area, these rates are also influenced by fluid compositions, including approach to equilibrium and fluid flow paths in the soil column (Schott et al. 2009; Linke et al., 2024).
View in article
Shoji, S., Nanzyo, M., Dahlgren, R. (1995) Volcanic Ash Soils: Genesis, Properties and Utilization. Developments in Soil Science, Elsevier, Amsterdam.

The studied soil receives large amounts of air borne volcanic material during 1) explosive volcanic eruptions in the form of glassy volcanic ash fallout, and 2) dust storms (Shoji et al., 1995; Arnalds et al., 2016).
View in article
Stockmann, U., Padarian, J., McBratney, A., Minasny, B., de Brogniez, D., Montanarella, L., Hong, S.Y., Rawlins, B.G., Field, D.J. (2015) Global soil organic carbon assessment. Global Food Security 6, 9–16 https://doi.org/10.1016/j.gfs.2015.07.001

This conclusion, based on the alkalinity export from our studied soil, which contains more organic matter (12 % to >20 % C) than most soils globally (<5 % C; e.g., Stockmann et al., 2015), is nevertheless supported by other recent enhanced rock weathering studies.
View in article
Strefler, J., Amann, T., Bauer, N., Kriegler, E., Hartmann, J. (2018) Potential and costs of carbon dioxide removal by enhanced weathering of rocks. Environmental Research Letters 13, 034010. https://doi.org/10.1088/1748-9326/aaa9c4

Enhanced weathering involves amending soils with crushed fine grained, fast reacting Ca-Mg silicate rocks and minerals such as basalts and peridotites (Strefler et al., 2018).
View in article
Taylor, L.L., Driscoll, C.T., Groffman, P.M., Rau, G.H., Blum, J.D., Beerling, D.J. (2021) Increased carbon capture by a silicate-treated forested watershed affected by acid deposition. Biogeosciences 18, 169–188. https://doi.org/10.5194/bg-18-169-2021

The natural weathering of basaltic and ultramafic rocks has been demonstrated to have a relatively large role in the drawdown of CO2 from the atmosphere (Dessert et al., 2003; Gislason et al., 2009; Taylor et al., 2021).
View in article
Our result of 0.17 ± 0.036 t ha−1 yr−1 of carbon drawdown from alkalinity export by enhanced rock weathering is within the range of the handful of large scale cropland EW studies of 0.0005–0.5 t C ha−1 yr−1 (Haque et al., 2020; Taylor et al., 2021; Larkin et al., 2022).
View in article
These estimates are in agreement with previous studies (Taylor et al., 2021).
View in article
Xu, J., Morris, P.J., Liu, J., Holden, J. (2018) PEATMAP: Refining estimates of global peatland distribution based on a meta-analysis. Catena 160, 134–140. https://doi.org/10.1016/j.catena.2017.09.010

Although peatlands cover only about 3 % of the continents (Xu et al., 2018), they store ∼10 % of all non-glacial freshwater and roughly 30 % of the land-based organic carbon (Mitra et al., 2005; Bragazza et al., 2013).
View in article
top
Supplementary Information
The Supplementary Information includes:
- Soil Classification and Soil Evolution in Iceland
- Detailed Field Site Description
- Details of Field Sampling
- Analytical Methods
- Elemental Analysis
- Calculation of Alkalinity Creation and Export in Our Studied Soil
- Estimated Organic Carbon Storage Within the Studied Soil
- Effect of Basalt on Organic Carbon
- Tables S-1 to S-4
- Supplementary Information References
Download the Supplementary Information (PDF)
Figures
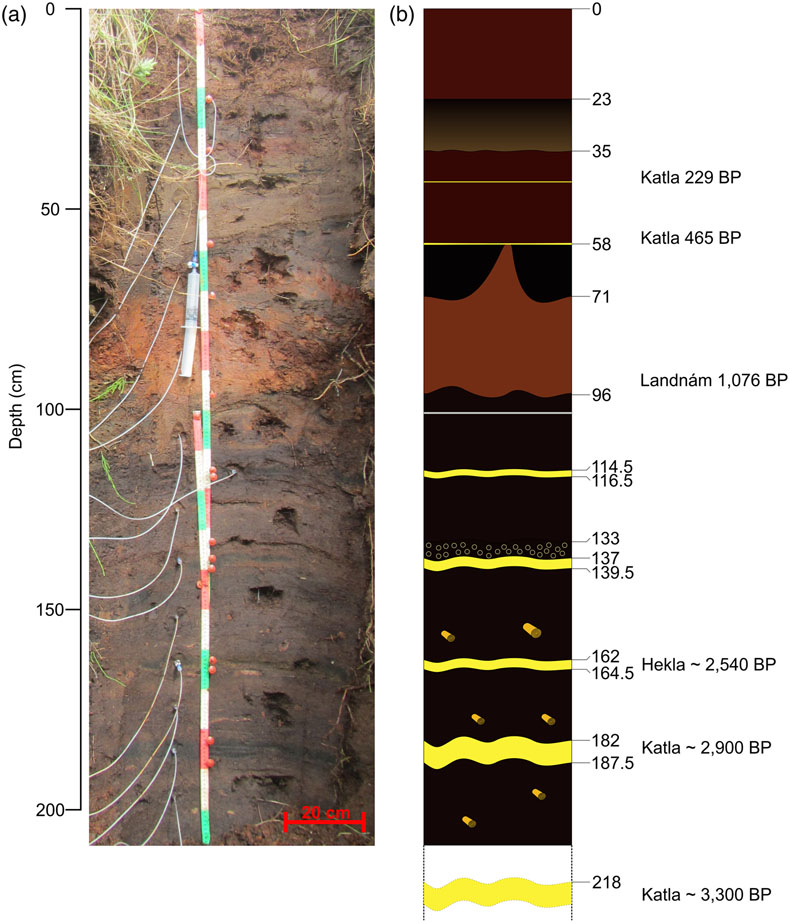
Figure 1 (a) Photograph of the studied soil system. The dark layers correspond to tephra originating from historic volcanic eruptions. (b) Schematic illustration of the soil profile with depths and ages (in years before present) of identified tephra layers shown in yellow for better visibility, the Landnám Layer that occurred around the time of the Icelandic settlement could not be clearly identified because of alteration. Plant remnants are visible in the lower part of the profile, indicating high organic content.
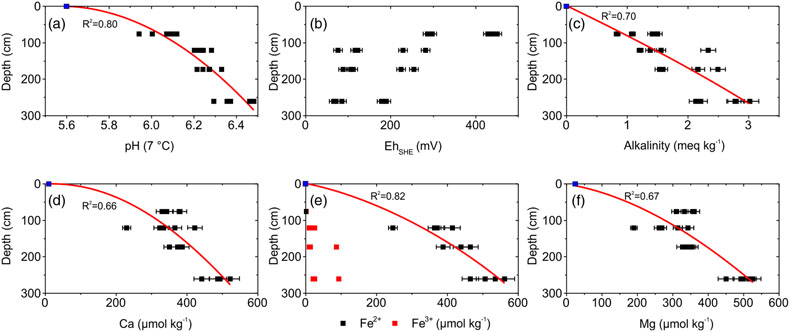
Figure 2 Measured soil water concentrations determined in the present study in all samples collected from May to November 2018, as a function of depth. The pH values are normalised to a 7 °C reference temperature and Eh values to a Standard Hydrogen Electrode. The black squares represent measured water concentrations, whereas the blue squares show the composition of rainwater. The error bars correspond to a ±5 % uncertainty on the measured concentrations; error bars do not appear if the uncertainty is smaller than the symbol size. The red curves in the figure show 2nd order polynomial fits of all the measured concentrations with the corresponding R2 values next to each curve.
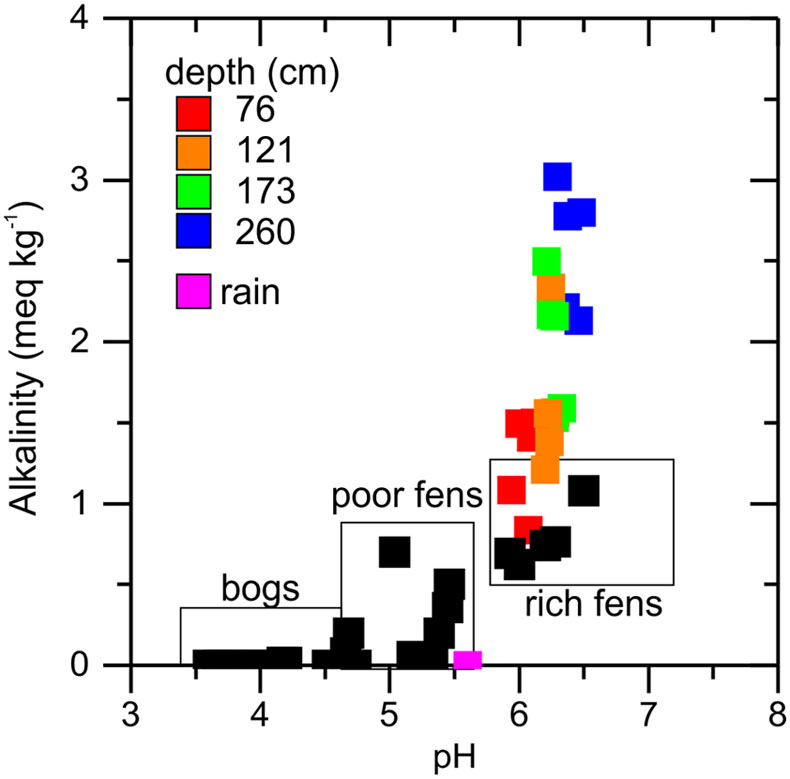
Figure 3 Comparison of pH and alkalinity of soil waters collected from our studied soil with similarly composed, but volcanic dust-free soils reported in other studies. The black symbols correspond to alkalinity values reported in the literature for Histosols from bogs, poor or rich fens located in non-volcanic regions and in the absence of carbonate bedrock. The black boxes around the black symbols represent the commonly reported pH-alkalinity ranges of bogs and fens respectively. The red, orange, green and blue symbols represent soil water samples measured in the present study at the depths indicated in the figure. The purple symbol shows the composition of rainwater at our field site. The sources and location of the literature data are provided in Table S-3 of the Supplementary Information.
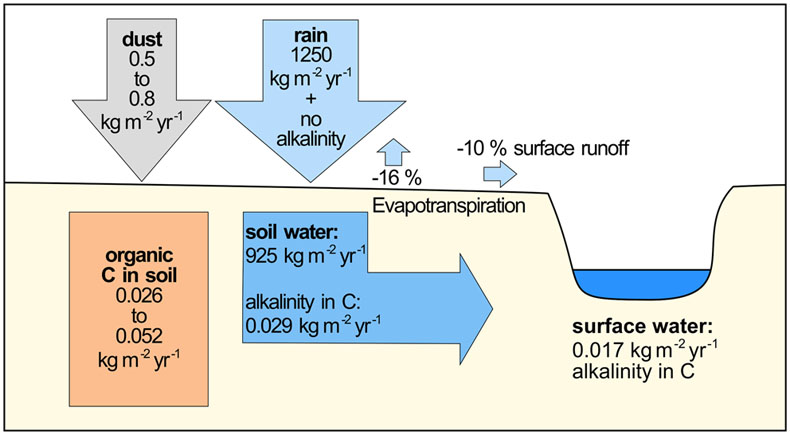
Figure 4 Schematic illustration of the processes drawing down CO2 at our field site. The site receives ∼1250 ± 200 kg m−2 yr−1 of rainfall. Of this rainfall 16 % is estimated to evaporate and 10 % is estimated to be lost to surface runoff. As the remaining 925 ± 150 kg m−2 yr−1 of water passes through the soil, its alkalinity increases on average from 0 to 2.59 ± 0.34 meq kg−1 at depth. Once these waters equilibrate with the atmosphere, this fluid oxidises and some CO2 is released such that the alkalinity decreases to 1.53 ± 0.2 meq kg−1 resulting in an annual export of 17 ± 3.6 g C per m2 soil surface area. At the same time 26–52 g m−2 yr−1 of C is drawn down from the atmosphere by organic carbon production and stored in the soil.