Solubility of calcium carbonate hemihydrate (CCHH): Where does CCHH occur?
Affiliations | Corresponding Author | Cite as | Funding information- Share this article
Article views:616Cumulative count of HTML views and PDF downloads.
- Download Citation
- Rights & Permissions
top
Abstract
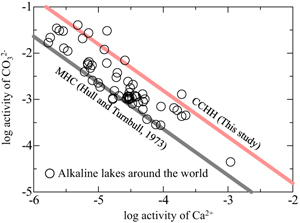
Figures
![]() Figure 1 XRD patterns of the reacted samples as a function of reaction time for (a) Run 1 and (b) Run 2. The integrated XRD pattern with better signal-noise ratio (210 min, Run 2) is shown in detail in Figure S-1. | ![]() Figure 2 (a) pH, (b) alkalinity, (c) Ca concentration, (d) Mg concentration, and (e) log IAP with respect to CaCO3·nH2O as functions of reaction time, and (f) the time derivative of log IAP during the interval between each measurement. Ara, aragonite; Cal, calcite. | ![]() Figure 3 Relationship between the activities of Ca2+ and CO32− in alkaline lakes worldwide (Fukushi and Matsumiya, 2018; Caumartin et al., 2023). Grey and red lines are the solubilities of MHC (log Ksp = −7.65; Hull and Turnbull, 1973) and CCHH (log Ksp = −6.80 ± 0.05; this study), respectively. |
Figure 1 | Figure 2 | Figure 3 |
top
Introduction
Calcium carbonate is ubiquitous on Earth and plays an important role in the geochemical carbon cycle. Calcium carbonates have been widely studied in the fields of palaeoenvironmental reconstruction (Alonso-Zarza, 2003
Alonso-Zarza, A.M. (2003) Palaeoenvironmental significance of palustrine carbonates and calcretes in the geological record. Earth-Science Reviews 60, 261–298. https://doi.org/10.1016/S0012-8252(02)00106-X
), biomineralogy (de Nooijer et al., 2014de Nooijer, L.J., Spero, H.J., Erez, J., Bijma, J., Reichart, G.J. (2014) Biomineralization in perforate foraminifera. Earth-Science Reviews 135, 48–58. https://doi.org/10.1016/j.earscirev.2014.03.013
), and carbon sequestration (Sanna et al., 2014Sanna, A., Uibu, M., Caramanna, G., Kuusik, R., Maroto-Valer, M.M. (2014) A review of mineral carbonation technologies to sequester CO2. Chemical Society Reviews 43, 8049–8080. https://doi.org/10.1039/C4CS00035H
). Six calcium carbonate species have been observed in nature: the three anhydrous polymorphs of CaCO3 (calcite, aragonite, and vaterite), two hydrous species (monohydrocalcite, MHC, CaCO3·H2O; and ikaite, CaCO3·6H2O), and amorphous calcium carbonate (ACC), which is widely recognised as a precursor to the crystalline species (Nielsen et al., 2014Nielsen, M.H., Aloni, S., De Yoreo, J.J. (2014) In situ TEM imaging of CaCO3 nucleation reveals coexistence of direct and indirect pathways. Science 345, 1158–1162. https://doi.org/10.1126/science.1254051
). Although the hydrous calcium carbonates are always metastable phases with short lifetimes in aqueous environments, the formation of these phases play an important role in controlling the chemical composition of the systems (Fukushi and Matsumiya, 2018Fukushi, K., Matsumiya, H. (2018) Control of Water Chemistry in Alkaline Lakes: Solubility of Monohydrocalcite and Amorphous Magnesium Carbonate in CaCl2–MgCl2–Na2CO3 Solutions. ACS Earth and Space Chemistry 2, 735–744. https://doi.org/10.1021/acsearthspacechem.8b00046
; Zeyen et al., 2021Zeyen, N., Benzerara, K., Beyssac, O., Daval, D., Muller, E., et al. (2021) Integrative analysis of the mineralogical and chemical composition of modern microbialites from ten Mexican lakes: What do we learn about their formation? Geochimica et Cosmochimica Acta 305, 148–184. https://doi.org/10.1016/j.gca.2021.04.030
; Tosca and Tutolo, 2023Tosca, N.J., Tutolo, B.M. (2023) How to Make an Alkaline Lake: Fifty Years of Chemical Divides. Elements 19, 15–21. https://doi.org/10.2138/gselements.19.1.15
). A new hydrated calcium carbonate (CaCO3·1/2H2O, calcium carbonate hemihydrate, CCHH) was recently produced in the laboratory (Zou et al., 2019Zou, Z., Habraken, W.J.E.M., Matveeva, G., Jensen, A.C.S., Bertinetti, L., et al. (2019) A hydrated crystalline calcium carbonate phase: Calcium carbonate hemihydrate. Science 363, 396–400. https://doi.org/10.1126/science.aav0210
) from a solution containing Ca2+, Mg2+, and dissolved inorganic carbon (DIC) at relatively high pH and ambient temperature. Although CCHH does not contain Mg in the structure, the Mg2+ in solutions is required for the formation of CCHH because of the dehydration-inhibiting effect of the Mg2+ ion (Zou et al., 2019Zou, Z., Habraken, W.J.E.M., Matveeva, G., Jensen, A.C.S., Bertinetti, L., et al. (2019) A hydrated crystalline calcium carbonate phase: Calcium carbonate hemihydrate. Science 363, 396–400. https://doi.org/10.1126/science.aav0210
; Aufort and Demichelis, 2020Aufort, J., Demichelis, R. (2020) Magnesium Impurities Decide the Structure of Calcium Carbonate Hemihydrate. Crystal Growth & Design 20, 8028–8038. https://doi.org/10.1021/acs.cgd.0c01282
). Such conditions are not uncommon at the Earth’s surface (Drever, 1997Drever, J.I. (1997) The Geochemistry of Natural Waters: Surface and Groundwater Environments. Prentice Hall, New York.
), and CCHH can be expected to occur in nature and may even play an important role in the carbon cycle. However, the natural occurrence of CCHH has not yet been reported. Because a useful criterion for identifying the formation environment of any mineral phases is its solubility, we here report the first laboratory measurements of CCHH solubility and compare our results to the chemistries of natural waters to predict potential formation environments of CCHH in nature. Methods used in this study are described in Supplementary Information.top
Results and Discussion
Evolution of solution chemistry and mineral composition. The solubility of CCHH was investigated by observing the temporal evolution of the solution chemistry and mineralogical compositions in CaCl2–MgCl2–Na2CO3 solutions. Mixed CaCl2–MgCl2 solutions were prepared in a 1 L plastic bottle to which a stock Na2CO3 solution (1 mol/kg) was poured. The initial concentrations of Ca, Mg and DIC were 0.05, 0.06 and 0.05 mol/kg, respectively. The solution condition allowed for CCHH to exist as a persistent phase for relatively long durations of over 5 hours, thus allowing for reliable offline monitoring of the solution chemistry and mineralogical composition. A duplicate batch experiment (Runs 1 and 2) was performed to confirm the reproducibility. During the experiments of both Runs 1 and 2, the major crystalline phases observed by XRD were CCHH and aragonite (Fig. 1). The XRD patterns confirmed the absence of MHC during the experiments (Fig. S-1a) (Swainson, 2008
Swainson, I.P. (2008) The structure of monohydrocalcite and the phase composition of the beachrock deposits of Lake Butler and Lake Fellmongery, South Australia. American Mineralogist 93, 1014–1018. https://doi.org/10.2138/am.2008.2825
; Zou et al., 2019Zou, Z., Habraken, W.J.E.M., Matveeva, G., Jensen, A.C.S., Bertinetti, L., et al. (2019) A hydrated crystalline calcium carbonate phase: Calcium carbonate hemihydrate. Science 363, 396–400. https://doi.org/10.1126/science.aav0210
; Aufort and Demichelis, 2020Aufort, J., Demichelis, R. (2020) Magnesium Impurities Decide the Structure of Calcium Carbonate Hemihydrate. Crystal Growth & Design 20, 8028–8038. https://doi.org/10.1021/acs.cgd.0c01282
). In addition to the dominant phases, small peaks attributed to halite and calcite were observed (Fig. S-2a,b). Because the samples were not washed with the pure water to avoid possible alteration, small peaks of halite (NaCl), which is derived from the dissolved electrolyte in the experiments, were observed in the patterns. The small peak at 29.8° 2θ observed from Run 2 after 480 min came from the strongest peak of calcite. The peak was also observed from Run 1 after 430 min, although it was much smaller than that from Run 2. The position of the peak was close to a peak attributed to CCHH at 29.4° 2θ. A shoulder peak at 29.8° 2θ was commonly associated with the predominant peak of CCHH at 29.4° 2θ in Run 2 (Figs. 1, S-1a), which most likely represents the presence of a small contribution of calcite with CCHH. The intensity of the peak at 29.8° 2θ was invariant with the reaction time (Fig. 1). Therefore, we consider that a small amount of the calcite formed at the initial stage of CCHH formation, but it behaved as a chemically inert material during the subsequent reactions.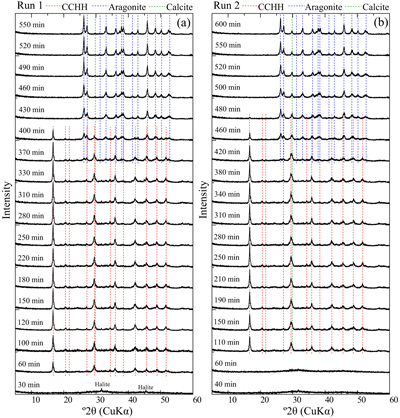
Figure 1 XRD patterns of the reacted samples as a function of reaction time for (a) Run 1 and (b) Run 2. The integrated XRD pattern with better signal-noise ratio (210 min, Run 2) is shown in detail in Figure S-1.
At the beginning of the experiments, no peaks (except for halite) were observed in the XRD patterns except for a broad band at about 30° and 45° 2θ, representing the presence of ACC (Rodriguez-Blanco et al., 2011
Rodriguez-Blanco, J.D., Shaw, S., Benning, L.G. (2011) The kinetics and mechanisms of amorphous calcium carbonate (ACC) crystallization to calcite, via vaterite. Nanoscale 3, 265–271. https://doi.org/10.1039/C0NR00589D
) (Fig. 1). The peak attributable to CCHH (Zou et al., 2019Zou, Z., Habraken, W.J.E.M., Matveeva, G., Jensen, A.C.S., Bertinetti, L., et al. (2019) A hydrated crystalline calcium carbonate phase: Calcium carbonate hemihydrate. Science 363, 396–400. https://doi.org/10.1126/science.aav0210
; Aufort and Demichelis, 2020Aufort, J., Demichelis, R. (2020) Magnesium Impurities Decide the Structure of Calcium Carbonate Hemihydrate. Crystal Growth & Design 20, 8028–8038. https://doi.org/10.1021/acs.cgd.0c01282
) appeared after 60 min (Run 1) and 110 min (Run 2). Figure S-3 shows the changes in the peak intensities of CCHH (16.7° 2θ) and aragonite (26.3° 2θ) with time. The peak intensity of CCHH increased with time, plateauing at maximum values from about 100 to 330 min (Run 1) and 200 to 380 min (Run 2). Peaks attributable to aragonite appeared at 370 min (Run 1) and 420 min (Run 2) and increased in intensity while the CCHH peaks decreased in intensity (Fig. S-3). The intensities of the broad band at around at about 30° 2θ decreased with time for both runs (Fig. S-2a,b). Figure S-2c shows the changes in the averaged intensities from 30.3° to 31.0°, where apparent peaks from crystalline phases were absent. The intensities were initially high before 50 min but dropped suddenly when the CCHH appeared. The intensities were almost at the same level before the appearance of the aragonite until 300–400 min. Then the intensities decreased as the CCHH decreased.At the initial stage of the experiments (up to ∼100 min), the peak intensity of CCHH increased with time (Fig. 1), and the pH and Mg concentration also increased, but the alkalinity and Ca concentration decreased (Fig. 2a–d, Table S-1). This stage represents the formation and growth of CCHH from the precursory ACC, which contains a significant amount of Mg (Loste et al., 2003
Loste, E., Wilson, R.M., Seshadri, R., Meldrum, F.C. (2003) The role of magnesium in stabilising amorphous calcium carbonate and controlling calcite morphologies. Journal of Crystal Growth 254, 206–218. https://doi.org/10.1016/S0022-0248(03)01153-9
). Based on the mass balances of Ca and Mg between the initial concentrations and the solution concentrations before CCHH formation, the molar ratio of Mg/(Ca + Mg) in ACC was calculated to be 20–30 %. The transformation of ACC to CCHH resulted in rapid decreases of the Ca concentration and alkalinity (Fig. 2b,c). During this transformation, Mg in the ACC was released to the solution (Fig. 2d). Indeed, the Mg concentrations in the solutions after the transformation from ACC to CCHH corresponded to the initial (total) Mg concentrations. Based on the mass balances, the molar ratios of Mg/(Ca + Mg) after the Mg release of CCHH were calculated to be 3 ± 4 %. Aufort and Demichelis (2020)Aufort, J., Demichelis, R. (2020) Magnesium Impurities Decide the Structure of Calcium Carbonate Hemihydrate. Crystal Growth & Design 20, 8028–8038. https://doi.org/10.1021/acs.cgd.0c01282
simulated XRD profiles of CCHH as a function of Mg substitution. They showed that the introduction of the Mg impurity causes a distortion in the unit cell. Our CCHH XRD patterns are consistent with their Mg-free content (Fig. S-1b), and the molar ratio of Mg/(Ca + Mg) is at most less than 3 %. This result suggests that Mg barely substituted into the CCHH structure, if at all. The intensities of the broad band at around 30° arising from the presence of ACC decreased with the release of Mg to solution (Fig. S-2c). The intensities took a constant value after the complete Mg release to the solutions before the subsequent transformation of CCHH. The coincidence most likely suggests that the ACC almost completely transformed to CCHH at the initial stage until ∼100 min. Further decreases in intensities at around 30° after ∼350 min coincided exactly with transformation of CCHH. Since ACC must have a different thermodynamic stability from CCHH, the simultaneous transformation of ACC with CCHH is not expected. Therefore, the decreases in XRD background are most likely due to the intrinsic changes of the XRD pattern from CCHH to aragonite without ACC.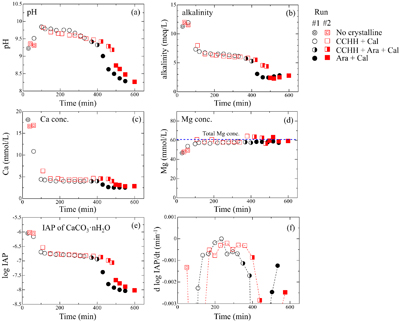
Figure 2 (a) pH, (b) alkalinity, (c) Ca concentration, (d) Mg concentration, and (e) log IAP with respect to CaCO3·nH2O as functions of reaction time, and (f) the time derivative of log IAP during the interval between each measurement. Ara, aragonite; Cal, calcite.
When the CCHH peak intensity plateaued and before the appearance of aragonite peaks, the Ca concentration and alkalinity became relatively constant (Fig. 2b,c). This stage, which lasted almost ∼300 min, most likely represents quasi-equilibrium between CCHH and the solution. After the aragonite peaks appeared at around 400 min, the pH, alkalinity, and Ca concentration suddenly decreased (Fig. 2a,c,d). This stage represents the transformation of CCHH to aragonite. Zou et al. (2019)
Zou, Z., Habraken, W.J.E.M., Matveeva, G., Jensen, A.C.S., Bertinetti, L., et al. (2019) A hydrated crystalline calcium carbonate phase: Calcium carbonate hemihydrate. Science 363, 396–400. https://doi.org/10.1126/science.aav0210
also performed time-resolved synthesis experiments and showed that CCHH formed from ACC after 30 min, and completely transformed to MHC after 120 min. The duration of this transformation herein was significantly longer than in their experiments, probably because of the difference in initial solution conditions (see Supplementary Information).Solubility of CCHH. Figure 2e shows the logarithmic form of the IAP with respect to CaCO3·nH2O. Due to the negligible Mg content in the CCHH (Mg/(Ca + Mg) < 3 %), the contribution of Mg to the mass action expression was ignored in this study. At the beginning of the experiments, log IAP took relatively constant values at around −6.0; it then decreased suddenly to −6.7 by 110 min (Run 1) and 140 min (Run 2), slowly decreased further to −6.9 by 380 min (Run 1) and 410 min (Run 2), and finally decreased suddenly to below −8.0 by 400 min (Run 1) and 480 min (Run 2). These temporal changes of log IAP correspond well to those of the mineral compositions (Fig. 1). The first sudden decrease of log IAP (from −6.0 to −6.7 at around 50–150 min) corresponds to the formation and growth of CCHH from ACC, and the second (from −6.9 to below −8.0 at around 400–500 min) to the formation and growth of aragonite from CCHH (Fig. 1). In the intervening period, log IAP decreased continuously from −6.7 to −6.9, most likely because the solution was first approaching equilibrium with CCHH, which was subsequently followed by aragonite formation. It should be noted that the IAP was always significantly higher than the solubility of calcite (log Ksp = −8.48; Drever, 1997
Drever, J.I. (1997) The Geochemistry of Natural Waters: Surface and Groundwater Environments. Prentice Hall, New York.
), suggesting that the calcite initially formed with CCHH was chemically isolated from the system during the experiments.Theoretically, when a solution approaches equilibrium from a state of oversaturation, the change in log IAP per unit time (i.e. the rate of IAP change) gradually decreases because the kinetics of mineral formation depend on the saturation states (ΔGR) (Nagy and Lasaga, 1992
Nagy, K.L., Lasaga, A.C. (1992) Dissolution and precipitation kinetics of gibbsite at 80°C and pH 3: The dependence on solution saturation state. Geochimica et Cosmochimica Acta 56, 3093–3111. https://doi.org/10.1016/0016-7037(92)90291-P
). In the equilibrium state, the rate of change reaches zero. On the other hand, when a mineral in an (quasi-)equilibrium state begins to transform into a stable mineral, the rate of change must be at a minimum when the transformation begins, and then it gradually increases as the transformation progresses (Fukushi and Matsumiya, 2018Fukushi, K., Matsumiya, H. (2018) Control of Water Chemistry in Alkaline Lakes: Solubility of Monohydrocalcite and Amorphous Magnesium Carbonate in CaCl2–MgCl2–Na2CO3 Solutions. ACS Earth and Space Chemistry 2, 735–744. https://doi.org/10.1021/acsearthspacechem.8b00046
). Therefore, periods in which the rate of IAP change was at a minimum most likely approximate the equilibrium state with respect to CCHH. Figure 2f shows the time derivatives of log IAP during the intervals between measurements for both runs. The rate of change was closest to zero during 220–250 min in Run 1 and 250–280 min in Run 2. The corresponding log IAP values during those intervals were consistent in both runs: −6.79 and −6.80 in Runs 1 and 2, respectively. Based on the possible errors associated with pH, Ca and alkalinity measurements, those log IAP values have maximum uncertainties of ±0.04. Therefore, we estimate the equilibrium constant (solubility product; log Ksp) of CCHH to be −6.80 ± 0.05. This range of log Ksp values includes the observed log IAP values during 150–330 min in Run 1 and 150–380 min in Run 2, when the XRD patterns showed CCHH peaks of constant intensity prior to the appearance of aragonite (Fig. 1). According to the solubility product of CCHH and the Gibbs free energies of formation (ΔGf°) of Ca2+ (−552.79 kJ/mol), CO32− (−527.983 kJ/mol), and H2O (−237.183 kJ/mol) from the thermodynamic database used for our speciation calculations, we calculate ΔGf° to be −1238.2 ± 0.3 kJ/mol for CCHH. In the CaCO3-H2O system at ambient conditions, the thermodynamic stabilities of solid phases increase in the order ACC < ikaite < MHC < vaterite < aragonite < calcite (Hull and Turnbull, 1973Hull, H., Turnbull, A.G. (1973) A thermochemical study of monohydrocalcite. Geochimica et Cosmochimica Acta 37, 685–694. https://doi.org/10.1016/0016-7037(73)90227-5
; Ogino et al., 1987Ogino, T., Suzuki, T., Sawada, K. (1987) The formation and transformation mechanism of calcium carbonate in water. Geochimica et Cosmochimica Acta 51, 2757–2767. https://doi.org/10.1016/0016-7037(87)90155-4
; Brečević and Nielsen, 1989Brečević, L., Nielsen, A.E. (1989) Solubility of amorphous calcium carbonate. Journal of Crystal Growth 98, 504–510. https://doi.org/10.1016/0022-0248(89)90168-1
; Drever, 1997Drever, J.I. (1997) The Geochemistry of Natural Waters: Surface and Groundwater Environments. Prentice Hall, New York.
; Königsberger et al., 1999Königsberger, E., Königsberger, L.-C., Gamsjäger, H. (1999) Low-temperature thermodynamic model for the system Na2CO3−MgCO3−CaCO3−H2O. Geochimica et Cosmochimica Acta 63, 3105–3119. https://doi.org/10.1016/S0016-7037(99)00238-0
; Mergelsberg et al., 2020Mergelsberg, S.T., De Yoreo, J.J., Miller, Q.R.S., Michel, F.M., Ulrich, R.N., Dove, P.M. (2020) Metastable solubility and local structure of amorphous calcium carbonate (ACC). Geochimica et Cosmochimica Acta 289, 196–206. https://doi.org/10.1016/j.gca.2020.06.030
). Our estimated ΔGf° for CCHH suggests that its stability is between those of ikaite and MHC. This stability relationship is consistent with the CCHH transformation pathway observed herein (ACC to CCHH to aragonite) and in Zou et al. (2019Zou, Z., Habraken, W.J.E.M., Matveeva, G., Jensen, A.C.S., Bertinetti, L., et al. (2019) A hydrated crystalline calcium carbonate phase: Calcium carbonate hemihydrate. Science 363, 396–400. https://doi.org/10.1126/science.aav0210
; ACC to CCHH to MHC).Implications for natural occurrences of CCHH. Zou et al. (2019)
Zou, Z., Habraken, W.J.E.M., Matveeva, G., Jensen, A.C.S., Bertinetti, L., et al. (2019) A hydrated crystalline calcium carbonate phase: Calcium carbonate hemihydrate. Science 363, 396–400. https://doi.org/10.1126/science.aav0210
showed that CCHH can form from a solution containing Ca, Mg, and DIC at pH > 10. Here, we explored more moderate conditions, i.e. pH > 9. In nature, the principal aqueous environments rich in calcium, magnesium, and DIC and having high pH are terrestrial alkaline lakes under evaporative conditions (Sekine et al., 2020Sekine, Y., Kitajima, T., Fukushi, K., Gankhurel, B., Tsetsgee, S., et al. (2020) Hydrogeochemical Study on Closed-Basin Lakes in Cold and Semi-Arid Climates of the Valley of the Gobi Lakes, Mongolia: Implications for Hydrology and Water Chemistry of Paleolakes on Mars. Minerals 10, 792. https://doi.org/10.3390/min10090792
; Tosca and Tutolo, 2023Tosca, N.J., Tutolo, B.M. (2023) How to Make an Alkaline Lake: Fifty Years of Chemical Divides. Elements 19, 15–21. https://doi.org/10.2138/gselements.19.1.15
). Most natural alkaline lakes are characterised by high pH (>9) and high Mg and DIC concentrations (Fukushi and Matsumiya, 2018Fukushi, K., Matsumiya, H. (2018) Control of Water Chemistry in Alkaline Lakes: Solubility of Monohydrocalcite and Amorphous Magnesium Carbonate in CaCl2–MgCl2–Na2CO3 Solutions. ACS Earth and Space Chemistry 2, 735–744. https://doi.org/10.1021/acsearthspacechem.8b00046
; Zeyen et al., 2021Zeyen, N., Benzerara, K., Beyssac, O., Daval, D., Muller, E., et al. (2021) Integrative analysis of the mineralogical and chemical composition of modern microbialites from ten Mexican lakes: What do we learn about their formation? Geochimica et Cosmochimica Acta 305, 148–184. https://doi.org/10.1016/j.gca.2021.04.030
). Figure 3 shows the relationship between the activities of Ca2+ and CO32− in alkaline lakes worldwide with pH > 9 and ionic strengths >0.1 (Na-Cl water type; Fukushi and Matsumiya, 2018Fukushi, K., Matsumiya, H. (2018) Control of Water Chemistry in Alkaline Lakes: Solubility of Monohydrocalcite and Amorphous Magnesium Carbonate in CaCl2–MgCl2–Na2CO3 Solutions. ACS Earth and Space Chemistry 2, 735–744. https://doi.org/10.1021/acsearthspacechem.8b00046
; Caumartin et al., 2023Caumartin, J., Benzerara, K., Havas, R., Thomazo, C., Lòpez-García, P., Duprat, E. (2023) The chemical conditions necessary for the formation of microbialites. Geochemical Perspectives Letters 25, 30–35. https://doi.org/10.7185/geochemlet.2311
) (see Supplementary Information and Table S-2). The activities of Ca2+ and CO32− are negatively correlated, suggesting that lake water chemistry is controlled by formation/dissolution processes of calcium carbonates. Indeed, MHC is considered to be a solubility controlling phase in alkaline lakes (Fukushi and Matsumiya, 2018Fukushi, K., Matsumiya, H. (2018) Control of Water Chemistry in Alkaline Lakes: Solubility of Monohydrocalcite and Amorphous Magnesium Carbonate in CaCl2–MgCl2–Na2CO3 Solutions. ACS Earth and Space Chemistry 2, 735–744. https://doi.org/10.1021/acsearthspacechem.8b00046
; Zeyen et al., 2021Zeyen, N., Benzerara, K., Beyssac, O., Daval, D., Muller, E., et al. (2021) Integrative analysis of the mineralogical and chemical composition of modern microbialites from ten Mexican lakes: What do we learn about their formation? Geochimica et Cosmochimica Acta 305, 148–184. https://doi.org/10.1016/j.gca.2021.04.030
; Caumartin et al., 2023Caumartin, J., Benzerara, K., Havas, R., Thomazo, C., Lòpez-García, P., Duprat, E. (2023) The chemical conditions necessary for the formation of microbialites. Geochemical Perspectives Letters 25, 30–35. https://doi.org/10.7185/geochemlet.2311
) based on the ubiquitous presence of MHC in suspended matter and sediments in alkaline lakes in southern Mongolia (Fukushi et al., 2020Fukushi, K., Imai, E., Sekine, Y., Kitajima, T., Gankhurel, B., Davaasuren, D., Hasebe, N. (2020) In Situ Formation of Monohydrocalcite in Alkaline Saline Lakes of the Valley of Gobi Lakes: Prediction for Mg, Ca, and Total Dissolved Carbonate Concentrations in Enceladus’ Ocean and Alkaline-Carbonate Ocean Worlds. Minerals 10, 669. https://doi.org/10.3390/min10080669
; Gankhurel et al., 2022Gankhurel, B., Fukushi, K., Davaasuren, D., Imai, E., Kitajima, T., et al. (2022) Arsenic and uranium contamination of Orog Lake in the Valley of Gobi Lakes, Mongolia: Field evidence of conservative accumulation of U in an alkaline, closed-basin lake during evaporation. Journal of Hazardous Materials 436, 129017. https://doi.org/10.1016/j.jhazmat.2022.129017
). However, the solubility of MHC can explain only the lower limit of the observed trend (grey line in Fig. 3). The red line in Figure 3 represents the solubility of CCHH, which can explain the upper limit of the trend, with 62 of the 68 water chemistry data sets plotting between the solubilities of CCHH and MHC.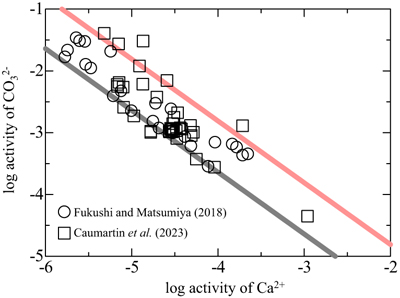
Figure 3 Relationship between the activities of Ca2+ and CO32− in alkaline lakes worldwide (Fukushi and Matsumiya, 2018
Fukushi, K., Matsumiya, H. (2018) Control of Water Chemistry in Alkaline Lakes: Solubility of Monohydrocalcite and Amorphous Magnesium Carbonate in CaCl2–MgCl2–Na2CO3 Solutions. ACS Earth and Space Chemistry 2, 735–744. https://doi.org/10.1021/acsearthspacechem.8b00046
; Caumartin et al., 2023Caumartin, J., Benzerara, K., Havas, R., Thomazo, C., Lòpez-García, P., Duprat, E. (2023) The chemical conditions necessary for the formation of microbialites. Geochemical Perspectives Letters 25, 30–35. https://doi.org/10.7185/geochemlet.2311
). Grey and red lines are the solubilities of MHC (log Ksp = −7.65; Hull and Turnbull, 1973Hull, H., Turnbull, A.G. (1973) A thermochemical study of monohydrocalcite. Geochimica et Cosmochimica Acta 37, 685–694. https://doi.org/10.1016/0016-7037(73)90227-5
) and CCHH (log Ksp = −6.80 ± 0.05; this study), respectively.The present study showed that the log IAP decreased during the transformation of CCHH to aragonite (Fig. 2e), probably because the rate of the CCHH dissolution is lower than that of aragonite formation. If this is the case for the transformation pathway of CCHH to MHC, the lake waters that are experiencing CCHH formation and alteration are most likely plotted below the solubility line of CCHH. On the other hand, Fukushi and Matsumiya (2018)
Fukushi, K., Matsumiya, H. (2018) Control of Water Chemistry in Alkaline Lakes: Solubility of Monohydrocalcite and Amorphous Magnesium Carbonate in CaCl2–MgCl2–Na2CO3 Solutions. ACS Earth and Space Chemistry 2, 735–744. https://doi.org/10.1021/acsearthspacechem.8b00046
, who studied the mineralogical evolution of MHC to aragonite from CaCl2-MgCl2-Na2CO3, showed that the IAP did not decrease during the transformation of MHC to aragonite, probably because the rate of the aragonite formation is lower than that of the MHC dissolution. If this is the case for the transformation pathway of MHC to other anhydrous calcium carbonates, i.e. calcite, then the lake waters containing the MHC (but not containing CCHH) and the alteration products are most likely plotted on the solubility line of MHC. Therefore, the lake waters that are experiencing the CCHH formation and the transformation to MHC must plot between the two lines.In alkaline lakes, evaporation or alternative sources (e.g., groundwater input and CO2 volcanic degassing) increase the saturation state with respect to calcium carbonate. This dynamic process can naturally produce CCHH in alkaline lakes. However, CCHH is a short lived species (this study; Zou et al., 2019
Zou, Z., Habraken, W.J.E.M., Matveeva, G., Jensen, A.C.S., Bertinetti, L., et al. (2019) A hydrated crystalline calcium carbonate phase: Calcium carbonate hemihydrate. Science 363, 396–400. https://doi.org/10.1126/science.aav0210
) that can quickly transform to a stable calcium carbonate species such as MHC under the chemical conditions (i.e. the relationship among Mg, Ca, and DIC in solution) of alkaline lakes. We therefore predict that CCHH is present in alkaline lakes as an initial precipitate during evaporation and exerts an important control on the associated water chemistry. It is solely due to its short lifetime that the presence of CCHH in alkaline lakes has not yet been reported.top
Conclusions
We investigated the solubility of CCHH, a recently discovered calcium carbonate species. The solubility was obtained from offline temporal monitoring of the solution and mineralogical compositions of mixed CaCl2-MgCl2-Na2CO3 solutions. We determined the solubility product (log Ksp) of CCHH to be −6.80 ± 0.05 and its Gibbs free energy of formation to be −1238.2 ± 0.3 kJ/mol, suggesting that the stability of CCHH is between those of ikaite and MHC. The solubility of CCHH corresponds to the upper limit of the saturation levels observed in natural alkaline lakes worldwide. From these results, we predict that CCHH forms naturally, though fleetingly, during evaporation in alkaline lakes.
top
Acknowledgements
This work was supported by Grants-in-Aid for Scientific Research from the Japan Society for Promotion of Science [grant numbers 21K18649, 21H04515 and 24H00268].
Editor: Eric Oelkers
top
References
Alonso-Zarza, A.M. (2003) Palaeoenvironmental significance of palustrine carbonates and calcretes in the geological record. Earth-Science Reviews 60, 261–298. https://doi.org/10.1016/S0012-8252(02)00106-X

Calcium carbonates have been widely studied in the fields of palaeoenvironmental reconstruction (Alonso-Zarza, 2003), biomineralogy (de Nooijer et al., 2014), and carbon sequestration (Sanna et al., 2014).
View in article
Aufort, J., Demichelis, R. (2020) Magnesium Impurities Decide the Structure of Calcium Carbonate Hemihydrate. Crystal Growth & Design 20, 8028–8038. https://doi.org/10.1021/acs.cgd.0c01282

Although CCHH does not contain Mg in the structure, the Mg2+ in solutions is required for the formation of CCHH because of the dehydration-inhibiting effect of the Mg2+ ion (Zou et al., 2019; Aufort and Demichelis, 2020).
View in article
The XRD patterns confirmed the absence of MHC during the experiments (Fig. S-1a) (Swainson, 2008; Zou et al., 2019; Aufort and Demichelis, 2020).
View in article
The peak attributable to CCHH (Zou et al., 2019; Aufort and Demichelis, 2020) appeared after 60 min (Run 1) and 110 min (Run 2).
View in article
Aufort and Demichelis (2020) simulated XRD profiles of CCHH as a function of Mg substitution.
View in article
Brečević, L., Nielsen, A.E. (1989) Solubility of amorphous calcium carbonate. Journal of Crystal Growth 98, 504–510. https://doi.org/10.1016/0022-0248(89)90168-1

In the CaCO3-H2O system at ambient conditions, the thermodynamic stabilities of solid phases increase in the order ACC < ikaite < MHC < vaterite < aragonite < calcite (Hull and Turnbull, 1973; Ogino et al., 1987; Brečević and Nielsen, 1989; Drever, 1997; Königsberger et al., 1999; Mergelsberg et al., 2020).
View in article
Caumartin, J., Benzerara, K., Havas, R., Thomazo, C., Lòpez-García, P., Duprat, E. (2023) The chemical conditions necessary for the formation of microbialites. Geochemical Perspectives Letters 25, 30–35. https://doi.org/10.7185/geochemlet.2311

Figure 3 shows the relationship between the activities of Ca2+ and CO32− in alkaline lakes worldwide with pH > 9 and ionic strengths >0.1 (Na-Cl water type; Fukushi and Matsumiya, 2018; Caumartin et al., 2023) (see Supplementary Information and Table S-2).
View in article
Indeed, MHC is considered to be a solubility controlling phase in alkaline lakes (Fukushi and Matsumiya, 2018; Zeyen et al., 2021; Caumartin et al., 2023) based on the ubiquitous presence of MHC in suspended matter and sediments in alkaline lakes in southern Mongolia (Fukushi et al., 2020; Gankhurel et al., 2022).
View in article
Relationship between the activities of Ca2+ and CO32− in alkaline lakes worldwide (Fukushi and Matsumiya, 2018; Caumartin et al., 2023).
View in article
de Nooijer, L.J., Spero, H.J., Erez, J., Bijma, J., Reichart, G.J. (2014) Biomineralization in perforate foraminifera. Earth-Science Reviews 135, 48–58. https://doi.org/10.1016/j.earscirev.2014.03.013

Calcium carbonates have been widely studied in the fields of palaeoenvironmental reconstruction (Alonso-Zarza, 2003), biomineralogy (de Nooijer et al., 2014), and carbon sequestration (Sanna et al., 2014).
View in article
Drever, J.I. (1997) The Geochemistry of Natural Waters: Surface and Groundwater Environments. Prentice Hall, New York.

Such conditions are not uncommon at the Earth’s surface (Drever, 1997), and CCHH can be expected to occur in nature and may even play an important role in the carbon cycle.
View in article
It should be noted that the IAP was always significantly higher than the solubility of calcite (log Ksp = −8.48; Drever, 1997), suggesting that the calcite initially formed with CCHH was chemically isolated from the system during the experiments.
View in article
In the CaCO3-H2O system at ambient conditions, the thermodynamic stabilities of solid phases increase in the order ACC < ikaite < MHC < vaterite < aragonite < calcite (Hull and Turnbull, 1973; Ogino et al., 1987; Brečević and Nielsen, 1989; Drever, 1997; Königsberger et al., 1999; Mergelsberg et al., 2020).
View in article
Fukushi, K., Matsumiya, H. (2018) Control of Water Chemistry in Alkaline Lakes: Solubility of Monohydrocalcite and Amorphous Magnesium Carbonate in CaCl2–MgCl2–Na2CO3 Solutions. ACS Earth and Space Chemistry 2, 735–744. https://doi.org/10.1021/acsearthspacechem.8b00046

Although the hydrous calcium carbonates are always metastable phases with short lifetimes in aqueous environments, the formation of these phases play an important role in controlling the chemical composition of the systems (Fukushi and Matsumiya, 2018; Zeyen et al., 2021; Tosca and Tutolo, 2023).
View in article
On the other hand, when a mineral in an (quasi-)equilibrium state begins to transform into a stable mineral, the rate of change must be at a minimum when the transformation begins, and then it gradually increases as the transformation progresses (Fukushi and Matsumiya, 2018).
View in article
Most natural alkaline lakes are characterised by high pH (>9) and high Mg and DIC concentrations (Fukushi and Matsumiya, 2018; Zeyen et al., 2021).
View in article
Figure 3 shows the relationship between the activities of Ca2+ and CO32− in alkaline lakes worldwide with pH > 9 and ionic strengths >0.1 (Na-Cl water type; Fukushi and Matsumiya, 2018; Caumartin et al., 2023) (see Supplementary Information and Table S-2).
View in article
Indeed, MHC is considered to be a solubility controlling phase in alkaline lakes (Fukushi and Matsumiya, 2018; Zeyen et al., 2021; Caumartin et al., 2023) based on the ubiquitous presence of MHC in suspended matter and sediments in alkaline lakes in southern Mongolia (Fukushi et al., 2020; Gankhurel et al., 2022).
View in article Fukushi and Matsumiya (2018), who studied the mineralogical evolution of MHC to aragonite from CaCl2-MgCl2-Na2CO3, showed that the IAP did not decrease during the transformation of MHC to aragonite, probably because the rate of the aragonite formation is lower than that of the MHC dissolution.
View in article
Relationship between the activities of Ca2+ and CO32− in alkaline lakes worldwide (Fukushi and Matsumiya, 2018; Caumartin et al., 2023).
View in article
Fukushi, K., Imai, E., Sekine, Y., Kitajima, T., Gankhurel, B., Davaasuren, D., Hasebe, N. (2020) In Situ Formation of Monohydrocalcite in Alkaline Saline Lakes of the Valley of Gobi Lakes: Prediction for Mg, Ca, and Total Dissolved Carbonate Concentrations in Enceladus’ Ocean and Alkaline-Carbonate Ocean Worlds. Minerals 10, 669. https://doi.org/10.3390/min10080669

Indeed, MHC is considered to be a solubility controlling phase in alkaline lakes (Fukushi and Matsumiya, 2018; Zeyen et al., 2021; Caumartin et al., 2023) based on the ubiquitous presence of MHC in suspended matter and sediments in alkaline lakes in southern Mongolia (Fukushi et al., 2020; Gankhurel et al., 2022).
View in article
Gankhurel, B., Fukushi, K., Davaasuren, D., Imai, E., Kitajima, T., et al. (2022) Arsenic and uranium contamination of Orog Lake in the Valley of Gobi Lakes, Mongolia: Field evidence of conservative accumulation of U in an alkaline, closed-basin lake during evaporation. Journal of Hazardous Materials 436, 129017. https://doi.org/10.1016/j.jhazmat.2022.129017

Indeed, MHC is considered to be a solubility controlling phase in alkaline lakes (Fukushi and Matsumiya, 2018; Zeyen et al., 2021; Caumartin et al., 2023) based on the ubiquitous presence of MHC in suspended matter and sediments in alkaline lakes in southern Mongolia (Fukushi et al., 2020; Gankhurel et al., 2022).
View in article
Hull, H., Turnbull, A.G. (1973) A thermochemical study of monohydrocalcite. Geochimica et Cosmochimica Acta 37, 685–694. https://doi.org/10.1016/0016-7037(73)90227-5

In the CaCO3-H2O system at ambient conditions, the thermodynamic stabilities of solid phases increase in the order ACC < ikaite < MHC < vaterite < aragonite < calcite (Hull and Turnbull, 1973; Ogino et al., 1987; Brečević and Nielsen, 1989; Drever, 1997; Königsberger et al., 1999; Mergelsberg et al., 2020).
View in article
Grey and red lines are the solubilities of MHC (log Ksp = −7.65; Hull and Turnbull, 1973) and CCHH (log Ksp = −6.80 ± 0.05; this study), respectively.
View in article
Königsberger, E., Königsberger, L.-C., Gamsjäger, H. (1999) Low-temperature thermodynamic model for the system Na2CO3−MgCO3−CaCO3−H2O. Geochimica et Cosmochimica Acta 63, 3105–3119. https://doi.org/10.1016/S0016-7037(99)00238-0

In the CaCO3-H2O system at ambient conditions, the thermodynamic stabilities of solid phases increase in the order ACC < ikaite < MHC < vaterite < aragonite < calcite (Hull and Turnbull, 1973; Ogino et al., 1987; Brečević and Nielsen, 1989; Drever, 1997; Königsberger et al., 1999; Mergelsberg et al., 2020).
View in article
Loste, E., Wilson, R.M., Seshadri, R., Meldrum, F.C. (2003) The role of magnesium in stabilising amorphous calcium carbonate and controlling calcite morphologies. Journal of Crystal Growth 254, 206–218. https://doi.org/10.1016/S0022-0248(03)01153-9

This stage represents the formation and growth of CCHH from the precursory ACC, which contains a significant amount of Mg (Loste et al., 2003).
View in article
Mergelsberg, S.T., De Yoreo, J.J., Miller, Q.R.S., Michel, F.M., Ulrich, R.N., Dove, P.M. (2020) Metastable solubility and local structure of amorphous calcium carbonate (ACC). Geochimica et Cosmochimica Acta 289, 196–206. https://doi.org/10.1016/j.gca.2020.06.030

In the CaCO3-H2O system at ambient conditions, the thermodynamic stabilities of solid phases increase in the order ACC < ikaite < MHC < vaterite < aragonite < calcite (Hull and Turnbull, 1973; Ogino et al., 1987; Brečević and Nielsen, 1989; Drever, 1997; Königsberger et al., 1999; Mergelsberg et al., 2020).
View in article
Nagy, K.L., Lasaga, A.C. (1992) Dissolution and precipitation kinetics of gibbsite at 80°C and pH 3: The dependence on solution saturation state. Geochimica et Cosmochimica Acta 56, 3093–3111. https://doi.org/10.1016/0016-7037(92)90291-P

Theoretically, when a solution approaches equilibrium from a state of oversaturation, the change in log IAP per unit time (i.e. the rate of IAP change) gradually decreases because the kinetics of mineral formation depend on the saturation states (ΔGR) (Nagy and Lasaga, 1992).
View in article
Nielsen, M.H., Aloni, S., De Yoreo, J.J. (2014) In situ TEM imaging of CaCO3 nucleation reveals coexistence of direct and indirect pathways. Science 345, 1158–1162. https://doi.org/10.1126/science.1254051

Six calcium carbonate species have been observed in nature: the three anhydrous polymorphs of CaCO3 (calcite, aragonite, and vaterite), two hydrous species (monohydrocalcite, MHC, CaCO3·H2O; and ikaite, CaCO3·6H2O), and amorphous calcium carbonate (ACC), which is widely recognised as a precursor to the crystalline species (Nielsen et al., 2014).
View in article
Ogino, T., Suzuki, T., Sawada, K. (1987) The formation and transformation mechanism of calcium carbonate in water. Geochimica et Cosmochimica Acta 51, 2757–2767. https://doi.org/10.1016/0016-7037(87)90155-4

In the CaCO3-H2O system at ambient conditions, the thermodynamic stabilities of solid phases increase in the order ACC < ikaite < MHC < vaterite < aragonite < calcite (Hull and Turnbull, 1973; Ogino et al., 1987; Brečević and Nielsen, 1989; Drever, 1997; Königsberger et al., 1999; Mergelsberg et al., 2020).
View in article
Rodriguez-Blanco, J.D., Shaw, S., Benning, L.G. (2011) The kinetics and mechanisms of amorphous calcium carbonate (ACC) crystallization to calcite, via vaterite. Nanoscale 3, 265–271. https://doi.org/10.1039/C0NR00589D

At the beginning of the experiments, no peaks (except for halite) were observed in the XRD patterns except for a broad band at about 30° and 45° 2θ, representing the presence of ACC (Rodriguez-Blanco et al., 2011) (Fig. 1).
View in article
Sanna, A., Uibu, M., Caramanna, G., Kuusik, R., Maroto-Valer, M.M. (2014) A review of mineral carbonation technologies to sequester CO2. Chemical Society Reviews 43, 8049–8080. https://doi.org/10.1039/C4CS00035H

Calcium carbonates have been widely studied in the fields of palaeoenvironmental reconstruction (Alonso-Zarza, 2003), biomineralogy (de Nooijer et al., 2014), and carbon sequestration (Sanna et al., 2014).
View in article
Sekine, Y., Kitajima, T., Fukushi, K., Gankhurel, B., Tsetsgee, S., et al. (2020) Hydrogeochemical Study on Closed-Basin Lakes in Cold and Semi-Arid Climates of the Valley of the Gobi Lakes, Mongolia: Implications for Hydrology and Water Chemistry of Paleolakes on Mars. Minerals 10, 792. https://doi.org/10.3390/min10090792

In nature, the principal aqueous environments rich in calcium, magnesium, and DIC and having high pH are terrestrial alkaline lakes under evaporative conditions (Sekine et al., 2020; Tosca and Tutolo, 2023).
View in article
Swainson, I.P. (2008) The structure of monohydrocalcite and the phase composition of the beachrock deposits of Lake Butler and Lake Fellmongery, South Australia. American Mineralogist 93, 1014–1018. https://doi.org/10.2138/am.2008.2825

The XRD patterns confirmed the absence of MHC during the experiments (Fig. S-1a) (Swainson, 2008; Zou et al., 2019; Aufort and Demichelis, 2020).
View in article
Tosca, N.J., Tutolo, B.M. (2023) How to Make an Alkaline Lake: Fifty Years of Chemical Divides. Elements 19, 15–21. https://doi.org/10.2138/gselements.19.1.15

In nature, the principal aqueous environments rich in calcium, magnesium, and DIC and having high pH are terrestrial alkaline lakes under evaporative conditions (Sekine et al., 2020; Tosca and Tutolo, 2023).
View in article
Although the hydrous calcium carbonates are always metastable phases with short lifetimes in aqueous environments, the formation of these phases play an important role in controlling the chemical composition of the systems (Fukushi and Matsumiya, 2018; Zeyen et al., 2021; Tosca and Tutolo, 2023).
View in article
Zeyen, N., Benzerara, K., Beyssac, O., Daval, D., Muller, E., et al. (2021) Integrative analysis of the mineralogical and chemical composition of modern microbialites from ten Mexican lakes: What do we learn about their formation? Geochimica et Cosmochimica Acta 305, 148–184. https://doi.org/10.1016/j.gca.2021.04.030

Although the hydrous calcium carbonates are always metastable phases with short lifetimes in aqueous environments, the formation of these phases play an important role in controlling the chemical composition of the systems (Fukushi and Matsumiya, 2018; Zeyen et al., 2021; Tosca and Tutolo, 2023).
View in article
Most natural alkaline lakes are characterised by high pH (>9) and high Mg and DIC concentrations (Fukushi and Matsumiya, 2018; Zeyen et al., 2021).
View in article
Indeed, MHC is considered to be a solubility controlling phase in alkaline lakes (Fukushi and Matsumiya, 2018; Zeyen et al., 2021; Caumartin et al., 2023) based on the ubiquitous presence of MHC in suspended matter and sediments in alkaline lakes in southern Mongolia (Fukushi et al., 2020; Gankhurel et al., 2022).
View in article
Zou, Z., Habraken, W.J.E.M., Matveeva, G., Jensen, A.C.S., Bertinetti, L., et al. (2019) A hydrated crystalline calcium carbonate phase: Calcium carbonate hemihydrate. Science 363, 396–400. https://doi.org/10.1126/science.aav0210

A new hydrated calcium carbonate (CaCO3·1/2H2O, calcium carbonate hemihydrate, CCHH) was recently produced in the laboratory (Zou et al., 2019) from a solution containing Ca2+, Mg2+, and dissolved inorganic carbon (DIC) at relatively high pH and ambient temperature.
View in article
Although CCHH does not contain Mg in the structure, the Mg2+ in solutions is required for the formation of CCHH because of the dehydration-inhibiting effect of the Mg2+ ion (Zou et al., 2019; Aufort and Demichelis, 2020).
View in article
The XRD patterns confirmed the absence of MHC during the experiments (Fig. S-1a) (Swainson, 2008; Zou et al., 2019; Aufort and Demichelis, 2020).
View in article
The peak attributable to CCHH (Zou et al., 2019; Aufort and Demichelis, 2020) appeared after 60 min (Run 1) and 110 min (Run 2).
View in article
Zou et al. (2019) also performed time-resolved synthesis experiments and showed that CCHH formed from ACC after 30 min, and completely transformed to MHC after 120 min.
View in article
This stability relationship is consistent with the CCHH transformation pathway observed herein (ACC to CCHH to aragonite) and in Zou et al. (2019; ACC to CCHH to MHC).
View in article
Zou et al. (2019) showed that CCHH can form from a solution containing Ca, Mg, and DIC at pH > 10.
View in article
However, CCHH is a short lived species (this study; Zou et al., 2019) that can quickly transform to a stable calcium carbonate species such as MHC under the chemical conditions (i.e. the relationship among Mg, Ca, and DIC in solution) of alkaline lakes.
View in article
top
Supplementary Information
The Supplementary Information includes:
- Materials and Methods
- Difference of Lifetime of CCHH Between This Study and Zou et al. (2019)
- Selection of Water Chemistry of Alkaline Lakes in the World
- Tables S-1 and S-2
- Figures S-1 to S-3
- Supplementary Information References
Download the Supplementary Information (PDF)
Download Tables S-1 and S-2 (.xlsx)
Figures
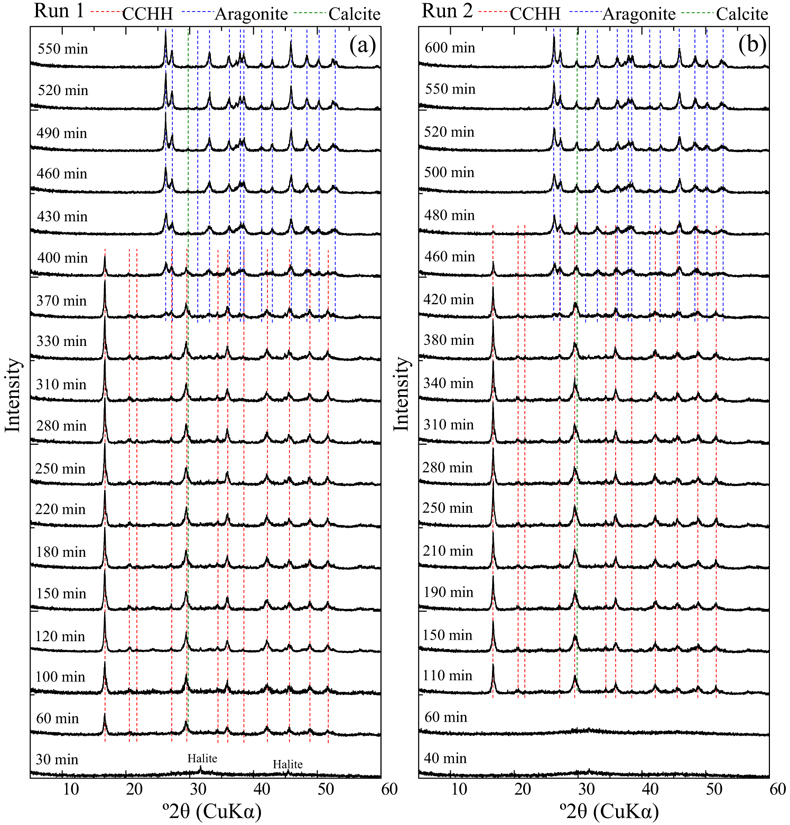
Figure 1 XRD patterns of the reacted samples as a function of reaction time for (a) Run 1 and (b) Run 2. The integrated XRD pattern with better signal-noise ratio (210 min, Run 2) is shown in detail in Figure S-1.
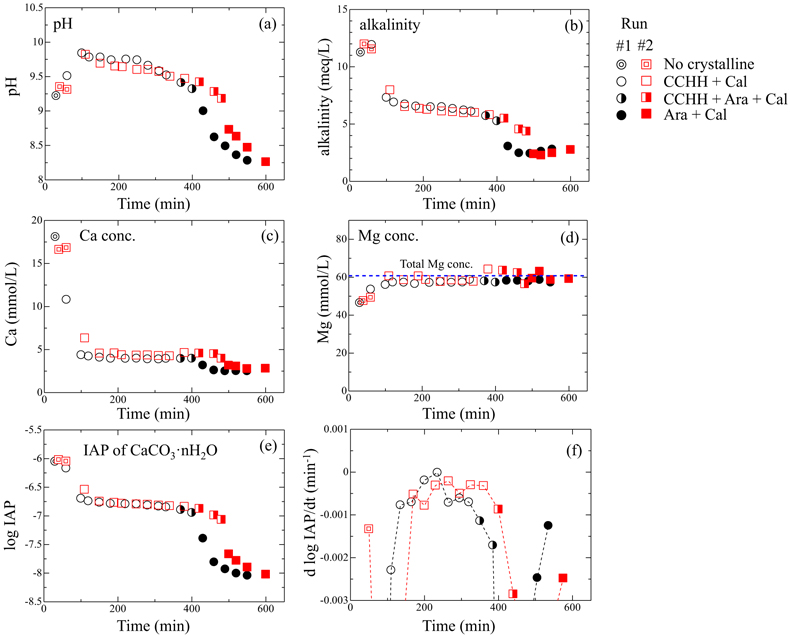
Figure 2 (a) pH, (b) alkalinity, (c) Ca concentration, (d) Mg concentration, and (e) log IAP with respect to CaCO3·nH2O as functions of reaction time, and (f) the time derivative of log IAP during the interval between each measurement. Ara, aragonite; Cal, calcite.
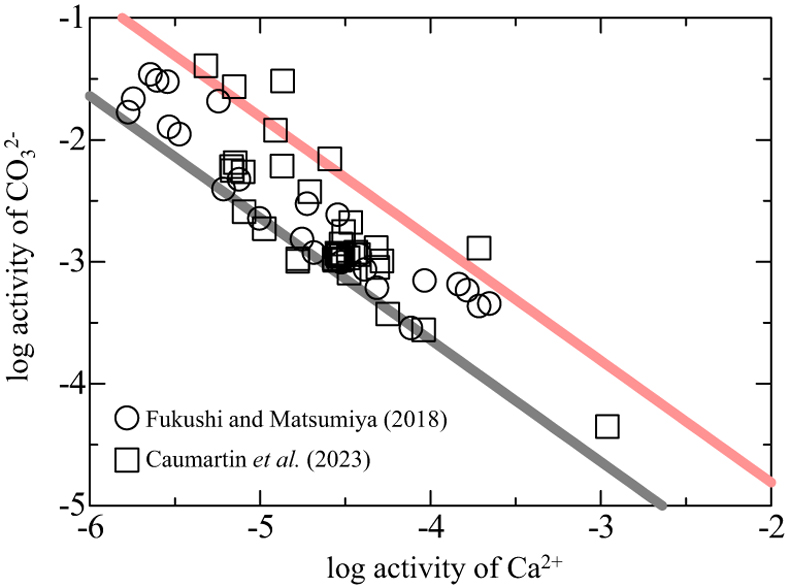
Figure 3 Relationship between the activities of Ca2+ and CO32− in alkaline lakes worldwide (Fukushi and Matsumiya, 2018
Fukushi, K., Matsumiya, H. (2018) Control of Water Chemistry in Alkaline Lakes: Solubility of Monohydrocalcite and Amorphous Magnesium Carbonate in CaCl2–MgCl2–Na2CO3 Solutions. ACS Earth and Space Chemistry 2, 735–744. https://doi.org/10.1021/acsearthspacechem.8b00046
; Caumartin et al., 2023Caumartin, J., Benzerara, K., Havas, R., Thomazo, C., Lòpez-García, P., Duprat, E. (2023) The chemical conditions necessary for the formation of microbialites. Geochemical Perspectives Letters 25, 30–35. https://doi.org/10.7185/geochemlet.2311
). Grey and red lines are the solubilities of MHC (log Ksp = −7.65; Hull and Turnbull, 1973Hull, H., Turnbull, A.G. (1973) A thermochemical study of monohydrocalcite. Geochimica et Cosmochimica Acta 37, 685–694. https://doi.org/10.1016/0016-7037(73)90227-5
) and CCHH (log Ksp = −6.80 ± 0.05; this study), respectively.