Spicule morphology impacts stable silicon isotopic composition of sponge archives
Affiliations | Corresponding Author | Cite as | Funding information- Share this article
-
Article views:917Cumulative count of HTML views and PDF downloads.
- Download Citation
- Rights & Permissions
top
Abstract
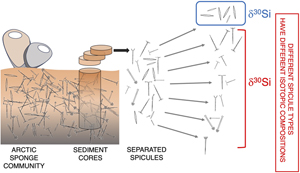
Figures
![]() Figure 1 (a) Remotely Operated Vehicle image of the sponge ground of the Schulz Bank summit (image courtesy of the University of Bergen). (b-c) Photographs of the subcores from BC1 and BC2. (d) Spicule morphology definitions used in this study. | ![]() Figure 2 Downcore δ30Si values from the different spicule types extracted from (a) BC1 and (b) BC2. Error bar shows 2 σ based on repeat measurements of reference materials. Each point represents the mean of repeat measurements of a sample aliquot (n = 2 or 3). (c) Assessment of true replicates of separate aliquots from a given horizon. Each symbol represents the mean of repeat measurements (n = 2 or 3, error bar 1 s.d.) of each of multiple aliquots (n = 2) of spicules extracted from the same horizon. | ![]() Figure 3 Box and whisker plots summarising downcore δ30Si data from (a) BC1 and (b) BC2 for the main spicule types present in the sediment. The notches represent the median values, the bottom and top edges of the box represent the 25 th and 75 th percentiles, and the whiskers encompass all data points not considered statistical outliers (outliers are shown by a red cross). | ![]() Figure 4 New spicule isotopic data from this study (grey hollow circles are non-Oxea Demosponge spicules; solid circles are Oxea spicules) plotted with existing field calibration of ambient DSi concentrations against (a) sponge spicule δ30Si values and (b) apparent isotopic fractionation (Δ30Si = δ30Sisponge − δ30Sisw), assuming ambient seawater δ30Sisw at the SB of +1.7 ‰ (Brzezinski et al., 2021). Blue symbols = demosponges without Cladorhizidae, red symbols = hexactinellids without hypersilicifiers. |
Figure 1 | Figure 2 | Figure 3 | Figure 4 |
top
Introduction
The global marine silicon cycle plays a critical role in the Earth’s climate system via carbon uptake and sequestration (Struyf et al., 2009
Struyf, E., Smis, A., Van Damme, S., Meire, P., Conley, D.J. (2009) The global biogeochemical silicon cycle. Silicon 1, 207–213. https://doi.org/10.1007/s12633-010-9035-x
; Tréguer et al., 2021Tréguer, P.J., Sutton, J.N., Brzezinski, M., Charette, M.A., Devries, T., Dutkiewicz, S., Ehlert, C., Hawkings, J., Leynaert, A., Liu, S.M. (2021) Reviews and syntheses: The biogeochemical cycle of silicon in the modern ocean. Biogeosciences 18, 1269–1289. https://doi.org/10.5194/bg-18-1269-2021
). Diatoms, a siliceous phytoplankton group common to most marine ecosystems, are one of the most important contributors to organic matter export production. As they form their cell walls from silica, diatoms have an absolute requirement for dissolved silicon (silicic acid, or DSi), and largely rely on upwelling of deeper waters to obtain the nutrients required for growth. As such, archives of past deep and bottom water DSi concentrations in addition to surface conditions are essential for understanding past ocean biogeochemistry and climate impacts (Ellwood et al., 2010Ellwood, M.J., Wille, M., Maher, W. (2010) Glacial silicic acid concentrations in the Southern Ocean. Science 330, 1088–1091. https://doi.org/10.1126/science.1194614
; Fontorbe et al., 2016Fontorbe, G., Frings, P.J., Christina, L., Hendry, K.R., Conley, D.J. (2016) A silicon depleted North Atlantic since the Palaeogene: Evidence from sponge and radiolarian silicon isotopes. Earth and Planetary Science Letters 453, 67–77. https://doi.org/10.1016/j.epsl.2016.08.006
; Sutton et al., 2018Sutton, J.N., André, L., Cardinal, D., Conley, D.J., De Souza, G.F., Dean, J., Dodd, J., Ehlert, C., Ellwood, M.J., Frings, P.J. (2018) A review of the stable isotope bio-geochemistry of the global silicon cycle and its associated trace elements. Frontiers in Earth Science 5, 112. https://doi.org/10.3389/feart.2017.00112
; Hendry et al., 2019Hendry, K.R., Cassarino, L., Bates, S.L., Culwick, T., Frost, M., Goodwin, C., Howell, K.L. (2019) Silicon isotopic systematics of deep-sea sponge grounds in the North Atlantic. Quaternary Science Reviews 210, 1–14. https://doi.org/10.1016/j.quascirev.2019.02.017
; Dumont et al., 2020Dumont, M., Pichevin, L., Geibert, W., Crosta, X., Michel, E., Moreton, S., Dobby, K., Ganeshram, R. (2020) The nature of deep overturning and reconfigurations of the silicon cycle across the last deglaciation. Nature Communications 11, 1534. https://doi.org/10.1038/s41467-020-15101-6
).Surface and subsurface silicon cycling processes can be reconstructed using the geochemistry of siliceous microfossils, such as diatoms and radiolarians, extracted from marine sediments (Hendry et al., 2014
Hendry, K.R., Robinson, L.F., McManus, J.F., Hays, J.D. (2014) Silicon isotopes indicate enhanced carbon export efficiency in the North Atlantic during deglaciation. Nature Communications 5, 3107. https://doi.org/10.1038/ncomms4107
; Abelmann et al., 2015Abelmann, A., Gersonde, R., Knorr, G., Zhang, X., Chapligin, B., Maier, E., Esper, O., Friedrichsen, H., Lohmann, G., Meyer, H. (2015) The seasonal sea-ice zone in the glacial Southern Ocean as a carbon sink. Nature Communications 6, 8136. https://doi.org/10.1038/ncomms9136
). In deeper waters benthic siliceous sponges create a skeleton of sponge spicules, which have the potential to act as archives of past bottom water conditions, providing a unique source of the critical information required to reconstruct past changes in DSi supply to the surface ocean from upwelling waters (De La Rocha, 2003De La Rocha, C.L. (2003) Silicon isotope fractionation by marine sponges and the reconstruction of the silicon isotope composition of ancient deep water. Geology 31, 423–426. https://doi.org/10.1130/0091-7613(2003)031<0423:SIFBMS>2.0.CO;2
; Łukowiak, 2020Łukowiak, M. (2020) Utilizing sponge spicules in taxonomic, ecological and environmental reconstructions: a review. PeerJ 8, e10601. https://doi.org/10.7717/peerj.10601
). Several field-based studies have shown that there is a non-linear relationship between the concentration of ambient DSi and both the isotopic composition (denoted by δ30Si) of spicules and apparent isotopic fractionation by sponges during growth that is apparently insensitive to temperature and other seawater properties (Hendry et al., 2010Hendry, K.R., Georg, R.B., Rickaby, R.E., Robinson, L.F., Halliday, A.N. (2010) Deep ocean nutrients during the Last Glacial Maximum deduced from sponge silicon isotopic compositions. Earth and Planetary Science Letters 292, 290–300. https://doi.org/10.1016/j.epsl.2010.02.005
; Wille et al., 2010Wille, M., Sutton, J., Ellwood, M.J., Sambridge, M., Maher, W., Eggins, S., Kelly, M. (2010) Silicon isotopic fractionation in marine sponges: A new model for understanding silicon isotopic variations in sponges. Earth and Planetary Science Letters 292, 281–289. https://doi.org/10.1016/j.epsl.2010.01.036
; Hendry and Robinson, 2012Hendry, K.R., Robinson, L.F. (2012) The relationship between silicon isotope fractionation in sponges and silicic acid concentration: Modern and core-top studies of biogenic opal. Geochimica et Cosmochimica Acta 81, 1–12. https://doi.org/10.1016/j.gca.2011.12.010
). At present, there is no evidence for any systematic difference in isotopic fractionation behaviour between the major groups of siliceous sponges, demosponges and hexactinellids, after differences in habitat and depth preference are taken into account (Cassarino et al., 2018Cassarino, L., Coath, C.D., Xavier, J.R., Hendry, K.R. (2018) Silicon isotopes of deep sea sponges: new insights into biomineralisation and skeletal structure. Biogeosciences 15, 6959–6977. https://doi.org/10.5194/bg-15-6959-2018
; Hendry et al., 2019Hendry, K.R., Cassarino, L., Bates, S.L., Culwick, T., Frost, M., Goodwin, C., Howell, K.L. (2019) Silicon isotopic systematics of deep-sea sponge grounds in the North Atlantic. Quaternary Science Reviews 210, 1–14. https://doi.org/10.1016/j.quascirev.2019.02.017
; Pack et al., 2023Pack, A., Bultmann, E.M., Tatzel, M., Reitner, J. (2023) A new method for silicon triple isotope analysis with application to siliceous sponge spicules. Geochemistry, Geophysics, Geosystems 24, e2023GC011243. https://doi.org/10.1029/2023GC011243
). However, there is considerable scatter in the spicule δ30Si-DSi calibration that remains poorly understood. Whilst some studies show good agreement in measurements of spicules taken from individual sponges and good agreement between laboratories (e.g., Hendry et al., 2011Hendry, K.R., Leng, M.J., Robinson, L.F., Sloane, H.J., Blusztjan, J., Rickaby, R.E., Georg, R.B., Halliday, A.N. (2011) Silicon isotopes in Antarctic sponges: an interlaboratory comparison. Antarctic Science 23, 34–42. https://doi.org/10.1017/S0954102010000593
), other studies highlight variation within an individual sponge, and between individuals, in both monospecific and more diverse communities (Hendry et al., 2019Hendry, K.R., Cassarino, L., Bates, S.L., Culwick, T., Frost, M., Goodwin, C., Howell, K.L. (2019) Silicon isotopic systematics of deep-sea sponge grounds in the North Atlantic. Quaternary Science Reviews 210, 1–14. https://doi.org/10.1016/j.quascirev.2019.02.017
). Genetically-controlled biomineralisation pathways appear to be strong drivers of spicule isotopic heterogeneity, with some derived sponge forms, including hypersilicified demosponges, glass sponges, and carnivorous sponges, exhibiting anomalous isotopic fractionation that deviates significantly from other sponges (Hendry et al., 2015Hendry, K., Swann, G.E., Leng, M.J., Sloane, H.J., Goodwin, C., Berman, J., Maldonado, M. (2015) Silica stable isotopes and silicification in a carnivorous sponge Asbestopluma sp. Biogeosciences 12, 3489–3498. https://doi.org/10.5194/bg-12-3489-2015
; Jochum et al., 2017Jochum, K., Schuessler, J., Wang, X.H., Stoll, B., Weis, U., Müller, W., Haug, G.H., Andreae, M., Froelich, P. (2017) Whole‐ocean changes in silica and Ge/Si ratios during the Last Deglacial deduced from long‐lived giant glass sponges. Geophysical Research Letters 44, 11,555–11,564. https://doi.org/10.1002/2017GL073897
; Cassarino et al., 2018Cassarino, L., Coath, C.D., Xavier, J.R., Hendry, K.R. (2018) Silicon isotopes of deep sea sponges: new insights into biomineralisation and skeletal structure. Biogeosciences 15, 6959–6977. https://doi.org/10.5194/bg-15-6959-2018
).Sponge spicules, when buried in sediments, can become part of the geological record forming a palaeo-archive that could potentially be used to reconstruct past bottom water DSi concentrations. In most cases, the sponge skeleton completely disintegrates and spicules in marine sediment cores are found as separate elements. The larger spicules (megascleres) are generally preserved better, and more readily isolated, than the smaller and more taxonomically diagnostic microscleres. As such, it can be highly challenging to determine from which sponge any given fossil spicule derived. Earlier palaeoceanographic studies simply extracted all spicule material for analysis (e.g., Hendry et al., 2010
Hendry, K.R., Georg, R.B., Rickaby, R.E., Robinson, L.F., Halliday, A.N. (2010) Deep ocean nutrients during the Last Glacial Maximum deduced from sponge silicon isotopic compositions. Earth and Planetary Science Letters 292, 290–300. https://doi.org/10.1016/j.epsl.2010.02.005
; Hendry et al., 2012Hendry, K.R., Robinson, L.F., Meredith, M.P., Mulitza, S., Chiessi, C.M., Arz, H. (2012) Abrupt changes in high-latitude nutrient supply to the Atlantic during the last glacial cycle. Geology 40, 123–126. https://doi.org/10.1130/G32779.1
), but more recent studies have taken the conservative approach of picking only one spicule type, usually selecting only the needle-like monaxonic spicules (e.g., Fontorbe et al., 2016Fontorbe, G., Frings, P.J., Christina, L., Hendry, K.R., Conley, D.J. (2016) A silicon depleted North Atlantic since the Palaeogene: Evidence from sponge and radiolarian silicon isotopes. Earth and Planetary Science Letters 453, 67–77. https://doi.org/10.1016/j.epsl.2016.08.006
; Fontorbe et al., 2017Fontorbe, G., Frings, P.J., De La Rocha, C.L., Hendry, K.R., Carstensen, J., Conley, D.J. (2017) Enrichment of dissolved silica in the deep equatorial Pacific during the Eocene‐Oligocene. Paleoceanography 32, 848–863. https://doi.org/10.1002/2017PA003090
). Developments in the use of secondary ion mass spectrometer (SIMS) methodologies have also allowed the targeting of individual spicules for isotopic analysis (e.g., Trower et al., 2021Trower, E.J., Strauss, J.V., Sperling, E.A., Fischer, W.W. (2021) Isotopic analyses of Ordovician–Silurian siliceous skeletons indicate silica‐depleted Paleozoic oceans. Geobiology 19, 460–472. https://doi.org/10.1111/gbi.12449
). However, to date, there has been no systematic study into the impact of spicule type on δ30Si records and – so – palaeoceanographic reconstructions.In this study, we address this gap using spicules extracted from box cores collected from a sponge ground in the Arctic Ocean. We ask the questions: do spicules of a given morphology picked from a given sediment horizon have consistent isotopic compositions? Do spicules of a given morphology picked from nearby sediment cores have consistent isotopic compositions? Is there a significant offset between different spicule morphologies picked from a given sediment horizon?
top
Methods
Box cores were collected from the Schulz Bank (SB) aboard the R/V G.O. Sars expedition GS2016109A in 2016. The summit of the SB is at approximately 560–600 m water depth, with a thick (∼20 cm) sponge spicule mat characterising the upper reaches of the slope and summit (Meyer et al., 2023
Meyer, H.K., Davies, A., Roberts, E.M., Xavier, J.R., Ribeiro, P., Glenner, H., Birkely, S.-R., Rapp, H. (2023) Beyond the tip of the seamount: Distinct megabenthic communities found beyond the charismatic summit sponge ground on an arctic seamount (Schulz Bank, Arctic Mid-Ocean Ridge). Deep Sea Research Part I: Oceanographic Research Papers 191, 103920. https://doi.org/10.1016/j.dsr.2022.103920
). The oceanographic conditions around the SB are beneficial to sponge growth, with relatively nutrient-rich bottom waters frequently flushed with high oxygen, warm Arctic Intermediate Waters (Hanz et al., 2021Hanz, U., Roberts, E.M., Duineveld, G., Davies, A., van Haren, H., Rapp, H.T., Reichart, G.J., Mienis, F. (2021) Long‐term observations reveal environmental conditions and food supply mechanisms at an Arctic deep‐sea sponge ground. Journal of Geophysical Research: Oceans 126, e2020JC016776. https://doi.org/10.1029/2020JC016776
). Mean nutrient concentrations from between 600 and 800 m on SB are 7.56 mM (2 s.d. 0.63), 13.97 mM (2 s.d. 0.30), and 0.95 mM (2 s.d. 0.01) for DSi, nitrate and phosphate respectively (full details in Roberts et al., 2018Roberts, E., Mienis, F., Rapp, H.T., Hanz, U., Meyer, H., Davies, A. (2018) Oceanographic setting and short-timescale environmental variability at an Arctic seamount sponge ground. Deep Sea Research Part I: Oceanographic Research Papers 138, 98–113. https://doi.org/10.1016/j.dsr.2018.06.007
). A high organic particulate matter supply is maintained by strong diurnal currents, which also act to prevent sedimentation (Roberts et al., 2018Roberts, E., Mienis, F., Rapp, H.T., Hanz, U., Meyer, H., Davies, A. (2018) Oceanographic setting and short-timescale environmental variability at an Arctic seamount sponge ground. Deep Sea Research Part I: Oceanographic Research Papers 138, 98–113. https://doi.org/10.1016/j.dsr.2018.06.007
).These conditions support a dense benthic community of sponges, or ‘sponge ground’ on the SB (Fig. 1a). The SB sponge ground is diverse including several species of demosponges and hexactinellids (see Supplementary Information).
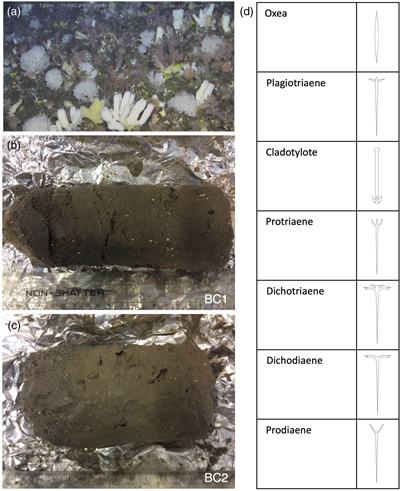
Figure 1 (a) Remotely Operated Vehicle image of the sponge ground of the Schulz Bank summit (image courtesy of the University of Bergen). (b-c) Photographs of the subcores from BC1 and BC2. (d) Spicule morphology definitions used in this study.
Box cores BC1 and BC2 were collected from 73.81° N, 7.51° E, from 773 m and 765 m water depth respectively, less than 5 m apart on the seafloor. BC1 and BC2 were subsampled using plastic tubes, which were extruded and wrapped in clean foil and frozen for transport (Fig. 1b,c). Although age models do not exist for these subcores, the age model based on radiocarbon age dating of a nearby core GS2017-47 collected during an expedition in 2017 would indicate that the top 16 cm as subsampled in this study should be approximately 6,000 years old (unpublished data).
The sediments were thawed in the UK, photographed (Fig. 1b,c) and sectioned into 1 cm slices. These slices were air dried in a cool oven (40 °C), washed and sieved at 63 μm, and then re-dried. Monoaxonic spicules were hand picked from the sediments using a very fine brush under a stereomicroscope and categorised into different spicule morphologies (see Fig. 1d). The categories represented are: Oxea, Plagiotriaene, Cladotylote, Dichotriaene, Protriaene, Dichodiaene (rare), and Prodiaene (rare) (Łukowiak et al., 2022
Łukowiak, M., Van Soest, R., Klautau, M., Pérez, T., Pisera, A., Tabachnick, K. (2022) The terminology of sponge spicules. Journal of Morphology 283, 1517–1545. https://doi.org/10.1002/jmor.21520
). It should be noted that these spicule morphologies are not diagnostic of sponge species and may be present across different taxonomic groups within classes of demospongiae (including carnivorous sponges). One sample of Pentactine spicules (from hexactinellid sponges) was recoverable from BC1.The spicules (3 to 10 spicules per replicate, depending on size and availability) were cleaned for organic matter by heating for 1 hr at 80 °C in 30 % reagent grade hydrogen peroxide before being rinsed in 18 MΩ cm Milli-Q. The samples were dried down in concentrated in house distilled nitric acid (HNO3) at 120 °C, and then dissolved in 1 ml 0.4 M sodium hydroxide (Analar) at 100 °C for 24–72 hours. The solutions were acidified with 50 μl 8 N HNO3, and diluted with 1 ml Milli-Q. All samples and standards were chemically purified using cation exchange resin (Bio-Rad AG50W-X12) following published protocols, which have shown quantitative Si yield and cation retention under neutral or acidic conditions (e.g., Georg et al., 2006
Georg, R., Reynolds, B.C., Frank, M., Halliday, A.N. (2006) New sample preparation techniques for the determination of Si isotopic compositions using MC-ICPMS. Chemical Geology 235, 95–104. https://doi.org/10.1016/j.chemgeo.2006.06.006
; Zambardi and Poitrasson, 2011Zambardi, T., Poitrasson, F. (2011) Precise determination of silicon isotopes in silicate rock reference materials by MC‐ICP‐MS. Geostandards and Geoanalytical Research 35, 89–99. https://doi.org/10.1111/j.1751-908X.2010.00067.x
; Savage and Moynier, 2013Savage, P.S., Moynier, F. (2013) Silicon isotopic variation in enstatite meteorites: clues to their origin and Earth-forming material. Earth and Planetary Science Letters 361, 487–496. https://doi.org/10.1016/j.epsl.2012.11.016
). Before analysis, the solutions were doped with a magnesium solution. The samples were analysed within 72 hours of purification for 28Si, 29Si, 30Si, 24Mg, 25Mg, and 26Mg using a Multi-Collector Inductively Coupled Plasma Mass Spectrometer (Thermo Neptune MC-ICP-MS) in medium resolution mode. Sample blank corrections and Mg isotope mass bias corrections were carried out offline, before being normalised to NBS28 (RM8546) (Hendry et al., 2015Hendry, K., Swann, G.E., Leng, M.J., Sloane, H.J., Goodwin, C., Berman, J., Maldonado, M. (2015) Silica stable isotopes and silicification in a carnivorous sponge Asbestopluma sp. Biogeosciences 12, 3489–3498. https://doi.org/10.5194/bg-12-3489-2015
). A three isotope plot shows that all analyses fall on a mass-dependent δ29Si and δ30Si line, with a gradient of 0.510 ± 0.003, within the range of equilibrium or kinetic fractionation (Reynolds et al., 2007Reynolds, B.C., Aggarwal, J., Andre, L., Baxter, D., Beucher, C., Brzezinski, M.A., Engström, E., Georg, R.B., Land, M., Leng, M.J. (2007) An inter-laboratory comparison of Si isotope reference materials. Journal of Analytical Atomic Spectrometry 22, 561–568. https://doi.org/10.1039/B616755A
). Repeat measurements of reference standards Diatomite and LMG08 (sponge material) reveal a long term δ30Si reproducibility of 1.24 ± 0.11 ‰ (n = 64) and −3.46 ± 0.12 ‰ (n = 65) respectively (2 s.d.), within published ranges (Reynolds et al., 2007Reynolds, B.C., Aggarwal, J., Andre, L., Baxter, D., Beucher, C., Brzezinski, M.A., Engström, E., Georg, R.B., Land, M., Leng, M.J. (2007) An inter-laboratory comparison of Si isotope reference materials. Journal of Analytical Atomic Spectrometry 22, 561–568. https://doi.org/10.1039/B616755A
; Hendry et al., 2011Hendry, K.R., Leng, M.J., Robinson, L.F., Sloane, H.J., Blusztjan, J., Rickaby, R.E., Georg, R.B., Halliday, A.N. (2011) Silicon isotopes in Antarctic sponges: an interlaboratory comparison. Antarctic Science 23, 34–42. https://doi.org/10.1017/S0954102010000593
). Each sample aliquot was measured at least twice and most were measured in triplicate. When possible, duplicate analyses were carried out on true replicates (i.e. analyses of separate aliquots of spicules that were picked from the same horizon). Statistical analyses (ANOVA and t-tests) were carried out using MATLAB R2021b for the more abundant spicule types, after testing for normality using an Anderson-Darling test (α = 0.05).top
Results
Oxea spicule δ30Si compositions ranged from −1.47 to −2.05 ‰. The other spicule types exhibited similar ranges, from −0.94 to −1.76 ‰ (Fig. 2a,b). The one sample of hexactinellid Pentactine spicules from BC1 (10–11 cm depth) had an isotopic composition of −1.11‰. The mean difference between δ30Si values of the true replicates was 0.14 ‰, with a maximum of 0.42 ‰ (Fig. 2c).
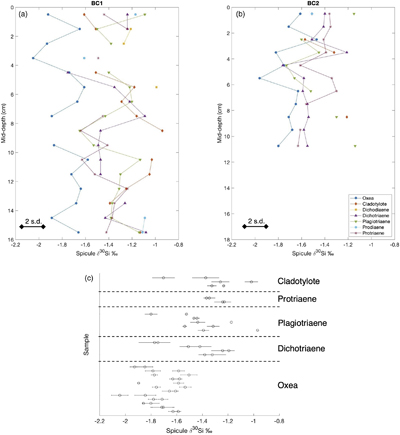
Figure 2 Downcore δ30Si values from the different spicule types extracted from (a) BC1 and (b) BC2. Error bar shows 2 σ based on repeat measurements of reference materials. Each point represents the mean of repeat measurements of a sample aliquot (n = 2 or 3). (c) Assessment of true replicates of separate aliquots from a given horizon. Each symbol represents the mean of repeat measurements (n = 2 or 3, error bar 1 s.d.) of each of multiple aliquots (n = 2) of spicules extracted from the same horizon.
Within each core, there was a significant difference between the mean isotopic composition of the different spicule morphologies downcore (for BC1, F -statistic= 24.77, d.f. = 3, p << 0.001; for BC2, F -statistic= 6.39, d.f. = 3, p = 0.001), with post hoc analysis indicating that the Oxea spicules were significantly different from the other spicule morphologies analysed. There was no significant difference for either core between the isotopic compositions of the Plagiotriaene, Dichotriaene, and Protriaene spicule morphologies (Fig. 3).
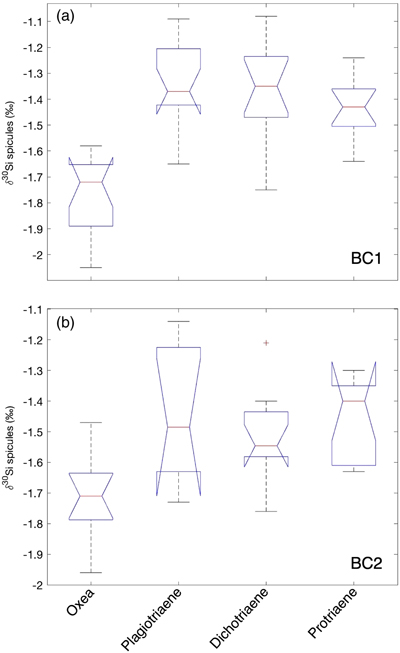
Figure 3 Box and whisker plots summarising downcore δ30Si data from (a) BC1 and (b) BC2 for the main spicule types present in the sediment. The notches represent the median values, the bottom and top edges of the box represent the 25 th and 75 th percentiles, and the whiskers encompass all data points not considered statistical outliers (outliers are shown by a red cross).
There was no significant difference between the mean downcore isotopic composition of same spicule morphologies between BC1 and BC2, except for a weak difference between the Dichotriaene spicules (p = 0.025). Note that there is an inherent caveat in taking averages of δ30Si values downcore, in that changes in environmental parameters through time could influence the spicule geochemical compositions.
top
Discussion
Comparison between Schulz Bank and other North Atlantic sponge grounds. To put our findings in a broader context, we can compare these findings from sedimentary spicules to field studies investigating stable silicon isotopic compositions of individual intact sponges. The SB study site is bathed in water with a similar DSi concentration to North Atlantic Geodia sponge aggregations at Orphan Knoll in the Labrador Sea and coastal Greenland (Hendry et al., 2019
Hendry, K.R., Cassarino, L., Bates, S.L., Culwick, T., Frost, M., Goodwin, C., Howell, K.L. (2019) Silicon isotopic systematics of deep-sea sponge grounds in the North Atlantic. Quaternary Science Reviews 210, 1–14. https://doi.org/10.1016/j.quascirev.2019.02.017
). The δ30Si values from different species of Geodia were assessed from these locations by taking subsamples from individual specimens, likely comprising a mixture of different spicule morphology types. The range of δ30Si values between the specimens was similar (−1.0 to −2.4 ‰) to the range from the differentiated sedimentary spicules measured in this study. Our observations show that spicule morphology likely contributes to variation in δ30Si between individuals of similar species, but other factors including localised differences in environmental conditions and growth rate will also play a role (Hendry et al., 2019Hendry, K.R., Cassarino, L., Bates, S.L., Culwick, T., Frost, M., Goodwin, C., Howell, K.L. (2019) Silicon isotopic systematics of deep-sea sponge grounds in the North Atlantic. Quaternary Science Reviews 210, 1–14. https://doi.org/10.1016/j.quascirev.2019.02.017
).Impact of spicule morphology on DSi concentration reconstructions. Here, we discuss the differences in δ30Si values obtained between spicule morphologies within and between the nearby box cores to address three key questions surrounding the impact of spicule form on palaeoceanographic archives.
1. Do spicules of a given morphology picked from a given sediment horizon have consistent isotopic compositions? The analyses of true replicates indicates that there is some variability in δ30Si within a given horizon for a particular spicule morphology. No differences were observed between column replicates (one aliquot of dissolved spicule passed through two columns), showing that the variability was not caused by sample processing. The mean difference between true duplicates was 0.14 ‰ – similar to the long term reproducibility based on repeat measurements of reference materials – and is often considerably less. However, there were some samples where there were larger differences between duplicate δ30Si measurements, up to 0.3 to 0.4 ‰, indicating significant environmental heterogeneity. There does not appear to be any relationship between duplicate offset and spicule type, indicating no clear link between isotopic variability and biomineralisation. Instead, these offsets could be due to recycling of silica (i.e. partial dissolution effects), localised differences in environmental conditions, or spicule growth rate (Hendry et al., 2019
Hendry, K.R., Cassarino, L., Bates, S.L., Culwick, T., Frost, M., Goodwin, C., Howell, K.L. (2019) Silicon isotopic systematics of deep-sea sponge grounds in the North Atlantic. Quaternary Science Reviews 210, 1–14. https://doi.org/10.1016/j.quascirev.2019.02.017
).2. Do spicules of a given morphology picked from nearby sediment cores have consistent isotopic compositions? Our findings indicate that spicules of a given morphology extracted from the two sediment cores do not have statistically different δ30Si compositions, and so should yield consistent [DSi] reconstructions. It is possible that there are differences in fossil age between spicules from the same 1 cm slice horizon in different cores due to small scale heterogeneities in sedimentation rate. However, given the assumed age of the core, there is only likely a 6,000 year age difference between the core top and 16 cm depth, and no large scale shifts in Arctic Ocean circulation that would have influenced bottom water conditions and nutrient dynamics are likely over this timescale (Rasmussen et al., 2014
Rasmussen, T.L., Thomsen, E., Skirbekk, K., Ślubowska-Woldengen, M., Kristensen, D.K., Koç, N. (2014) Spatial and temporal distribution of Holocene temperature maxima in the northern Nordic seas: interplay of Atlantic-, Arctic-and polar water masses. Quaternary Science Reviews 92, 280–291. https://doi.org/10.1016/j.quascirev.2013.10.034
).3. Is there a significant offset between different spicule morphologies picked from a given sediment horizon? Our analyses of different spicule morphologies extracted from the same core horizons indicated that the δ30Si composition of sponge silica, used to reconstruct past bottom water DSi, depends on the spicules chosen during the picking process. Oxea spicules could be a potential target for palaeoceanographic research as they are commonly found, are often large and so provide a useful amount of silica for analysis, and are readily identified as simple double-pointed needles. However, our findings reveal a significant offset between Oxea spicules and tetraxonic spicules analysed (common forms being Plagiotriaene, Dichotriaene, and Protriaene). There was no significant difference downcore between Plagiotriaene, Dichotriaene, and Protriaene spicules. In other words, the Oxea spicules form the outlier, yielding significantly lighter isotopic compositions than tetraxonic spicules, potentially reflecting an impact of biomineralisation processes, differential preservation, or redistribution by currents. When the shallow core spicule data are added to the δ30Si-DSi calibration plot (Fig. 4a), it is apparent that Oxea plot towards the isotopically lighter region of the observed scatter, with the other spicules falling more in the mid-range of the variability seen in field studies. This observation is robust even when taking into consideration any possible bias due to the isotopic composition of ambient seawater (Fig. 4b). Our results could imply that using Oxea could bias [DSi] reconstructions towards higher values. To test this possibility, we have substituted the range in spicule compositions into Equation 1 (adapted from Hendry et al., 2019
Hendry, K.R., Cassarino, L., Bates, S.L., Culwick, T., Frost, M., Goodwin, C., Howell, K.L. (2019) Silicon isotopic systematics of deep-sea sponge grounds in the North Atlantic. Quaternary Science Reviews 210, 1–14. https://doi.org/10.1016/j.quascirev.2019.02.017
):Eq. 1

where we have assumed the bottom water isotopic composition, δ30Si DSi, to be +1.7 ‰, similar to the deep Central Arctic Basin (Brzezinski et al., 2021
Brzezinski, M.A., Closset, I., Jones, J.L., De Souza, G.F., Maden, C. (2021) New constraints on the physical and biological controls on the silicon isotopic composition of the Arctic Ocean. Frontiers in Marine Science 8, 699762. https://doi.org/10.3389/fmars.2021.699762
). Reconstructed [DSi] for Oxea ranged from 12 to 25 mM, with a mean of 16 mM, whereas the range for the other spicules was 7 to 17 mM (mean 11 mM), matching better with modern [DSi] observations (mean value approximately 8 mM; Roberts et al., 2018Roberts, E., Mienis, F., Rapp, H.T., Hanz, U., Meyer, H., Davies, A. (2018) Oceanographic setting and short-timescale environmental variability at an Arctic seamount sponge ground. Deep Sea Research Part I: Oceanographic Research Papers 138, 98–113. https://doi.org/10.1016/j.dsr.2018.06.007
). As such, our findings suggest that Oxea may not be the best spicule morphology to select when using the existing field-based calibration, or that a new calibration using core top Oxea spicules is required.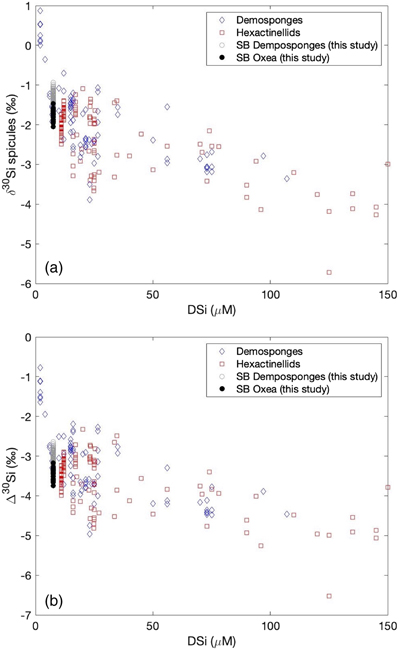
Figure 4 New spicule isotopic data from this study (grey hollow circles are non-Oxea Demosponge spicules; solid circles are Oxea spicules) plotted with existing field calibration of ambient DSi concentrations against (a) sponge spicule δ30Si values and (b) apparent isotopic fractionation (Δ30Si = δ30Sisponge − δ30Sisw), assuming ambient seawater δ30Sisw at the SB of +1.7 ‰ (Brzezinski et al., 2021
Brzezinski, M.A., Closset, I., Jones, J.L., De Souza, G.F., Maden, C. (2021) New constraints on the physical and biological controls on the silicon isotopic composition of the Arctic Ocean. Frontiers in Marine Science 8, 699762. https://doi.org/10.3389/fmars.2021.699762
). Blue symbols = demosponges without Cladorhizidae, red symbols = hexactinellids without hypersilicifiers.top
Synthesis
We have presented a systematic study of core top and downcore δ30Si measurements from different sponge spicule types extracted from box core material. Mean δ30Si compositions of the spicules of a given morphology in the top ∼16 cm were invariant with depth and largely did not show any significant differences between the subcores. Our results revealed a difference between spicule types extracted from a given sediment horizon, and significant differences between morphologies in the downcore mean δ30Si values. Our new findings imply that picking a single spicule type is best practice for palaeoceanographic applications of sponge archives, and the choice of Oxea spicules could bias these reconstructions towards high DSi concentrations.
top
Acknowledgements
This research has been performed in the scope of the SponGES project, which received funding from the European Union’s Horizon 2020 research and innovation programme under grant agreement No. 679849. KH and HP were also funded by ERC Starting Grant ICY-LAB (ERC-2015-STG grant agreement number 678371). JRX research is further supported by national funds through FCT Foundation for Science and Technology within the scope of UIDB/04423/2020, UIDP/04423/2020 and CEECIND/00577/2018. The authors would like to thank Hans Tore Rapp, and the captain, crew and scientists on board the R/V G.O. Sars. Thanks also to Stephanie Firth for assistance with sediment washing and spicule picking in the laboratory. Thanks to three anonymous reviewers for constructive comments during the review process. All data are available at https://doi.org/10.1594/PANGAEA.965455.
Editor: Claudine Stirling
top
References
Abelmann, A., Gersonde, R., Knorr, G., Zhang, X., Chapligin, B., Maier, E., Esper, O., Friedrichsen, H., Lohmann, G., Meyer, H. (2015) The seasonal sea-ice zone in the glacial Southern Ocean as a carbon sink. Nature Communications 6, 8136. https://doi.org/10.1038/ncomms9136

Surface and subsurface silicon cycling processes can be reconstructed using the geochemistry of siliceous microfossils, such as diatoms and radiolarians, extracted from marine sediments (Hendry et al., 2014; Abelmann et al., 2015).
View in article
Brzezinski, M.A., Closset, I., Jones, J.L., De Souza, G.F., Maden, C. (2021) New constraints on the physical and biological controls on the silicon isotopic composition of the Arctic Ocean. Frontiers in Marine Science 8, 699762. https://doi.org/10.3389/fmars.2021.699762

To test this possibility, we have substituted the range in spicule compositions into Equation 1 (adapted from Hendry et al., 2019):
Eq. 1
where we have assumed the bottom water isotopic composition, δ30Si DSi, to be +1.7 ‰, similar to the deep Central Arctic Basin (Brzezinski et al., 2021).
View in article
New spicule isotopic data from this study (grey hollow circles are non-Oxea Demosponge spicules; solid circles are Oxea spicules) plotted with existing field calibration of ambient DSi concentrations against (a) sponge spicule δ30Si values and (b) apparent isotopic fractionation (Δ30Si = δ30Sisponge − δ30Sisw), assuming ambient seawater δ30Sisw at the SB of +1.7 ‰ (Brzezinski et al., 2021).
View in article
Cassarino, L., Coath, C.D., Xavier, J.R., Hendry, K.R. (2018) Silicon isotopes of deep sea sponges: new insights into biomineralisation and skeletal structure. Biogeosciences 15, 6959–6977. https://doi.org/10.5194/bg-15-6959-2018

At present, there is no evidence for any systematic difference in isotopic fractionation behaviour between the major groups of siliceous sponges, demosponges and hexactinellids, after differences in habitat and depth preference are taken into account (Cassarino et al., 2018; Hendry et al., 2019; Pack et al., 2023).
View in article
Genetically-controlled biomineralisation pathways appear to be strong drivers of spicule isotopic heterogeneity, with some derived sponge forms, including hypersilicified demosponges, glass sponges, and carnivorous sponges, exhibiting anomalous isotopic fractionation that deviates significantly from other sponges (Hendry et al., 2015; Jochum et al., 2017; Cassarino et al., 2018).
View in article
De La Rocha, C.L. (2003) Silicon isotope fractionation by marine sponges and the reconstruction of the silicon isotope composition of ancient deep water. Geology 31, 423–426. https://doi.org/10.1130/0091-7613(2003)031<0423:SIFBMS>2.0.CO;2

In deeper waters benthic siliceous sponges create a skeleton of sponge spicules, which have the potential to act as archives of past bottom water conditions, providing a unique source of the critical information required to reconstruct past changes in DSi supply to the surface ocean from upwelling waters (De La Rocha, 2003; Łukowiak, 2020).
View in article
Dumont, M., Pichevin, L., Geibert, W., Crosta, X., Michel, E., Moreton, S., Dobby, K., Ganeshram, R. (2020) The nature of deep overturning and reconfigurations of the silicon cycle across the last deglaciation. Nature Communications 11, 1534. https://doi.org/10.1038/s41467-020-15101-6

As such, archives of past deep and bottom water DSi concentrations in addition to surface conditions are essential for understanding past ocean biogeochemistry and climate impacts (Ellwood et al., 2010; Fontorbe et al., 2016; Sutton et al., 2018; Hendry et al., 2019; Dumont et al., 2020).
View in article
Ellwood, M.J., Wille, M., Maher, W. (2010) Glacial silicic acid concentrations in the Southern Ocean. Science 330, 1088–1091. https://doi.org/10.1126/science.1194614

As such, archives of past deep and bottom water DSi concentrations in addition to surface conditions are essential for understanding past ocean biogeochemistry and climate impacts (Ellwood et al., 2010; Fontorbe et al., 2016; Sutton et al., 2018; Hendry et al., 2019; Dumont et al., 2020).
View in article
Fontorbe, G., Frings, P.J., Christina, L., Hendry, K.R., Conley, D.J. (2016) A silicon depleted North Atlantic since the Palaeogene: Evidence from sponge and radiolarian silicon isotopes. Earth and Planetary Science Letters 453, 67–77. https://doi.org/10.1016/j.epsl.2016.08.006

As such, archives of past deep and bottom water DSi concentrations in addition to surface conditions are essential for understanding past ocean biogeochemistry and climate impacts (Ellwood et al., 2010; Fontorbe et al., 2016; Sutton et al., 2018; Hendry et al., 2019; Dumont et al., 2020).
View in article
Earlier palaeoceanographic studies simply extracted all spicule material for analysis (e.g., Hendry et al., 2010; Hendry et al., 2012), but more recent studies have taken the conservative approach of picking only one spicule type, usually selecting only the needle-like monaxonic spicules (e.g., Fontorbe et al., 2016; Fontorbe et al., 2017).
View in article
Fontorbe, G., Frings, P.J., De La Rocha, C.L., Hendry, K.R., Carstensen, J., Conley, D.J. (2017) Enrichment of dissolved silica in the deep equatorial Pacific during the Eocene‐Oligocene. Paleoceanography 32, 848–863. https://doi.org/10.1002/2017PA003090

Earlier palaeoceanographic studies simply extracted all spicule material for analysis (e.g., Hendry et al., 2010; Hendry et al., 2012), but more recent studies have taken the conservative approach of picking only one spicule type, usually selecting only the needle-like monaxonic spicules (e.g., Fontorbe et al., 2016; Fontorbe et al., 2017).
View in article
Georg, R., Reynolds, B.C., Frank, M., Halliday, A.N. (2006) New sample preparation techniques for the determination of Si isotopic compositions using MC-ICPMS. Chemical Geology 235, 95–104. https://doi.org/10.1016/j.chemgeo.2006.06.006

All samples and standards were chemically purified using cation exchange resin (Bio-Rad AG50W-X12) following published protocols, which have shown quantitative Si yield and cation retention under neutral or acidic conditions (e.g., Georg et al., 2006; Zambardi and Poitrasson, 2011; Savage and Moynier, 2013).
View in article
Hanz, U., Roberts, E.M., Duineveld, G., Davies, A., van Haren, H., Rapp, H.T., Reichart, G.J., Mienis, F. (2021) Long‐term observations reveal environmental conditions and food supply mechanisms at an Arctic deep‐sea sponge ground. Journal of Geophysical Research: Oceans 126, e2020JC016776. https://doi.org/10.1029/2020JC016776

The oceanographic conditions around the SB are beneficial to sponge growth, with relatively nutrient-rich bottom waters frequently flushed with high oxygen, warm Arctic Intermediate Waters (Hanz et al., 2021).
View in article
Hendry, K.R., Georg, R.B., Rickaby, R.E., Robinson, L.F., Halliday, A.N. (2010) Deep ocean nutrients during the Last Glacial Maximum deduced from sponge silicon isotopic compositions. Earth and Planetary Science Letters 292, 290–300. https://doi.org/10.1016/j.epsl.2010.02.005

Several field-based studies have shown that there is a non-linear relationship between the concentration of ambient DSi and both the isotopic composition (denoted by δ30Si) of spicules and apparent isotopic fractionation by sponges during growth that is apparently insensitive to temperature and other seawater properties (Hendry et al., 2010; Wille et al., 2010; Hendry and Robinson, 2012).
View in article
Earlier palaeoceanographic studies simply extracted all spicule material for analysis (e.g., Hendry et al., 2010; Hendry et al., 2012), but more recent studies have taken the conservative approach of picking only one spicule type, usually selecting only the needle-like monaxonic spicules (e.g., Fontorbe et al., 2016; Fontorbe et al., 2017).
View in article
Hendry, K.R., Leng, M.J., Robinson, L.F., Sloane, H.J., Blusztjan, J., Rickaby, R.E., Georg, R.B., Halliday, A.N. (2011) Silicon isotopes in Antarctic sponges: an interlaboratory comparison. Antarctic Science 23, 34–42. https://doi.org/10.1017/S0954102010000593

However, there is considerable scatter in the spicule δ30Si-DSi calibration that remains poorly understood. Whilst some studies show good agreement in measurements of spicules taken from individual sponges and good agreement between laboratories (e.g., Hendry et al., 2011), other studies highlight variation within an individual sponge, and between individuals, in both monospecific and more diverse communities (Hendry et al., 2019).
View in article
Repeat measurements of reference standards Diatomite and LMG08 (sponge material) reveal a long term δ30Si reproducibility of 1.24 ± 0.11 ‰ (n = 64) and −3.46 ± 0.12 ‰ (n = 65) respectively (2 s.d.), within published ranges (Reynolds et al., 2007; Hendry et al., 2011).
View in article
Hendry, K.R., Robinson, L.F. (2012) The relationship between silicon isotope fractionation in sponges and silicic acid concentration: Modern and core-top studies of biogenic opal. Geochimica et Cosmochimica Acta 81, 1–12. https://doi.org/10.1016/j.gca.2011.12.010

Several field-based studies have shown that there is a non-linear relationship between the concentration of ambient DSi and both the isotopic composition (denoted by δ30Si) of spicules and apparent isotopic fractionation by sponges during growth that is apparently insensitive to temperature and other seawater properties (Hendry et al., 2010; Wille et al., 2010; Hendry and Robinson, 2012).
View in article
Hendry, K.R., Robinson, L.F., Meredith, M.P., Mulitza, S., Chiessi, C.M., Arz, H. (2012) Abrupt changes in high-latitude nutrient supply to the Atlantic during the last glacial cycle. Geology 40, 123–126. https://doi.org/10.1130/G32779.1

Earlier palaeoceanographic studies simply extracted all spicule material for analysis (e.g., Hendry et al., 2010; Hendry et al., 2012), but more recent studies have taken the conservative approach of picking only one spicule type, usually selecting only the needle-like monaxonic spicules (e.g., Fontorbe et al., 2016; Fontorbe et al., 2017).
View in article
Hendry, K.R., Robinson, L.F., McManus, J.F., Hays, J.D. (2014) Silicon isotopes indicate enhanced carbon export efficiency in the North Atlantic during deglaciation. Nature Communications 5, 3107. https://doi.org/10.1038/ncomms4107

Surface and subsurface silicon cycling processes can be reconstructed using the geochemistry of siliceous microfossils, such as diatoms and radiolarians, extracted from marine sediments (Hendry et al., 2014; Abelmann et al., 2015).
View in article
Hendry, K., Swann, G.E., Leng, M.J., Sloane, H.J., Goodwin, C., Berman, J., Maldonado, M. (2015) Silica stable isotopes and silicification in a carnivorous sponge Asbestopluma sp. Biogeosciences 12, 3489–3498. https://doi.org/10.5194/bg-12-3489-2015

Genetically-controlled biomineralisation pathways appear to be strong drivers of spicule isotopic heterogeneity, with some derived sponge forms, including hypersilicified demosponges, glass sponges, and carnivorous sponges, exhibiting anomalous isotopic fractionation that deviates significantly from other sponges (Hendry et al., 2015; Jochum et al., 2017; Cassarino et al., 2018).
View in article
The samples were analysed within 72 hours of purification for 28Si, 29Si, 30Si, 24Mg, 25Mg, and 26Mg using a Multi-Collector Inductively Coupled Plasma Mass Spectrometer (Thermo Neptune MC-ICP-MS) in medium resolution mode. Sample blank corrections and Mg isotope mass bias corrections were carried out offline, before being normalised to NBS28 (RM8546) (Hendry et al., 2015).
View in article
Hendry, K.R., Cassarino, L., Bates, S.L., Culwick, T., Frost, M., Goodwin, C., Howell, K.L. (2019) Silicon isotopic systematics of deep-sea sponge grounds in the North Atlantic. Quaternary Science Reviews 210, 1–14. https://doi.org/10.1016/j.quascirev.2019.02.017

As such, archives of past deep and bottom water DSi concentrations in addition to surface conditions are essential for understanding past ocean biogeochemistry and climate impacts (Ellwood et al., 2010; Fontorbe et al., 2016; Sutton et al., 2018; Hendry et al., 2019; Dumont et al., 2020).
View in article
At present, there is no evidence for any systematic difference in isotopic fractionation behaviour between the major groups of siliceous sponges, demosponges and hexactinellids, after differences in habitat and depth preference are taken into account (Cassarino et al., 2018; Hendry et al., 2019; Pack et al., 2023).
View in article
However, there is considerable scatter in the spicule δ30Si-DSi calibration that remains poorly understood. Whilst some studies show good agreement in measurements of spicules taken from individual sponges and good agreement between laboratories (e.g., Hendry et al., 2011), other studies highlight variation within an individual sponge, and between individuals, in both monospecific and more diverse communities (Hendry et al., 2019).
View in article
The SB study site is bathed in water with a similar DSi concentration to North Atlantic Geodia sponge aggregations at Orphan Knoll in the Labrador Sea and coastal Greenland (Hendry et al., 2019).
View in article
Our observations show that spicule morphology likely contributes to variation in δ30Si between individuals of similar species, but other factors including localised differences in environmental conditions and growth rate will also play a role (Hendry et al., 2019).
View in article
Instead, these offsets could be due to recycling of silica (i.e. partial dissolution effects), localised differences in environmental conditions, or spicule growth rate (Hendry et al., 2019).
View in article
To test this possibility, we have substituted the range in spicule compositions into Equation 1 (adapted from Hendry et al., 2019):
Eq. 1
where we have assumed the bottom water isotopic composition, δ30Si DSi, to be +1.7 ‰, similar to the deep Central Arctic Basin (Brzezinski et al., 2021).
View in article
Jochum, K., Schuessler, J., Wang, X.H., Stoll, B., Weis, U., Müller, W., Haug, G.H., Andreae, M., Froelich, P. (2017) Whole‐ocean changes in silica and Ge/Si ratios during the Last Deglacial deduced from long‐lived giant glass sponges. Geophysical Research Letters 44, 11,555–11,564. https://doi.org/10.1002/2017GL073897

Genetically-controlled biomineralisation pathways appear to be strong drivers of spicule isotopic heterogeneity, with some derived sponge forms, including hypersilicified demosponges, glass sponges, and carnivorous sponges, exhibiting anomalous isotopic fractionation that deviates significantly from other sponges (Hendry et al., 2015; Jochum et al., 2017; Cassarino et al., 2018).
View in article
Łukowiak, M. (2020) Utilizing sponge spicules in taxonomic, ecological and environmental reconstructions: a review. PeerJ 8, e10601. https://doi.org/10.7717/peerj.10601

In deeper waters benthic siliceous sponges create a skeleton of sponge spicules, which have the potential to act as archives of past bottom water conditions, providing a unique source of the critical information required to reconstruct past changes in DSi supply to the surface ocean from upwelling waters (De La Rocha, 2003; Łukowiak, 2020).
View in article
Łukowiak, M., Van Soest, R., Klautau, M., Pérez, T., Pisera, A., Tabachnick, K. (2022) The terminology of sponge spicules. Journal of Morphology 283, 1517–1545. https://doi.org/10.1002/jmor.21520

The categories represented are: Oxea, Plagiotriaene, Cladotylote, Dichotriaene, Protriaene, Dichodiaene (rare), and Prodiaene (rare) (Łukowiak et al., 2022).
View in article
Meyer, H.K., Davies, A., Roberts, E.M., Xavier, J.R., Ribeiro, P., Glenner, H., Birkely, S.-R., Rapp, H. (2023) Beyond the tip of the seamount: Distinct megabenthic communities found beyond the charismatic summit sponge ground on an arctic seamount (Schulz Bank, Arctic Mid-Ocean Ridge). Deep Sea Research Part I: Oceanographic Research Papers 191, 103920. https://doi.org/10.1016/j.dsr.2022.103920

The summit of the SB is at approximately 560–600 m water depth, with a thick (∼20 cm) sponge spicule mat characterising the upper reaches of the slope and summit (Meyer et al., 2023).
View in article
Pack, A., Bultmann, E.M., Tatzel, M., Reitner, J. (2023) A new method for silicon triple isotope analysis with application to siliceous sponge spicules. Geochemistry, Geophysics, Geosystems 24, e2023GC011243. https://doi.org/10.1029/2023GC011243

At present, there is no evidence for any systematic difference in isotopic fractionation behaviour between the major groups of siliceous sponges, demosponges and hexactinellids, after differences in habitat and depth preference are taken into account (Cassarino et al., 2018; Hendry et al., 2019; Pack et al., 2023).
View in article
Rasmussen, T.L., Thomsen, E., Skirbekk, K., Ślubowska-Woldengen, M., Kristensen, D.K., Koç, N. (2014) Spatial and temporal distribution of Holocene temperature maxima in the northern Nordic seas: interplay of Atlantic-, Arctic-and polar water masses. Quaternary Science Reviews 92, 280–291. https://doi.org/10.1016/j.quascirev.2013.10.034

However, given the assumed age of the core, there is only likely a 6,000 year age difference between the core top and 16 cm depth, and no large scale shifts in Arctic Ocean circulation that would have influenced bottom water conditions and nutrient dynamics are likely over this timescale (Rasmussen et al., 2014).
View in article
Reynolds, B.C., Aggarwal, J., Andre, L., Baxter, D., Beucher, C., Brzezinski, M.A., Engström, E., Georg, R.B., Land, M., Leng, M.J. (2007) An inter-laboratory comparison of Si isotope reference materials. Journal of Analytical Atomic Spectrometry 22, 561–568. https://doi.org/10.1039/B616755A

A three isotope plot shows that all analyses fall on a mass-dependent δ29Si and δ30Si line, with a gradient of 0.510 ± 0.003, within the range of equilibrium or kinetic fractionation (Reynolds et al., 2007).
View in article
Repeat measurements of reference standards Diatomite and LMG08 (sponge material) reveal a long term δ30Si reproducibility of 1.24 ± 0.11 ‰ (n = 64) and −3.46 ± 0.12 ‰ (n = 65) respectively (2 s.d.), within published ranges (Reynolds et al., 2007; Hendry et al., 2011).
View in article
Roberts, E., Mienis, F., Rapp, H.T., Hanz, U., Meyer, H., Davies, A. (2018) Oceanographic setting and short-timescale environmental variability at an Arctic seamount sponge ground. Deep Sea Research Part I: Oceanographic Research Papers 138, 98–113. https://doi.org/10.1016/j.dsr.2018.06.007

Mean nutrient concentrations from between 600 and 800 m on SB are 7.56 mM (2 s.d. 0.63), 13.97 mM (2 s.d. 0.30), and 0.95 mM (2 s.d. 0.01) for DSi, nitrate and phosphate respectively (full details in Roberts et al., 2018).
View in article
A high organic particulate matter supply is maintained by strong diurnal currents, which also act to prevent sedimentation (Roberts et al., 2018).
View in article
Reconstructed [DSi] for Oxea ranged from 12 to 25 mM, with a mean of 16 mM, whereas the range for the other spicules was 7 to 17 mM (mean 11 mM), matching better with modern [DSi] observations (mean value approximately 8 mM; Roberts et al., 2018).
View in article
Savage, P.S., Moynier, F. (2013) Silicon isotopic variation in enstatite meteorites: clues to their origin and Earth-forming material. Earth and Planetary Science Letters 361, 487–496. https://doi.org/10.1016/j.epsl.2012.11.016

All samples and standards were chemically purified using cation exchange resin (Bio-Rad AG50W-X12) following published protocols, which have shown quantitative Si yield and cation retention under neutral or acidic conditions (e.g., Georg et al., 2006; Zambardi and Poitrasson, 2011; Savage and Moynier, 2013).
View in article
Struyf, E., Smis, A., Van Damme, S., Meire, P., Conley, D.J. (2009) The global biogeochemical silicon cycle. Silicon 1, 207–213. https://doi.org/10.1007/s12633-010-9035-x

The global marine silicon cycle plays a critical role in the Earth’s climate system via carbon uptake and sequestration (Struyf et al., 2009; Tréguer et al., 2021).
View in article
Sutton, J.N., André, L., Cardinal, D., Conley, D.J., De Souza, G.F., Dean, J., Dodd, J., Ehlert, C., Ellwood, M.J., Frings, P.J. (2018) A review of the stable isotope bio-geochemistry of the global silicon cycle and its associated trace elements. Frontiers in Earth Science 5, 112. https://doi.org/10.3389/feart.2017.00112

As such, archives of past deep and bottom water DSi concentrations in addition to surface conditions are essential for understanding past ocean biogeochemistry and climate impacts (Ellwood et al., 2010; Fontorbe et al., 2016; Sutton et al., 2018; Hendry et al., 2019; Dumont et al., 2020).
View in article
Tréguer, P.J., Sutton, J.N., Brzezinski, M., Charette, M.A., Devries, T., Dutkiewicz, S., Ehlert, C., Hawkings, J., Leynaert, A., Liu, S.M. (2021) Reviews and syntheses: The biogeochemical cycle of silicon in the modern ocean. Biogeosciences 18, 1269–1289. https://doi.org/10.5194/bg-18-1269-2021

The global marine silicon cycle plays a critical role in the Earth’s climate system via carbon uptake and sequestration (Struyf et al., 2009; Tréguer et al., 2021).
View in article
Trower, E.J., Strauss, J.V., Sperling, E.A., Fischer, W.W. (2021) Isotopic analyses of Ordovician–Silurian siliceous skeletons indicate silica‐depleted Paleozoic oceans. Geobiology 19, 460–472. https://doi.org/10.1111/gbi.12449

Developments in the use of secondary ion mass spectrometer (SIMS) methodologies have also allowed the targeting of individual spicules for isotopic analysis (e.g., Trower et al., 2021).
View in article
Wille, M., Sutton, J., Ellwood, M.J., Sambridge, M., Maher, W., Eggins, S., Kelly, M. (2010) Silicon isotopic fractionation in marine sponges: A new model for understanding silicon isotopic variations in sponges. Earth and Planetary Science Letters 292, 281–289. https://doi.org/10.1016/j.epsl.2010.01.036

Several field-based studies have shown that there is a non-linear relationship between the concentration of ambient DSi and both the isotopic composition (denoted by δ30Si) of spicules and apparent isotopic fractionation by sponges during growth that is apparently insensitive to temperature and other seawater properties (Hendry et al., 2010; Wille et al., 2010; Hendry and Robinson, 2012).
View in article
Zambardi, T., Poitrasson, F. (2011) Precise determination of silicon isotopes in silicate rock reference materials by MC‐ICP‐MS. Geostandards and Geoanalytical Research 35, 89–99. https://doi.org/10.1111/j.1751-908X.2010.00067.x

All samples and standards were chemically purified using cation exchange resin (Bio-Rad AG50W-X12) following published protocols, which have shown quantitative Si yield and cation retention under neutral or acidic conditions (e.g., Georg et al., 2006; Zambardi and Poitrasson, 2011; Savage and Moynier, 2013).
View in article
top
Supplementary Information
The Supplementary Information includes:
- Species of Demosponges and Hexactinellids from the Schulz Bank Sponge Ground
- Supplementary Information References
Download the Supplementary Information (PDF)
Figures
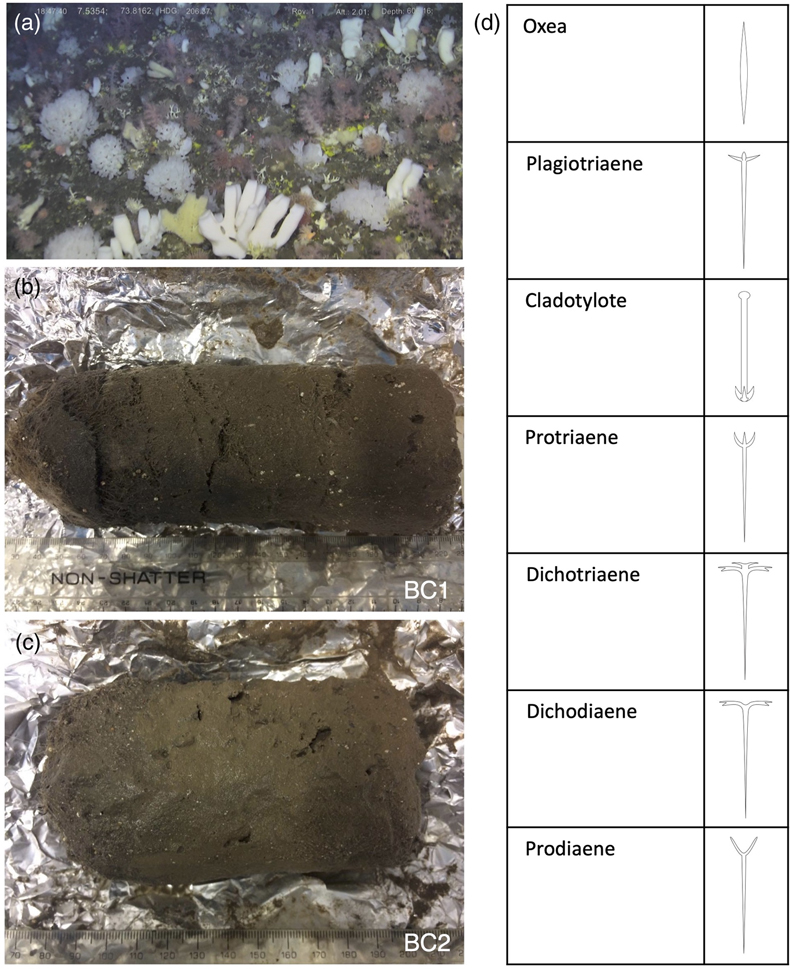
Figure 1 (a) Remotely Operated Vehicle image of the sponge ground of the Schulz Bank summit (image courtesy of the University of Bergen). (b-c) Photographs of the subcores from BC1 and BC2. (d) Spicule morphology definitions used in this study.
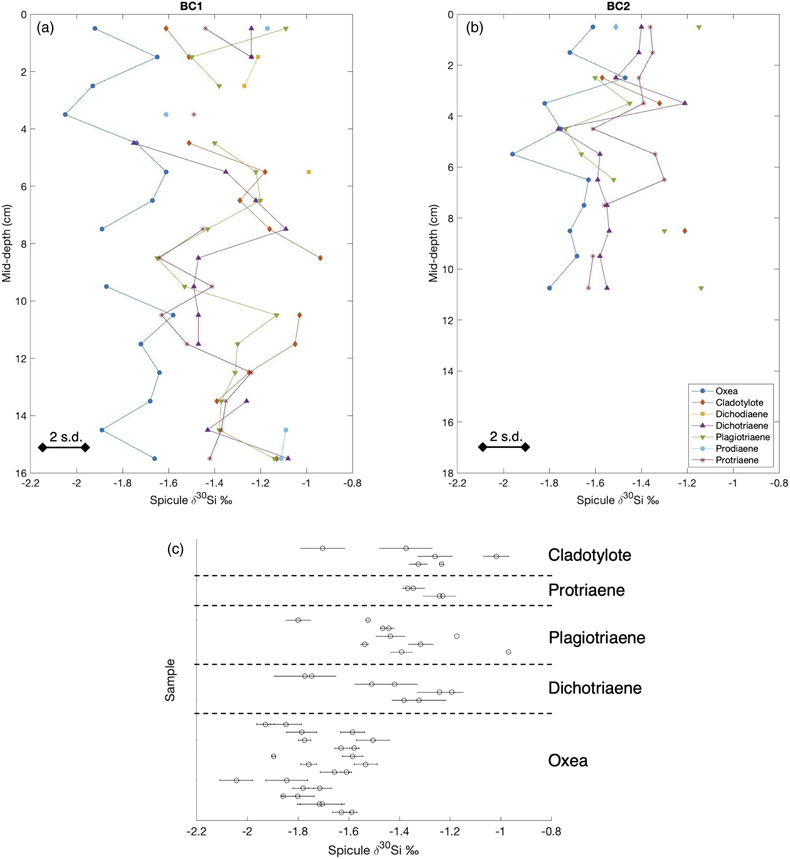
Figure 2 Downcore δ30Si values from the different spicule types extracted from (a) BC1 and (b) BC2. Error bar shows 2 σ based on repeat measurements of reference materials. Each point represents the mean of repeat measurements of a sample aliquot (n = 2 or 3). (c) Assessment of true replicates of separate aliquots from a given horizon. Each symbol represents the mean of repeat measurements (n = 2 or 3, error bar 1 s.d.) of each of multiple aliquots (n = 2) of spicules extracted from the same horizon.
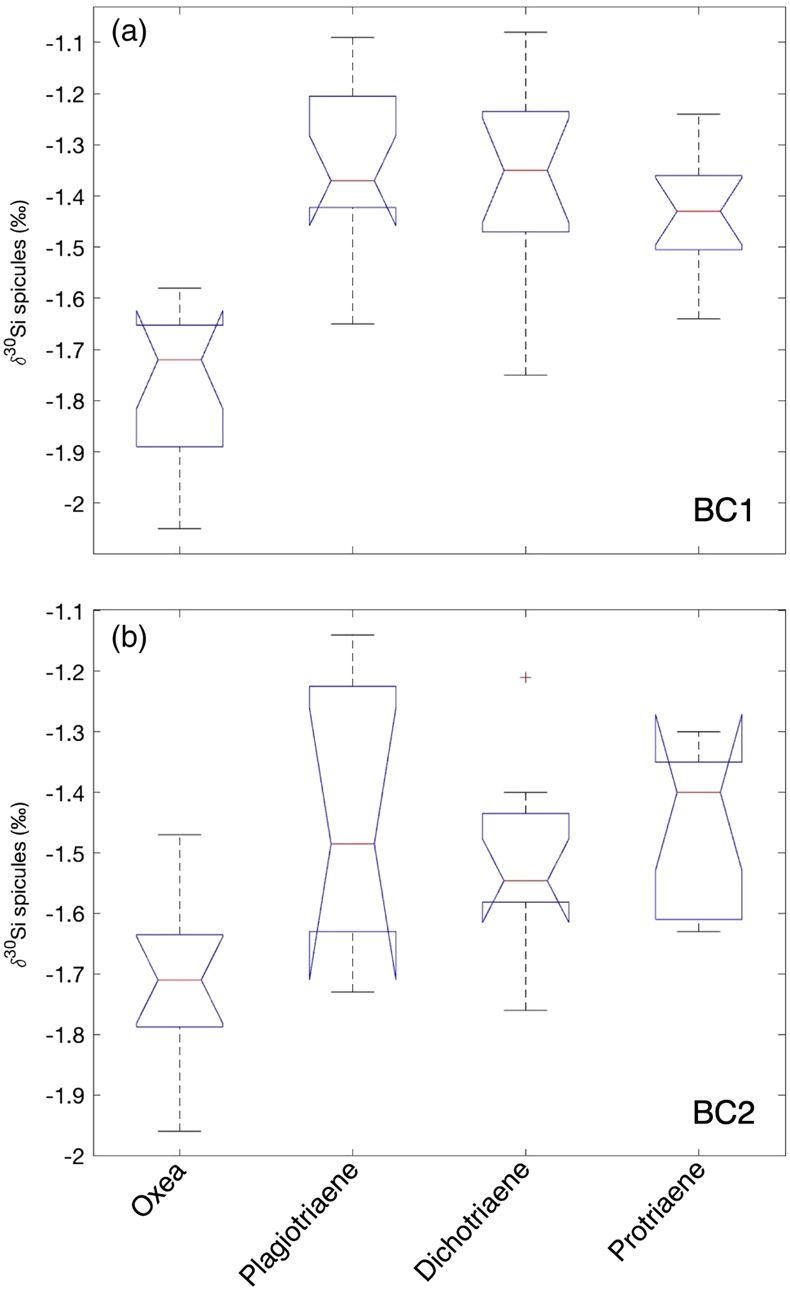
Figure 3 Box and whisker plots summarising downcore δ30Si data from (a) BC1 and (b) BC2 for the main spicule types present in the sediment. The notches represent the median values, the bottom and top edges of the box represent the 25 th and 75 th percentiles, and the whiskers encompass all data points not considered statistical outliers (outliers are shown by a red cross).
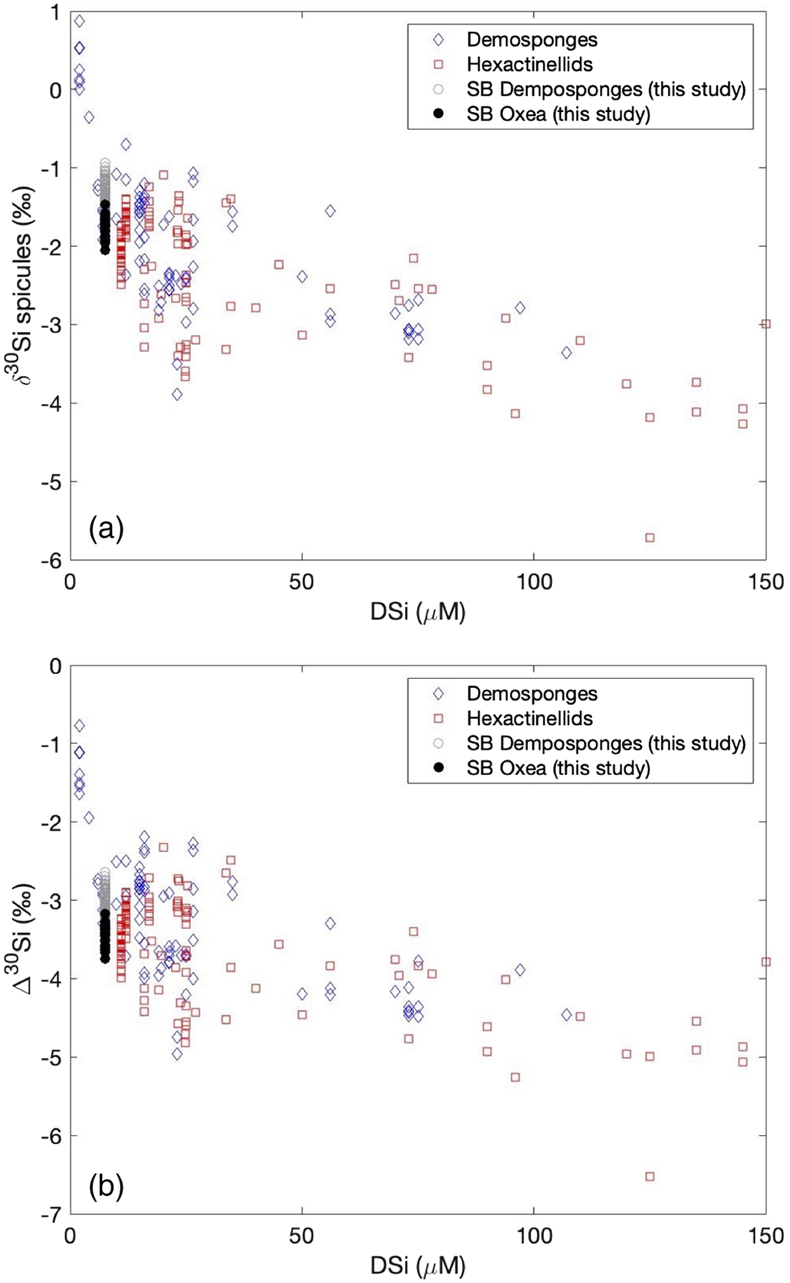
Figure 4 New spicule isotopic data from this study (grey hollow circles are non-Oxea Demosponge spicules; solid circles are Oxea spicules) plotted with existing field calibration of ambient DSi concentrations against (a) sponge spicule δ30Si values and (b) apparent isotopic fractionation (Δ30Si = δ30Sisponge − δ30Sisw), assuming ambient seawater δ30Sisw at the SB of +1.7 ‰ (Brzezinski et al., 2021
Brzezinski, M.A., Closset, I., Jones, J.L., De Souza, G.F., Maden, C. (2021) New constraints on the physical and biological controls on the silicon isotopic composition of the Arctic Ocean. Frontiers in Marine Science 8, 699762. https://doi.org/10.3389/fmars.2021.699762
). Blue symbols = demosponges without Cladorhizidae, red symbols = hexactinellids without hypersilicifiers.