Barium isotope evidence for a magmatic fluid-dominated petrogenesis of LCT-type pegmatites
Affiliations | Corresponding Author | Cite as | Funding information-
Share this article
-
Article views:153Cumulative count of HTML views and PDF downloads.
- Download Citation
-
Rights & Permissions
top
Abstract
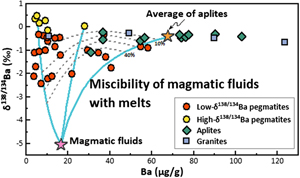
Figures
![]() Figure 1 (a) Location and grades of representative pegmatite, brine, and clay Li deposits worldwide. See data sources in Table S-1. (b) Simplified geological map of the Jiajika pegmatite field (modified after Zhao et al., 2022). |
![]() Figure 2 (a) Schematic cross section of the studied drill hole (modified after Xu et al., 2023). (b) Ba and (c) δ138/134Ba vs. depth. The purple band represents the weighted average δ138/134Ba of the upper continental crust (UCC, 0.00 ± 0.04 ‰; Nan et al., 2018). Error bar is smaller than the symbols. |
![]() Figure 3 δ138/134Ba vs. (a) Ba, (b) Ba/Rb, and (c) Nb/Ta. Arrows in (b) indicate evolution of melts during fractional crystallisation of K-feldspar and mica. (d) Simulation of magmatic fluids mixing with highly differentiated melts and comparison with observations. Numbers on the line of mixing denote the fraction of magmatic fluids. The purple band represents the weighted average δ138/134Ba of the UCC. Error bar is smaller than the symbols. |
![]() Figure 4 Schematic model for the role of magmatic fluids in intra-crustal material transport and petrogenesis and metallogenesis of granitic pegmatites. |
Figure 1 | Figure 2 | Figure 3 | Figure 4 |
top
Introduction
Granitic pegmatites are granitoids with characteristic textures and constitute one of the most important sources of rare metal elements (London, 2018
London, D. (2018) Ore-forming processes within granitic pegmatites. Ore Geology Reviews 101, 349–383. https://doi.org/10.1016/j.oregeorev.2018.04.020
). The Li-Cs-Ta (LCT) pegmatites, as a type of Li deposit with high grade and wide distribution (Fig. 1a), supply approximately half of global Li production and are the main target of future exploration (Benson et al., 2017Benson, T.R., Coble, M.A., Rytuba, J.J., Mahood, G.A. (2017) Lithium enrichment in intracontinental rhyolite magmas leads to Li deposits in caldera basins. Nature Communications 8, 270. https://doi.org/10.1038/s41467-017-00234-y
). Exploring the petrogenesis of LCT-type granitic pegmatites is thus crucial for understanding the enrichment mechanisms of Li and other rare metals.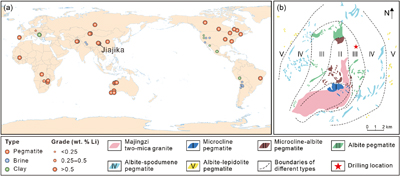
Figure 1 (a) Location and grades of representative pegmatite, brine, and clay Li deposits worldwide. See data sources in Table S-1. (b) Simplified geological map of the Jiajika pegmatite field (modified after Zhao et al., 2022
Zhao, H., Chen, B., Huang, C., Bao, C., Yang, Q., Cao, R. (2022) Geochemical and Sr–Nd–Li isotopic constraints on the genesis of the Jiajika Li-rich pegmatites, eastern Tibetan Plateau: implications for Li mineralization. Contributions to Mineralogy and Petrology 177, 4. https://doi.org/10.1007/s00410-021-01869-3
).Most LCT-type pegmatites are considered to be the product of extreme differentiation of granitic magmas (Thomas and Davidson, 2016
Thomas, R., Davidson, P. (2016) Revisiting complete miscibility between silicate melts and hydrous fluids, and the extreme enrichment of some elements in the supercritical state — Consequences for the formation of pegmatites and ore deposits. Ore Geology Reviews 72, 1088–1101. https://doi.org/10.1016/j.oregeorev.2015.10.004
; London, 2018London, D. (2018) Ore-forming processes within granitic pegmatites. Ore Geology Reviews 101, 349–383. https://doi.org/10.1016/j.oregeorev.2018.04.020
). However, the nature of the pegmatite-forming liquids, especially with regard to the role of H2O and fluxing elements (e.g., B, F, and P), is still highly controversial. The constitutional zone refining model, based mainly on experimental petrology, large scale whole rock analyses, and simulations, suggests that the initial pegmatite-forming liquids are essentially granitic melts inherited from the parental magmas (London, 2018London, D. (2018) Ore-forming processes within granitic pegmatites. Ore Geology Reviews 101, 349–383. https://doi.org/10.1016/j.oregeorev.2018.04.020
). Incompatible components, including H2O, fluxing elements, and rare metals, are continuously enriched in a boundary layer liquid adjacent to the crystallisation front (London, 2014London, D. (2014) A petrologic assessment of internal zonation in granitic pegmatites. Lithos 184–187, 74–104. https://doi.org/10.1016/j.lithos.2013.10.025
). In this scenario, H2O and fluxing elements only play a role in locally lowering viscosity and increasing elemental diffusivity, with aqueous fluid considered unnecessary or even unlikely. In contrast, an alternative model, based mainly on the studies of melt and fluid inclusions, proposes that the formation of LCT-type pegmatites begins with single phase supercritical fluids resulting from miscibility of magmatic fluids and silicate melts (Thomas and Davidson, 2016Thomas, R., Davidson, P. (2016) Revisiting complete miscibility between silicate melts and hydrous fluids, and the extreme enrichment of some elements in the supercritical state — Consequences for the formation of pegmatites and ore deposits. Ore Geology Reviews 72, 1088–1101. https://doi.org/10.1016/j.oregeorev.2015.10.004
). These supercritical fluids can acquire very high initial water and rare metal contents from granitic source magmas, followed by phase separation after emplacement that further enriches Li in the H2O-rich melt/fluid phase (Thomas et al., 2019Thomas, R., Davidson, P., Appel, K. (2019) The enhanced element enrichment in the supercritical states of granite–pegmatite systems. Acta Geochimica 38, 335–349. https://doi.org/10.1007/s11631-019-00319-z
). Numerous attempts have been made to distinguish between the two models (Zhang et al., 2021Zhang, H., Tian, S., Wang, D., Li, X., Liu, T., Zhang, Y., Fu, X., Hao, X., Hou, K., Zhao, Y., Qin, Y. (2021) Lithium isotope behavior during magmatic differentiation and fluid exsolution in the Jiajika granite–pegmatite deposit, Sichuan, China. Ore Geology Reviews 134, 104139. https://doi.org/10.1016/j.oregeorev.2021.104139
; Troch et al., 2022Troch, J., Huber, C., Bachmann, O. (2022) The physical and chemical evolution of magmatic fluids in near-solidus silicic magma reservoirs: Implications for the formation of pegmatites. American Mineralogist 107, 190–205. https://doi.org/10.2138/am-2021-7915
), yet a consensus remains elusive.Barium (Ba) is fluid-mobile and compatible during the crystallisation of granitic magmas. Recent studies highlight the great potential of Ba isotopes in distinguishing between crystal fractionation and magmatic fluid effect. The near eutectic composition of granitic pegmatites implies that K-feldspar controls the Ba budget of the crystalline mineral assemblage, which could result in significantly higher δ138/134Ba (defined as [(138Ba/134Ba)sample /(138Ba/134Ba)NIST3104a − 1] × 1000 (‰)) in the residual melts (Deng et al., 2021
Deng, G., Kang, J., Nan, X., Li, Y., Guo, J., Ding, X., Huang, F. (2021) Barium isotope evidence for crystal-melt separation in granitic magma reservoirs. Geochimica et Cosmochimica Acta 292, 115–129. https://doi.org/10.1016/j.gca.2020.09.027
). Conversely, as magmatic fluids are experimentally demonstrated to preferentially incorporate light Ba isotopes relative to silicate melts (Guo et al., 2020Guo, H., Li, W.-Y., Nan, X., Huang, F. (2020) Experimental evidence for light Ba isotopes favouring aqueous fluids over silicate melts. Geochemical Perspectives Letters 16, 6–11. http://dx.doi.org/10.7185/geochemlet.2036
), their substantial involvement would dramatically reduce δ138/134Ba of the pegmatite systems (Huang et al., 2021Huang, F., Bai, R., Deng, G., Liu, X., Li, X. (2021) Barium isotope evidence for the role of magmatic fluids in the origin of Himalayan leucogranites. Science Bulletin 66, 2329–2336. https://doi.org/10.1016/j.scib.2021.07.020
; G. Deng et al., 2022Deng, G., Jiang, D., Zhang, R., Huang, J., Zhang, X., Huang, F. (2022) Barium isotopes reveal the role of deep magmatic fluids in magmatic-hydrothermal evolution and tin enrichment in granites. Earth and Planetary Science Letters 594, 117724. https://doi.org/10.1016/j.epsl.2022.117724
). Hence, Ba isotopes offer a powerful tool for deciphering the nature of pegmatite-forming liquids.Here, we present high precision Ba isotope data for a suite of borehole samples from the Jiajika granitic pegmatite field (eastern Tibetan Plateau). This pegmatite field is formed within the Jiajika gneiss dome that has a two-mica granite core, mantled by a succession of metasedimentary rocks. More than 500 LCT-type pegmatite veins are spatially zoned around the two-mica granite, in the order from the pluton outward: microcline pegmatite (I), microcline-albite pegmatite (II), albite pegmatite (III), albite-spodumene pegmatite (IV), and albite-lepidolite pegmatite (V) (Fig. 1b). The Li mineralisation is mainly developed within zones IV and V, as well as some veins in zone III, with total estimated Li2O reserves of up to 3.0 Mt, ranking the first in Asia (Huang et al., 2020
Huang, T., Fu, X., Ge, L., Zou, F., Hao, X., Yang, R., Xiao, R., Fan, J. (2020) The genesis of giant lithium pegmatite veins in Jiajika, Sichuan, China: Insights from geophysical, geochemical as well as structural geology approach. Ore Geology Reviews 124, 103557. https://doi.org/10.1016/j.oregeorev.2020.103557
). The studied samples were collected from different depths of a borehole of 3211.21 m, which consists of 35 % metasediments, 14 % granite-aplites, and 51 % pegmatites (Fig. 2a) (Xu et al., 2023Xu, Z., Zheng, B., Zhu, W., Chen, Y., Li, G., Gao, J., Che, X., Zhang, R., Wei, H., Li, W., Wang, G., Wei, G., Yan, H. (2023) Geologic scenario from granitic sheet to Li-rich pegmatite uncovered by Scientific Drilling at the Jiajika lithium deposit in eastern Tibetan Plateau. Ore Geology Reviews 161, 105636. https://doi.org/10.1016/j.oregeorev.2023.105636
). Spodumene-bearing pegmatites are only observed at depths between 0 and 100 m, while deeper pegmatites correspond to surface zones I and II pegmatites without Li mineralisation (Wang et al., 2023Wang, G.-G., Zheng, F.-B., Ni, P., Wu, Y.-W., Qi, W.-X., Li, Z.-A. (2023) Fluid properties and ore-forming process of the giant Jiajika pegmatite Li deposit, western China. Ore Geology Reviews 160, 105613. https://doi.org/10.1016/j.oregeorev.2023.105613
). Detailed geological background and sample description are available in the Supplementary Information. Due to their proximity to the buried main part of the parental granite compared to samples collected from the surface, these borehole samples may provide a strong documentation of the initial compositional characteristics of the pegmatite system. Our results show extremely low δ138/134Ba of pegmatites, supporting a comprehensive influence of magmatic fluids on their compositions, and thus a possible key role in rare metal enrichment.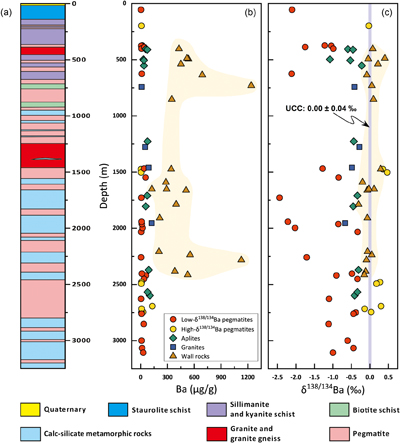
Figure 2 (a) Schematic cross section of the studied drill hole (modified after Xu et al., 2023
Xu, Z., Zheng, B., Zhu, W., Chen, Y., Li, G., Gao, J., Che, X., Zhang, R., Wei, H., Li, W., Wang, G., Wei, G., Yan, H. (2023) Geologic scenario from granitic sheet to Li-rich pegmatite uncovered by Scientific Drilling at the Jiajika lithium deposit in eastern Tibetan Plateau. Ore Geology Reviews 161, 105636. https://doi.org/10.1016/j.oregeorev.2023.105636
). (b) Ba and (c) δ138/134Ba vs. depth. The purple band represents the weighted average δ138/134Ba of the upper continental crust (UCC, 0.00 ± 0.04 ‰; Nan et al., 2018Nan, X.-Y., Yu, H.-M., Rudnick, R.L., Gaschnig, R.M., Xu, J., Li, W.-Y., Zhang, Q., Jin, Z.-D., Li, X.-H., Huang, F. (2018) Barium isotopic composition of the upper continental crust. Geochimica et Cosmochimica Acta 233, 33–49. https://doi.org/10.1016/j.gca.2018.05.004
). Error bar is smaller than the symbols.top
Results
The Ba isotope analytical method is detailed in the Supplementary Information. The long-term external precision for δ138/134Ba is better than 0.05 ‰ (2 s.d.). The δ138/134B of rock standards agree with published values, and the results of replicates are consistent within error (Table S-2), demonstrating the reliability of our data.
There are significant variations in δ138/134Ba of the borehole samples (Fig. 2). Overall, pegmatites have the lowest Ba contents (3.7–130.2 μg/g, mostly <50 μg/g) and the largest range of δ138/134Ba (−2.44 to +0.47 ‰) among the four sample types. Notably, their δ138/134Ba does not show a systematic variation with depth. Granitic aplites and granites have moderate and less variable Ba contents (30.9–102.9 μg/g and 11.0–123.8 μg/g, respectively) and narrower δ138/134Ba ranges (−1.08 to −0.22 ‰ and −0.67 to −0.28 ‰, respectively). The Ba contents of metasedimentary wall rocks (125–1238 μg/g) are significantly higher and more variable than the other samples, but their δ138/134Ba shows a rather restricted range (−0.31 to +0.40 ‰) around a mean of 0.00 ‰, which matches the upper continental crust (UCC, 0.00 ± 0.04 ‰; Nan et al., 2018
Nan, X.-Y., Yu, H.-M., Rudnick, R.L., Gaschnig, R.M., Xu, J., Li, W.-Y., Zhang, Q., Jin, Z.-D., Li, X.-H., Huang, F. (2018) Barium isotopic composition of the upper continental crust. Geochimica et Cosmochimica Acta 233, 33–49. https://doi.org/10.1016/j.gca.2018.05.004
).top
Discussion
Controls on Ba isotope variation. The possible mechanisms driving Ba isotope fractionation in granitoids include chemical alteration, wall rock assimilation, anatexis, and magma differentiation. The first scenario can be excluded as all igneous samples are fresh. Their loss on ignition (LOI) is generally very low (≤1 wt. %, Table S-2), the chemical index of alteration (CIA) mostly falls within the range of fresh granitoids, and neither parameter shows any correlation with δ138/134Ba (Fig. S-1). Secondly, because the wall rocks have a limited δ138/134Ba range that is higher than granites, aplites, and most pegmatites, wall rock assimilation could not produce the extremely low δ138/134Ba of most pegmatites. Although some pegmatite samples have similar δ138/134Ba as the wall rocks, they do not show significantly elevated Ba contents except for sample J084317 (higher than all aplites and granites) (Fig. 3a). Therefore, wall rock assimilation cannot explain Ba isotope fractionation between the Jiajika igneous samples.
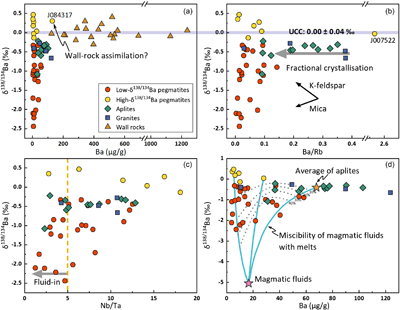
Figure 3 δ138/134Ba vs. (a) Ba, (b) Ba/Rb, and (c) Nb/Ta. Arrows in (b) indicate evolution of melts during fractional crystallisation of K-feldspar and mica. (d) Simulation of magmatic fluids mixing with highly differentiated melts and comparison with observations. Numbers on the line of mixing denote the fraction of magmatic fluids. The purple band represents the weighted average δ138/134Ba of the UCC. Error bar is smaller than the symbols.
Some studies suggest that LCT-type pegmatites may result from low degree muscovite dehydration melting of a metasedimentary source containing Li-rich rocks, rather than as products of extreme magma differentiation (Zhao et al., 2022
Zhao, H., Chen, B., Huang, C., Bao, C., Yang, Q., Cao, R. (2022) Geochemical and Sr–Nd–Li isotopic constraints on the genesis of the Jiajika Li-rich pegmatites, eastern Tibetan Plateau: implications for Li mineralization. Contributions to Mineralogy and Petrology 177, 4. https://doi.org/10.1007/s00410-021-01869-3
). However, our Ba isotope data do not support this model. According to the dehydration melting reaction Muscovite + Plagioclase + Quartz = K-feldspar + Sillimanite + melt, K-feldspar should govern the Ba budget of the restite. Because K-feldspar is enriched in light Ba isotopes relative to melts (Deng et al., 2021Deng, G., Kang, J., Nan, X., Li, Y., Guo, J., Ding, X., Huang, F. (2021) Barium isotope evidence for crystal-melt separation in granitic magma reservoirs. Geochimica et Cosmochimica Acta 292, 115–129. https://doi.org/10.1016/j.gca.2020.09.027
), the resulting melts should acquire higher δ138/134Ba than the source. In this case, the extremely low δ138/134Ba of most pegmatites implies even lower δ138/134Ba for their source rocks, which is not consistent with the observation of (meta-)sedimentary rocks (Nan et al., 2018Nan, X.-Y., Yu, H.-M., Rudnick, R.L., Gaschnig, R.M., Xu, J., Li, W.-Y., Zhang, Q., Jin, Z.-D., Li, X.-H., Huang, F. (2018) Barium isotopic composition of the upper continental crust. Geochimica et Cosmochimica Acta 233, 33–49. https://doi.org/10.1016/j.gca.2018.05.004
). For the pegmatite samples with high δ138/134Ba, their low Rb/Sr does not support muscovite dehydration melting of a metasedimentary source either (Gao et al., 2017Gao, L.-E., Zeng, L., Asimow, P.D. (2017) Contrasting geochemical signatures of fluid-absent versus fluid-fluxed melting of muscovite in metasedimentary sources: The Himalayan leucogranites. Geology 45, 39–42. https://doi.org/10.1130/G38336.1
) (Fig. S-2a).As the vast majority of studies support the Jiajika granite-pegmatite association (Huang et al., 2020
Huang, T., Fu, X., Ge, L., Zou, F., Hao, X., Yang, R., Xiao, R., Fan, J. (2020) The genesis of giant lithium pegmatite veins in Jiajika, Sichuan, China: Insights from geophysical, geochemical as well as structural geology approach. Ore Geology Reviews 124, 103557. https://doi.org/10.1016/j.oregeorev.2020.103557
; Zhang et al., 2021Zhang, H., Tian, S., Wang, D., Li, X., Liu, T., Zhang, Y., Fu, X., Hao, X., Hou, K., Zhao, Y., Qin, Y. (2021) Lithium isotope behavior during magmatic differentiation and fluid exsolution in the Jiajika granite–pegmatite deposit, Sichuan, China. Ore Geology Reviews 134, 104139. https://doi.org/10.1016/j.oregeorev.2021.104139
), the Ba isotope variation among them should be linked to the magma evolution toward pegmatite-forming liquids. Due to the highly evolved nature of the studied granitoids, major element indicators, such as SiO2 and MgO contents, cannot discern the degree of differentiation (Fig. S-2b,c), while trace element ratios, such as Ba/Rb, can help (Zhang et al., 2021Zhang, H., Tian, S., Wang, D., Li, X., Liu, T., Zhang, Y., Fu, X., Hao, X., Hou, K., Zhao, Y., Qin, Y. (2021) Lithium isotope behavior during magmatic differentiation and fluid exsolution in the Jiajika granite–pegmatite deposit, Sichuan, China. Ore Geology Reviews 134, 104139. https://doi.org/10.1016/j.oregeorev.2021.104139
). With the exception of sample J007522, which has abnormally high Ba/Rb likely due to sampling bias, Ba/Rb decreases continuously from granites through aplites to pegmatites (Fig. 3b). This reflects the fractional crystallisation of Ba-rich minerals, which, based on the variation in mineral composition of the borehole samples (Xu et al., 2023Xu, Z., Zheng, B., Zhu, W., Chen, Y., Li, G., Gao, J., Che, X., Zhang, R., Wei, H., Li, W., Wang, G., Wei, G., Yan, H. (2023) Geologic scenario from granitic sheet to Li-rich pegmatite uncovered by Scientific Drilling at the Jiajika lithium deposit in eastern Tibetan Plateau. Ore Geology Reviews 161, 105636. https://doi.org/10.1016/j.oregeorev.2023.105636
), first involves both K-feldspar and mica, and then is dominated by K-feldspar. Because K-feldspar and mica preferentially incorporate light and heavy Ba isotopes, respectively (Deng et al., 2021Deng, G., Kang, J., Nan, X., Li, Y., Guo, J., Ding, X., Huang, F. (2021) Barium isotope evidence for crystal-melt separation in granitic magma reservoirs. Geochimica et Cosmochimica Acta 292, 115–129. https://doi.org/10.1016/j.gca.2020.09.027
), such fractional crystallisation can explain the overall consistent δ138/134Ba of granites and aplites, as well as the elevated δ138/134Ba of some pegmatites (Fig. 3b). However, it cannot account for the significantly lower δ138/134Ba and higher K2O contents of most pegmatites than those of granites and aplites (Fig. S-2d).The low δ138/134Ba and high K2O contents of most pegmatites cannot be explained by crystallisation within pegmatite veins. According to the constitutional zone refining model, light Ba isotopes with higher diffusivity can preferentially feed mineral crystallisation through the boundary layer liquid. Meanwhile, the variation in mineral assemblage of the Jiajika pegmatites indicates that the early crystalline minerals are dominated by Ba- and K-rich microcline that preferentially incorporates light Ba isotopes. Thus, δ138/134Ba of most pegmatites is expected to gradually increase with decreasing Ba and K contents, which is not observed (Figs. 3d, S-2d). Furthermore, although Fe isotope fractionation among these pegmatite samples was suggested to result from fractional crystallisation (Luo et al., 2024
Luo, X.-L., Li, W., Du, D.-H., An, S., Zheng, B., Zhu, W., Xu, Z. (2024) Iron isotope systematics of the Jiajika granite-pegmatite lithium deposit, Sichuan, China. Ore Geology Reviews 165, 105903. https://doi.org/10.1016/j.oregeorev.2024.105903
), no significant correlation between δ138/134Ba and δ56Fe is seen (Fig. S-2e).The most likely process responsible for the low δ138/134Ba of pegmatites is modification by magmatic fluids. According to a previous experimental study by Guo et al. (2020)
Guo, H., Li, W.-Y., Nan, X., Huang, F. (2020) Experimental evidence for light Ba isotopes favouring aqueous fluids over silicate melts. Geochemical Perspectives Letters 16, 6–11. http://dx.doi.org/10.7185/geochemlet.2036
, the apparent Ba isotope fractionation factor between exsolved fluids and residual melts can be up to −4.6 to −5.1 ‰ under the near solidus cold storage conditions (650–600 °C) of the shallow “mushy” magma reservoirs (Rubin et al., 2017Rubin, A.E., Cooper, K.M., Till, C.B., Kent, A.J.R., Costa, F., Bose, M., Gravley, D., Deering, C., Cole, J. (2017) Rapid cooling and cold storage in a silicic magma reservoir recorded in individual crystals. Science 356, 1154–1156. https://doi.org/10.1126/science.aam8720
). Considering the generally low Ba contents of most pegmatites (<50 μg/g with an average of 17.7 μg/g), their extremely low δ138/134Ba could well be the result of the incorporation of magmatic fluids into the pegmatite-forming liquids. This interpretation is in line with their tendency towards low Nb/Ta (<5) and Zr/Hf (<26) (Figs. 3c, S-2f), which are often interpreted as results from magmatic-hydrothermal interaction (Ballouard et al., 2016Ballouard, C., Poujol, M., Boulvais, P., Branquet, Y., Tartese, R., Vigneresse, J.-L. (2016) Nb-Ta fractionation in peraluminous granites: A marker of the magmatic-hydrothermal transition. Geology 44, 231–234. https://doi.org/10.1130/G37475.1
). The slightly higher Nb/Ta and Zr/Hf of some low-δ138/134Ba pegmatites are likely influenced by the crystallisation of accessory minerals, such as columbite-tantalite and zircon, indicating that Ba isotopes might be more sensitive to magmatic fluid activity than these elemental indicators. Consistent with our conclusion, the B and Li isotope compositions (also fluid-mobile elements) of the Jiajika pegmatites have been demonstrated to be significantly modified by magmatic fluids (Zhang et al., 2021Zhang, H., Tian, S., Wang, D., Li, X., Liu, T., Zhang, Y., Fu, X., Hao, X., Hou, K., Zhao, Y., Qin, Y. (2021) Lithium isotope behavior during magmatic differentiation and fluid exsolution in the Jiajika granite–pegmatite deposit, Sichuan, China. Ore Geology Reviews 134, 104139. https://doi.org/10.1016/j.oregeorev.2021.104139
; Huan et al., 2023Huan, C., Wei, H.-Z., Zhu, W.-B., Palmer, M.R., Lin, H.-F., Zheng, B.-H., Cai, Y., Zuo, D.-S., Wang, J.-L., Xu, Z.-Q. (2023) Boron isotopes in tourmaline from drill core of the Jiajika granitic pegmatite type lithium deposit: Insights for granitic magma evolution and lithium enrichment. Ore Geology Reviews 163, 105742. https://doi.org/10.1016/j.oregeorev.2023.105742
). Metamorphic dehydrated fluids from the wall rocks are enriched in heavy Ba isotopes (Wu et al., 2020Wu, F., Turner, S., Schaefer, B.F. (2020) Mélange versus fluid and melt enrichment of subarc mantle: A novel test using barium isotopes in the Tonga-Kermadec arc. Geology 48, 1053–1057. https://doi.org/10.1130/G47549.1
), thus cannot provide the light Ba isotope compositions for most pegmatites.To further decipher the exact mechanism of magmatic fluid participation in pegmatite formation, a model of magmatic fluid mixing with highly differentiated melt is developed in a δ138/134Ba-Ba diagram. Because of continuous decompression and crystallisation, fluid exsolution can occur early in magma evolution and throughout the magma ascent path (Edmonds and Woods, 2018
Edmonds, M., Woods, A.W. (2018) Exsolved volatiles in magma reservoirs. Journal of Volcanology and Geothermal Research 368, 13–30. https://doi.org/10.1016/j.jvolgeores.2018.10.018
). Thus, when calculating the composition of the magmatic fluid using the Rayleigh fractionation equations, the initial melt is represented by the average of all granites and aplites from various depths. The average of aplite samples and two pegmatites with elevated δ138/134Ba and different Ba/Rb ratios covering the main Ba/Rb range of Jiajika pegmatites are selected to represent highly differentiated melts. The calculations and results are detailed in the Supplementary Information.As shown in Figure 3d, the variations in Ba elemental and isotope compositions of most pegmatite samples can be explained by the mixing of 10–40 % magmatic fluids with melts of different degrees of evolution. This range of fluid proportions is much wider than that of typical granitic magmas, but it is consistent with the supercritical fluids model of the pegmatite-forming liquids. The studies of melt and fluid inclusions show that supercritical fluids have initial water contents ranging from 20 to 33.3 % (Thomas and Davidson, 2016
Thomas, R., Davidson, P. (2016) Revisiting complete miscibility between silicate melts and hydrous fluids, and the extreme enrichment of some elements in the supercritical state — Consequences for the formation of pegmatites and ore deposits. Ore Geology Reviews 72, 1088–1101. https://doi.org/10.1016/j.oregeorev.2015.10.004
). After intrusion, they separate into conjugated H2O-poor melts and H2O-rich melts/fluids by melt-melt-fluid immiscibility. The former become the pegmatites with low fluid proportions, while the latter can continue to migrate away from the parental granite due to low viscosities and high mobilities (Troch et al., 2022Troch, J., Huber, C., Bachmann, O. (2022) The physical and chemical evolution of magmatic fluids in near-solidus silicic magma reservoirs: Implications for the formation of pegmatites. American Mineralogist 107, 190–205. https://doi.org/10.2138/am-2021-7915
). Potential supercritical fluid activity in the Jiajika pegmatite field is also supported by previous observations of melt/fluid inclusions. Firstly, representative primary inclusions are commonly enriched in H2O, fluxing components, alkaline elements, CO2, and carbonates, but depleted in alkaline earth elements (Li and Chou, 2017Li, J., Chou, I-M. (2017) Homogenization Experiments of Crystal-Rich Inclusions in Spodumene from Jiajika Lithium Deposit, China, under Elevated External Pressures in a Hydrothermal Diamond-Anvil Cell. Geofluids 2017, 9252913. https://doi.org/10.1155/2017/9252913
; J. Deng et al., 2022Deng, G., Jiang, D., Zhang, R., Huang, J., Zhang, X., Huang, F. (2022) Barium isotopes reveal the role of deep magmatic fluids in magmatic-hydrothermal evolution and tin enrichment in granites. Earth and Planetary Science Letters 594, 117724. https://doi.org/10.1016/j.epsl.2022.117724
). These features are similar to those found in other pegmatites showing supercritical fluid activity (Thomas et al., 2019Thomas, R., Davidson, P., Appel, K. (2019) The enhanced element enrichment in the supercritical states of granite–pegmatite systems. Acta Geochimica 38, 335–349. https://doi.org/10.1007/s11631-019-00319-z
; Wang et al., 2023Wang, G.-G., Zheng, F.-B., Ni, P., Wu, Y.-W., Qi, W.-X., Li, Z.-A. (2023) Fluid properties and ore-forming process of the giant Jiajika pegmatite Li deposit, western China. Ore Geology Reviews 160, 105613. https://doi.org/10.1016/j.oregeorev.2023.105613
), and could explain the higher K2O contents of most pegmatites compared to granites and aplites. Secondly, the trapping conditions of these inclusions (500–720 °C, 3–5 kbar) are consistent with the critical conditions for the complete miscibility of silicate melts with fluids obtained from experiments with addition of B, F, and Na (600 °C, 4 kbar) (Sowerby and Keppler, 2002Sowerby, J.R., Keppler, H. (2002) The effect of fluorine, boron and excess sodium on the critical curve in the albite–H2O system. Contributions to Mineralogy and Petrology 143, 32–37. https://doi.org/10.1007/s00410-001-0334-5
). In this case, the multiple magmatic-hydrothermal events experienced by the Jiajika pegmatites (Xu et al., 2023Xu, Z., Zheng, B., Zhu, W., Chen, Y., Li, G., Gao, J., Che, X., Zhang, R., Wei, H., Li, W., Wang, G., Wei, G., Yan, H. (2023) Geologic scenario from granitic sheet to Li-rich pegmatite uncovered by Scientific Drilling at the Jiajika lithium deposit in eastern Tibetan Plateau. Ore Geology Reviews 161, 105636. https://doi.org/10.1016/j.oregeorev.2023.105636
) could result from multiple injections of supercritical fluids, thereby leading to the lack of systematic variation in their δ138/134Ba with depth. Overall, the Ba isotope signatures of our studied deep pegmatites unequivocally demonstrate that magmatic fluids should overwhelmingly influence the composition and nature of the system from the early stages of pegmatite formation. Barium isotopes can therefore be used in studies of supercritical fluid activity associated with pegmatite systems.Implications for Li enrichment in granitic pegmatites. Despite the importance of granitic pegmatite Li deposits in securing future lithium supply, the mechanism behind Li enrichment, exceeding thousands of times the average continental crust in the formation of such deposits, remains enigmatic. The substantial involvement of magmatic fluids in the petrogenesis of LCT-type pegmatites revealed by our study implies that they should be a key medium in extracting, concentrating, and transporting Li to provide a material source for mineralisation in granitic pegmatites (Fig. 4). The large and super-large pegmatite Li deposits are mainly developed within collisional orogenic belts related to supercontinent assembly (London, 2018
London, D. (2018) Ore-forming processes within granitic pegmatites. Ore Geology Reviews 101, 349–383. https://doi.org/10.1016/j.oregeorev.2018.04.020
), where thickened crust implies more extensive intra-crustal magmatism. Available geophysical studies indicate the presence of vertically extended (up to 15 km) magma reservoirs beneath a number of LCT-type pegmatites like the Jiajika (Huang et al., 2020Huang, T., Fu, X., Ge, L., Zou, F., Hao, X., Yang, R., Xiao, R., Fan, J. (2020) The genesis of giant lithium pegmatite veins in Jiajika, Sichuan, China: Insights from geophysical, geochemical as well as structural geology approach. Ore Geology Reviews 124, 103557. https://doi.org/10.1016/j.oregeorev.2020.103557
). This is consistent with the recent concept of crystal mush-dominated transcrustal magmatic system (Cashman et al., 2017Cashman, K.V., Sparks, R.S.J., Blundy, J.D. (2017) Vertically extensive and unstable magmatic systems: A unified view of igneous processes. Science 355, eaag3055. https://doi.org/10.1126/science.aag3055
), wherein pegmatites are regarded as the last drops of magmatic distillates at the top (Troch et al., 2022Troch, J., Huber, C., Bachmann, O. (2022) The physical and chemical evolution of magmatic fluids in near-solidus silicic magma reservoirs: Implications for the formation of pegmatites. American Mineralogist 107, 190–205. https://doi.org/10.2138/am-2021-7915
). In such a scenario, hydrothermal fluids stored in shallow magma reservoirs represent the time-integrated products of magmatic differentiation and fluid exsolution operating over a significant vertical extent (Blundy et al., 2021Blundy, J., Afanasyev, A., Tattitch, B., Sparks, S., Melnik, O., Utkin, I., Rust, A. (2021) The economic potential of metalliferous sub-volcanic brines. Royal Society Open Science 8, 202192. https://doi.org/10.1098/rsos.202192
). Because ascending magmatic fluids can be decoupled from residual melts, even the fluid-mobile rare metals and fluxing components enriched in the deep crystal mush can be efficiently extracted and transported to aggregate in shallow magma reservoirs through fluid channels among the crystals (Parmigiani et al., 2016Parmigiani, A., Faroughi, S., Huber, C., Bachmann, O., Su, Y. (2016) Bubble accumulation and its role in the evolution of magma reservoirs in the upper crust. Nature 532, 492–495. https://doi.org/10.1038/nature17401
). The elevated contents of fluxing components in shallow magma reservoirs not only effectively reduce the polymerisation of the melts and promote their miscibility with magmatic fluids, but also provide sufficient ligands, thereby enhancing solubility of incompatible rare metals in the resulting supercritical fluids (Thomas and Davidson, 2016Thomas, R., Davidson, P. (2016) Revisiting complete miscibility between silicate melts and hydrous fluids, and the extreme enrichment of some elements in the supercritical state — Consequences for the formation of pegmatites and ore deposits. Ore Geology Reviews 72, 1088–1101. https://doi.org/10.1016/j.oregeorev.2015.10.004
). Theoretically, the supercritical fluids can efficiently extract Li and other rare metal elements with enrichment factors of up to 104 (Thomas et al., 2019Thomas, R., Davidson, P., Appel, K. (2019) The enhanced element enrichment in the supercritical states of granite–pegmatite systems. Acta Geochimica 38, 335–349. https://doi.org/10.1007/s11631-019-00319-z
). Meanwhile, the accumulated magmatic fluids in shallow magma reservoirs are capable of driving overpressure and causing episodic hydraulic fracturing for supercritical fluids intrusion (Holtzman et al., 2012Holtzman, R., Szulczewski, M.L., Juanes, R. (2012) Capillary Fracturing in Granular Media. Physical Review Letters 108, 264504. https://link.aps.org/doi/10.1103/PhysRevLett.108.264504
). Finally, as phase separation occurs to supercritical fluids, Li can be further transported by H2O-rich melt/fluid phase to mineralisation away from the parental granite, as shown by the Jiajika and other LCT-type granitic pegmatite fields worldwide (London, 2018London, D. (2018) Ore-forming processes within granitic pegmatites. Ore Geology Reviews 101, 349–383. https://doi.org/10.1016/j.oregeorev.2018.04.020
; Troch et al., 2022Troch, J., Huber, C., Bachmann, O. (2022) The physical and chemical evolution of magmatic fluids in near-solidus silicic magma reservoirs: Implications for the formation of pegmatites. American Mineralogist 107, 190–205. https://doi.org/10.2138/am-2021-7915
). In short, fluid exsolution within the parental magmatic system provides an additional material source for pegmatite petrogenesis and mineralisation. Liquid immiscibility within pegmatite veins determines the spatial distribution of Li mineralised pegmatites. Therefore, after observing highly differentiated granites with high Li content in the collisional orogenic belts, potential Li-rich pegmatites in wall rocks could occur vertically higher and/or horizontally distant (up to several kilometres), which can be an effective exploration strategy for pegmatite Li deposits.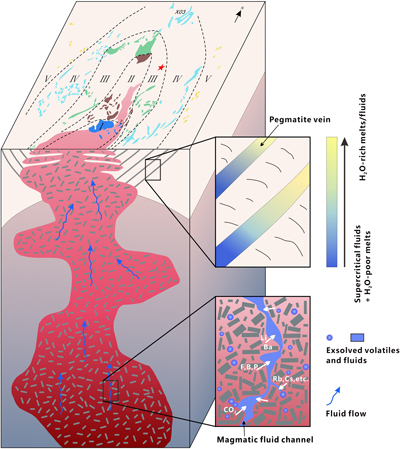
Figure 4 Schematic model for the role of magmatic fluids in intra-crustal material transport and petrogenesis and metallogenesis of granitic pegmatites.
top
Acknowledgements
This work is supported by grants from the Postdoctoral Foundation of China (BH2080000157) and the National Natural Science Foundation of China (GG2080000748).
Editor: Raul O.C. Fonseca
top
References
Ballouard, C., Poujol, M., Boulvais, P., Branquet, Y., Tartese, R., Vigneresse, J.-L. (2016) Nb-Ta fractionation in peraluminous granites: A marker of the magmatic-hydrothermal transition. Geology 44, 231–234. https://doi.org/10.1130/G37475.1

This interpretation is in line with their tendency towards low Nb/Ta (<5) and Zr/Hf (<26) (Figs. 3c, S-2f), which are often interpreted as results from magmatic-hydrothermal interaction (Ballouard et al., 2016).
View in article
Benson, T.R., Coble, M.A., Rytuba, J.J., Mahood, G.A. (2017) Lithium enrichment in intracontinental rhyolite magmas leads to Li deposits in caldera basins. Nature Communications 8, 270. https://doi.org/10.1038/s41467-017-00234-y

The Li-Cs-Ta (LCT) pegmatites, as a type of Li deposit with high grade and wide distribution (Fig. 1a), supply approximately half of global Li production and are the main target of future exploration (Benson et al., 2017).
View in article
Blundy, J., Afanasyev, A., Tattitch, B., Sparks, S., Melnik, O., Utkin, I., Rust, A. (2021) The economic potential of metalliferous sub-volcanic brines. Royal Society Open Science 8, 202192. https://doi.org/10.1098/rsos.202192

In such a scenario, hydrothermal fluids stored in shallow magma reservoirs represent the time-integrated products of magmatic differentiation and fluid exsolution operating over a significant vertical extent (Blundy et al., 2021).
View in article
Cashman, K.V., Sparks, R.S.J., Blundy, J.D. (2017) Vertically extensive and unstable magmatic systems: A unified view of igneous processes. Science 355, eaag3055. https://doi.org/10.1126/science.aag3055

This is consistent with the recent concept of crystal mush-dominated transcrustal magmatic system (Cashman et al., 2017), wherein pegmatites are regarded as the last drops of magmatic distillates at the top (Troch et al., 2022).
View in article
Deng, G., Kang, J., Nan, X., Li, Y., Guo, J., Ding, X., Huang, F. (2021) Barium isotope evidence for crystal-melt separation in granitic magma reservoirs. Geochimica et Cosmochimica Acta 292, 115–129. https://doi.org/10.1016/j.gca.2020.09.027

The near eutectic composition of granitic pegmatites implies that K-feldspar controls the Ba budget of the crystalline mineral assemblage, which could result in significantly higher δ138/134Ba (defined as [(138Ba/134Ba)sample /(138Ba/134Ba)NIST3104a − 1] × 1000 (‰)) in the residual melts (Deng et al., 2021).
View in article
Because K-feldspar is enriched in light Ba isotopes relative to melts (Deng et al., 2021), the resulting melts should acquire higher δ138/134Ba than the source.
View in article
Because K-feldspar and mica preferentially incorporate light and heavy Ba isotopes, respectively (Deng et al., 2021), such fractional crystallisation can explain the overall consistent δ138/134Ba of granites and aplites, as well as the elevated δ138/134Ba of some pegmatites (Fig. 3b).
View in article
Deng, G., Jiang, D., Zhang, R., Huang, J., Zhang, X., Huang, F. (2022) Barium isotopes reveal the role of deep magmatic fluids in magmatic-hydrothermal evolution and tin enrichment in granites. Earth and Planetary Science Letters 594, 117724. https://doi.org/10.1016/j.epsl.2022.117724

Conversely, as magmatic fluids are experimentally demonstrated to preferentially incorporate light Ba isotopes relative to silicate melts (Guo et al., 2020), their substantial involvement would dramatically reduce δ138/134Ba of the pegmatite systems (Huang et al., 2021; G. Deng et al., 2022).
View in article
Firstly, representative primary inclusions are commonly enriched in H2O, fluxing components, alkaline elements, CO2, and carbonates, but depleted in alkaline earth elements (Li and Chou, 2017; J. Deng et al., 2022).
View in article
Deng, J., Li, J., Zhang, D., Chou, I-M., Yan, Q., Xiong, X. (2022) Origin of pegmatitic melts from granitic magmas in the formation of the Jiajika lithium deposit in the eastern Tibetan Plateau. Journal of Asian Earth Sciences 229, 105147. https://doi.org/10.1016/j.jseaes.2022.105147

Conversely, as magmatic fluids are experimentally demonstrated to preferentially incorporate light Ba isotopes relative to silicate melts (Guo et al., 2020), their substantial involvement would dramatically reduce δ138/134Ba of the pegmatite systems (Huang et al., 2021; G. Deng et al., 2022).
View in article
Edmonds, M., Woods, A.W. (2018) Exsolved volatiles in magma reservoirs. Journal of Volcanology and Geothermal Research 368, 13–30. https://doi.org/10.1016/j.jvolgeores.2018.10.018

Because of continuous decompression and crystallisation, fluid exsolution can occur early in magma evolution and throughout the magma ascent path (Edmonds and Woods, 2018).
View in article
Gao, L.-E., Zeng, L., Asimow, P.D. (2017) Contrasting geochemical signatures of fluid-absent versus fluid-fluxed melting of muscovite in metasedimentary sources: The Himalayan leucogranites. Geology 45, 39–42. https://doi.org/10.1130/G38336.1

For the pegmatite samples with high δ138/134Ba, their low Rb/Sr does not support muscovite dehydration melting of a metasedimentary source either (Gao et al., 2017) (Fig. S-2a).
View in article
Guo, H., Li, W.-Y., Nan, X., Huang, F. (2020) Experimental evidence for light Ba isotopes favouring aqueous fluids over silicate melts. Geochemical Perspectives Letters 16, 6–11. https://dx.doi.org/10.7185/geochemlet.2036

Conversely, as magmatic fluids are experimentally demonstrated to preferentially incorporate light Ba isotopes relative to silicate melts (Guo et al., 2020), their substantial involvement would dramatically reduce δ138/134Ba of the pegmatite systems (Huang et al., 2021; G. Deng et al., 2022).
View in article
According to a previous experimental study by Guo et al. (2020), the apparent Ba isotope fractionation factor between exsolved fluids and residual melts can be up to −4.6 to −5.1 ‰ under the near solidus cold storage conditions (650–600 °C) of the shallow “mushy” magma reservoirs (Rubin et al., 2017).
View in article
Holtzman, R., Szulczewski, M.L., Juanes, R. (2012) Capillary Fracturing in Granular Media. Physical Review Letters 108, 264504. https://link.aps.org/doi/10.1103/PhysRevLett.108.264504

Meanwhile, the accumulated magmatic fluids in shallow magma reservoirs are capable of driving overpressure and causing episodic hydraulic fracturing for supercritical fluids intrusion (Holtzman et al., 2012).
View in article
Huan, C., Wei, H.-Z., Zhu, W.-B., Palmer, M.R., Lin, H.-F., Zheng, B.-H., Cai, Y., Zuo, D.-S., Wang, J.-L., Xu, Z.-Q. (2023) Boron isotopes in tourmaline from drill core of the Jiajika granitic pegmatite type lithium deposit: Insights for granitic magma evolution and lithium enrichment. Ore Geology Reviews 163, 105742. https://doi.org/10.1016/j.oregeorev.2023.105742

Consistent with our conclusion, the B and Li isotope compositions (also fluid-mobile elements) of the Jiajika pegmatites have been demonstrated to be significantly modified by magmatic fluids (Zhang et al., 2021; Huan et al., 2023).
View in article
Huang, F., Bai, R., Deng, G., Liu, X., Li, X. (2021) Barium isotope evidence for the role of magmatic fluids in the origin of Himalayan leucogranites. Science Bulletin 66, 2329–2336. https://doi.org/10.1016/j.scib.2021.07.020

Conversely, as magmatic fluids are experimentally demonstrated to preferentially incorporate light Ba isotopes relative to silicate melts (Guo et al., 2020), their substantial involvement would dramatically reduce δ138/134Ba of the pegmatite systems (Huang et al., 2021; G. Deng et al., 2022).
View in article
Huang, T., Fu, X., Ge, L., Zou, F., Hao, X., Yang, R., Xiao, R., Fan, J. (2020) The genesis of giant lithium pegmatite veins in Jiajika, Sichuan, China: Insights from geophysical, geochemical as well as structural geology approach. Ore Geology Reviews 124, 103557. https://doi.org/10.1016/j.oregeorev.2020.103557

The Li mineralisation is mainly developed within zones IV and V, as well as some veins in zone III, with total estimated Li2O reserves of up to 3.0 Mt, ranking the first in Asia (Huang et al., 2020).
View in article
As the vast majority of studies support the Jiajika granite-pegmatite association (Huang et al., 2020; Zhang et al., 2021), the Ba isotope variation among them should be linked to the magma evolution toward pegmatite-forming liquids.
View in article
Available geophysical studies indicate the presence of vertically extended (up to 15 km) magma reservoirs beneath a number of LCT-type pegmatites like the Jiajika (Huang et al., 2020).
View in article
Li, J., Chou, I-M. (2017) Homogenization Experiments of Crystal-Rich Inclusions in Spodumene from Jiajika Lithium Deposit, China, under Elevated External Pressures in a Hydrothermal Diamond-Anvil Cell. Geofluids 2017, 9252913. https://doi.org/10.1155/2017/9252913

Firstly, representative primary inclusions are commonly enriched in H2O, fluxing components, alkaline elements, CO2, and carbonates, but depleted in alkaline earth elements (Li and Chou, 2017; J. Deng et al., 2022).
View in article
London, D. (2014) A petrologic assessment of internal zonation in granitic pegmatites. Lithos 184–187, 74–104. https://doi.org/10.1016/j.lithos.2013.10.025

Incompatible components, including H2O, fluxing elements, and rare metals, are continuously enriched in a boundary layer liquid adjacent to the crystallisation front (London, 2014).
View in article
London, D. (2018) Ore-forming processes within granitic pegmatites. Ore Geology Reviews 101, 349–383. https://doi.org/10.1016/j.oregeorev.2018.04.020

Granitic pegmatites are granitoids with characteristic textures and constitute one of the most important sources of rare metal elements (London, 2018).
View in article
Most LCT-type pegmatites are considered to be the product of extreme differentiation of granitic magmas (Thomas and Davidson, 2016; London, 2018).
View in article
The constitutional zone refining model, based mainly on experimental petrology, large scale whole rock analyses, and simulations, suggests that the initial pegmatite-forming liquids are essentially granitic melts inherited from the parental magmas (London, 2018).
View in article
The large and super-large pegmatite Li deposits are mainly developed within collisional orogenic belts related to supercontinent assembly (London, 2018), where thickened crust implies more extensive intra-crustal magmatism.
View in article
Finally, as phase separation occurs to supercritical fluids, Li can be further transported by H2O-rich melt/fluid phase to mineralisation away from the parental granite, as shown by the Jiajika and other LCT-type granitic pegmatite fields worldwide (London, 2018; Troch et al., 2022).
View in article
Luo, X.-L., Li, W., Du, D.-H., An, S., Zheng, B., Zhu, W., Xu, Z. (2024) Iron isotope systematics of the Jiajika granite-pegmatite lithium deposit, Sichuan, China. Ore Geology Reviews 165, 105903. https://doi.org/10.1016/j.oregeorev.2024.105903

Furthermore, although Fe isotope fractionation among these pegmatite samples was suggested to result from fractional crystallisation (Luo et al., 2024), no significant correlation between δ138/134Ba and δ56Fe is seen (Fig. S-2e).
View in article
Nan, X.-Y., Yu, H.-M., Rudnick, R.L., Gaschnig, R.M., Xu, J., Li, W.-Y., Zhang, Q., Jin, Z.-D., Li, X.-H., Huang, F. (2018) Barium isotopic composition of the upper continental crust. Geochimica et Cosmochimica Acta 233, 33–49. https://doi.org/10.1016/j.gca.2018.05.004

The purple band represents the weighted average δ138/134Ba of the upper continental crust (UCC, 0.00 ± 0.04 ‰; Nan et al., 2018).
View in article
The Ba contents of metasedimentary wall rocks (125–1238 μg/g) are significantly higher and more variable than the other samples, but their δ138/134Ba shows a rather restricted range (−0.31 to +0.40 ‰) around a mean of 0.00 ‰, which matches the upper continental crust (UCC, 0.00 ± 0.04 ‰; Nan et al., 2018).
View in article
In this case, the extremely low δ138/134Ba of most pegmatites implies even lower δ138/134Ba for their source rocks, which is not consistent with the observation of (meta-)sedimentary rocks (Nan et al., 2018).
View in article
Parmigiani, A., Faroughi, S., Huber, C., Bachmann, O., Su, Y. (2016) Bubble accumulation and its role in the evolution of magma reservoirs in the upper crust. Nature 532, 492–495. https://doi.org/10.1038/nature17401

Because ascending magmatic fluids can be decoupled from residual melts, even the fluid-mobile rare metals and fluxing components enriched in the deep crystal mush can be efficiently extracted and transported to aggregate in shallow magma reservoirs through fluid channels among the crystals (Parmigiani et al., 2016).
View in article
Rubin, A.E., Cooper, K.M., Till, C.B., Kent, A.J.R., Costa, F., Bose, M., Gravley, D., Deering, C., Cole, J. (2017) Rapid cooling and cold storage in a silicic magma reservoir recorded in individual crystals. Science 356, 1154–1156. https://doi.org/10.1126/science.aam8720

According to a previous experimental study by Guo et al. (2020), the apparent Ba isotope fractionation factor between exsolved fluids and residual melts can be up to −4.6 to −5.1 ‰ under the near solidus cold storage conditions (650–600 °C) of the shallow “mushy” magma reservoirs (Rubin et al., 2017).
View in article
Sowerby, J.R., Keppler, H. (2002) The effect of fluorine, boron and excess sodium on the critical curve in the albite–H2O system. Contributions to Mineralogy and Petrology 143, 32–37. https://doi.org/10.1007/s00410-001-0334-5

Secondly, the trapping conditions of these inclusions (500–720 °C, 3–5 kbar) are consistent with the critical conditions for the complete miscibility of silicate melts with fluids obtained from experiments with addition of B, F, and Na (600 °C, 4 kbar) (Sowerby and Keppler, 2002).
View in article
Thomas, R., Davidson, P. (2016) Revisiting complete miscibility between silicate melts and hydrous fluids, and the extreme enrichment of some elements in the supercritical state — Consequences for the formation of pegmatites and ore deposits. Ore Geology Reviews 72, 1088–1101. https://doi.org/10.1016/j.oregeorev.2015.10.004

Most LCT-type pegmatites are considered to be the product of extreme differentiation of granitic magmas (Thomas and Davidson, 2016; London, 2018).
View in article
In contrast, an alternative model, based mainly on the studies of melt and fluid inclusions, proposes that the formation of LCT-type pegmatites begins with single phase supercritical fluids resulting from miscibility of magmatic fluids and silicate melts (Thomas and Davidson, 2016).
View in article
The studies of melt and fluid inclusions show that supercritical fluids have initial water contents ranging from 20 to 33.3 % (Thomas and Davidson, 2016).
View in article
The elevated contents of fluxing components in shallow magma reservoirs not only effectively reduce the polymerisation of the melts and promote their miscibility with magmatic fluids, but also provide sufficient ligands, thereby enhancing solubility of incompatible rare metals in the resulting supercritical fluids (Thomas and Davidson, 2016).
View in article
Thomas, R., Davidson, P., Appel, K. (2019) The enhanced element enrichment in the supercritical states of granite–pegmatite systems. Acta Geochimica 38, 335–349. https://doi.org/10.1007/s11631-019-00319-z

These supercritical fluids can acquire very high initial water and rare metal contents from granitic source magmas, followed by phase separation after emplacement that further enriches Li in the H2O-rich melt/fluid phase (Thomas et al., 2019).
View in article
These features are similar to those found in other pegmatites showing supercritical fluid activity (Thomas et al., 2019; Wang et al., 2023), and could explain the higher K2O contents of most pegmatites compared to granites and aplites.
View in article
Theoretically, the supercritical fluids can efficiently extract Li and other rare metal elements with enrichment factors of up to 104 (Thomas et al., 2019).
View in article
Troch, J., Huber, C., Bachmann, O. (2022) The physical and chemical evolution of magmatic fluids in near-solidus silicic magma reservoirs: Implications for the formation of pegmatites. American Mineralogist 107, 190–205. https://doi.org/10.2138/am-2021-7915

Numerous attempts have been made to distinguish between the two models (Zhang et al., 2021; Troch et al., 2022), yet a consensus remains elusive.
View in article
The former become the pegmatites with low fluid proportions, while the latter can continue to migrate away from the parental granite due to low viscosities and high mobilities (Troch et al., 2022).
View in article
This is consistent with the recent concept of crystal mush-dominated transcrustal magmatic system (Cashman et al., 2017), wherein pegmatites are regarded as the last drops of magmatic distillates at the top (Troch et al., 2022).
View in article
Finally, as phase separation occurs to supercritical fluids, Li can be further transported by H2O-rich melt/fluid phase to mineralisation away from the parental granite, as shown by the Jiajika and other LCT-type granitic pegmatite fields worldwide (London, 2018; Troch et al., 2022).
View in article
Wang, G.-G., Zheng, F.-B., Ni, P., Wu, Y.-W., Qi, W.-X., Li, Z.-A. (2023) Fluid properties and ore-forming process of the giant Jiajika pegmatite Li deposit, western China. Ore Geology Reviews 160, 105613. https://doi.org/10.1016/j.oregeorev.2023.105613

Spodumene-bearing pegmatites are only observed at depths between 0 and 100 m, while deeper pegmatites correspond to surface zones I and II pegmatites without Li mineralisation (Wang et al., 2023).
View in article
These features are similar to those found in other pegmatites showing supercritical fluid activity (Thomas et al., 2019; Wang et al., 2023), and could explain the higher K2O contents of most pegmatites compared to granites and aplites.
View in article
Wu, F., Turner, S., Schaefer, B.F. (2020) Mélange versus fluid and melt enrichment of subarc mantle: A novel test using barium isotopes in the Tonga-Kermadec arc. Geology 48, 1053–1057. https://doi.org/10.1130/G47549.1

Metamorphic dehydrated fluids from the wall rocks are enriched in heavy Ba isotopes (Wu et al., 2020), thus cannot provide the light Ba isotope compositions for most pegmatites.
View in article
Xu, Z., Zheng, B., Zhu, W., Chen, Y., Li, G., Gao, J., Che, X., Zhang, R., Wei, H., Li, W., Wang, G., Wei, G., Yan, H. (2023) Geologic scenario from granitic sheet to Li-rich pegmatite uncovered by Scientific Drilling at the Jiajika lithium deposit in eastern Tibetan Plateau. Ore Geology Reviews 161, 105636. https://doi.org/10.1016/j.oregeorev.2023.105636

The studied samples were collected from different depths of a borehole of 3211.21 m, which consists of 35 % metasediments, 14 % granite-aplites, and 51 % pegmatites (Fig. 2a) (Xu et al., 2023).
View in article
(a) Schematic cross section of the studied drill hole (modified after Xu et al., 2023).
View in article
This reflects the fractional crystallisation of Ba-rich minerals, which, based on the variation in mineral composition of the borehole samples (Xu et al., 2023), first involves both K-feldspar and mica, and then is dominated by K-feldspar.
View in article
In this case, the multiple magmatic-hydrothermal events experienced by the Jiajika pegmatites (Xu et al., 2023) could result from multiple injections of supercritical fluids, thereby leading to the lack of systematic variation in their δ138/134Ba with depth.
View in article
Zhang, H., Tian, S., Wang, D., Li, X., Liu, T., Zhang, Y., Fu, X., Hao, X., Hou, K., Zhao, Y., Qin, Y. (2021) Lithium isotope behavior during magmatic differentiation and fluid exsolution in the Jiajika granite–pegmatite deposit, Sichuan, China. Ore Geology Reviews 134, 104139. https://doi.org/10.1016/j.oregeorev.2021.104139

Numerous attempts have been made to distinguish between the two models (Zhang et al., 2021; Troch et al., 2022), yet a consensus remains elusive.
View in article
As the vast majority of studies support the Jiajika granite-pegmatite association (Huang et al., 2020; Zhang et al., 2021), the Ba isotope variation among them should be linked to the magma evolution toward pegmatite-forming liquids.
View in article
Due to the highly evolved nature of the studied granitoids, major element indicators, such as SiO2 and MgO contents, cannot discern the degree of differentiation (Fig. S-2b,c), while trace element ratios, such as Ba/Rb, can help (Zhang et al., 2021).
View in article
Consistent with our conclusion, the B and Li isotope compositions (also fluid-mobile elements) of the Jiajika pegmatites have been demonstrated to be significantly modified by magmatic fluids (Zhang et al., 2021; Huan et al., 2023).
View in article
Zhao, H., Chen, B., Huang, C., Bao, C., Yang, Q., Cao, R. (2022) Geochemical and Sr–Nd–Li isotopic constraints on the genesis of the Jiajika Li-rich pegmatites, eastern Tibetan Plateau: implications for Li mineralization. Contributions to Mineralogy and Petrology 177, 4. https://doi.org/10.1007/s00410-021-01869-3

(b) Simplified geological map of the Jiajika pegmatite field (modified after Zhao et al., 2022).
View in article
Some studies suggest that LCT-type pegmatites may result from low degree muscovite dehydration melting of a metasedimentary source containing Li-rich rocks, rather than as products of extreme magma differentiation (Zhao et al., 2022).
View in article
top
Supplementary Information
The Supplementary Information includes:
- Geological Background and Samples
- Analytical Method
- Simulation of Magmatic Fluid Mixing with Highly Differentiated Melts
- Figures S-1 and S-2
- Tables S-1 to S-3
- Supplementary Information References
Download the Supplementary Information (PDF)
Download Tables S-1 to S-3 (.xlsx)
Figures
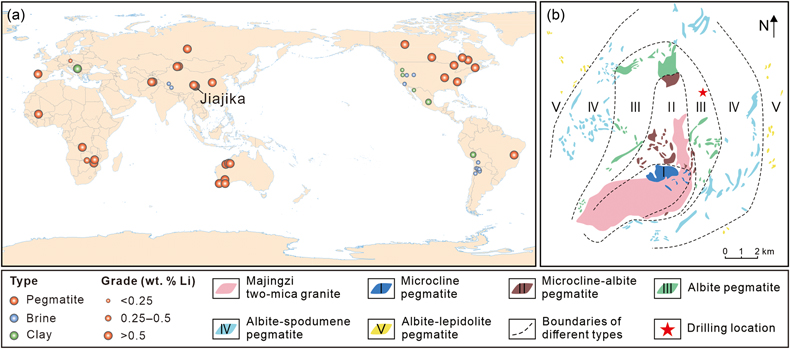
Figure 1 (a) Location and grades of representative pegmatite, brine, and clay Li deposits worldwide. See data sources in Table S-1. (b) Simplified geological map of the Jiajika pegmatite field (modified after Zhao et al., 2022
Zhao, H., Chen, B., Huang, C., Bao, C., Yang, Q., Cao, R. (2022) Geochemical and Sr–Nd–Li isotopic constraints on the genesis of the Jiajika Li-rich pegmatites, eastern Tibetan Plateau: implications for Li mineralization. Contributions to Mineralogy and Petrology 177, 4. https://doi.org/10.1007/s00410-021-01869-3
).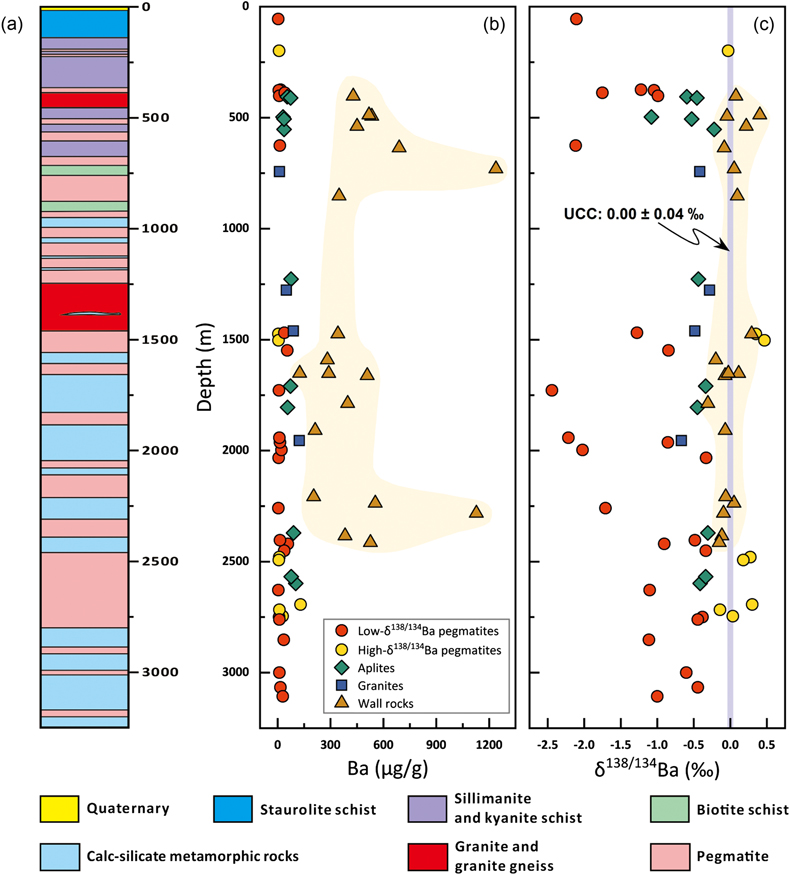
Figure 2 (a) Schematic cross section of the studied drill hole (modified after Xu et al., 2023
Xu, Z., Zheng, B., Zhu, W., Chen, Y., Li, G., Gao, J., Che, X., Zhang, R., Wei, H., Li, W., Wang, G., Wei, G., Yan, H. (2023) Geologic scenario from granitic sheet to Li-rich pegmatite uncovered by Scientific Drilling at the Jiajika lithium deposit in eastern Tibetan Plateau. Ore Geology Reviews 161, 105636. https://doi.org/10.1016/j.oregeorev.2023.105636
). (b) Ba and (c) δ138/134Ba vs. depth. The purple band represents the weighted average δ138/134Ba of the upper continental crust (UCC, 0.00 ± 0.04 ‰; Nan et al., 2018Nan, X.-Y., Yu, H.-M., Rudnick, R.L., Gaschnig, R.M., Xu, J., Li, W.-Y., Zhang, Q., Jin, Z.-D., Li, X.-H., Huang, F. (2018) Barium isotopic composition of the upper continental crust. Geochimica et Cosmochimica Acta 233, 33–49. https://doi.org/10.1016/j.gca.2018.05.004
). Error bar is smaller than the symbols.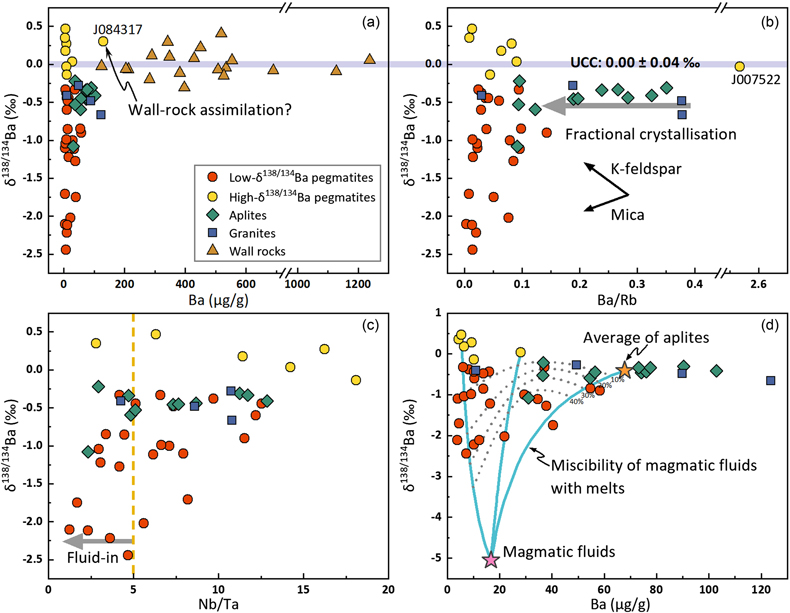
Figure 3 δ138/134Ba vs. (a) Ba, (b) Ba/Rb, and (c) Nb/Ta. Arrows in (b) indicate evolution of melts during fractional crystallisation of K-feldspar and mica. (d) Simulation of magmatic fluids mixing with highly differentiated melts and comparison with observations. Numbers on the line of mixing denote the fraction of magmatic fluids. The purple band represents the weighted average δ138/134Ba of the UCC. Error bar is smaller than the symbols.
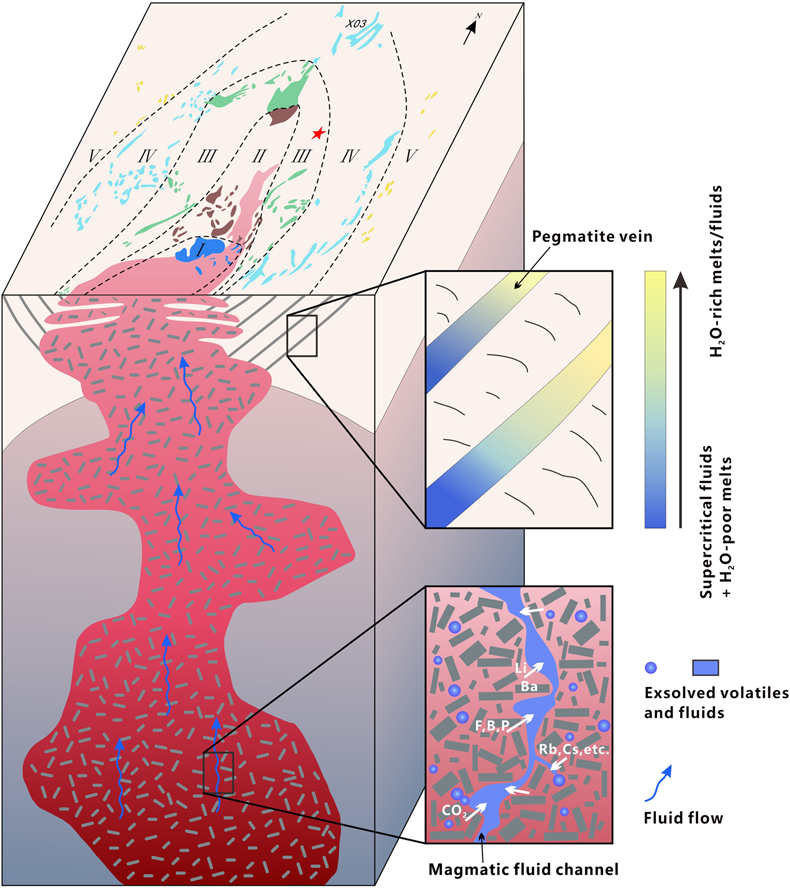
Figure 4 Schematic model for the role of magmatic fluids in intra-crustal material transport and petrogenesis and metallogenesis of granitic pegmatites.