Pore water chemical constraints on petrophysical shifts following biosilica diagenesis
Affiliations | Corresponding Author | Cite as | Funding information- Share this article
-
Article views:78Cumulative count of HTML views and PDF downloads.
- Download Citation
- Rights & Permissions
top
Abstract
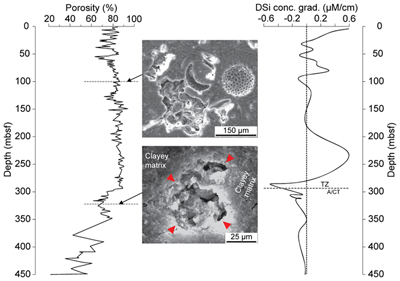
Figures
![]() Figure 1 Integration of mineralogical and petrophysical measurements with seismic profiling, Site 794. (a) Reduced biosilica extents (green area) correlated with rising opal-CT contents (black and red circles), (b,c) anomalous density and porosity changes, and (d) onset of chert/porcellanite production across the TZA/CT at ∼293.5 mbsf are tied to (e) exhibition of a high amplitude reflector ∼0.37 s below the seafloor. The petrophysical profiles were modified from IODP (2014), and the biosilica distribution pattern with depth from Varkouhi et al. (2020a, 2020b). E(y|x)—non-linear regression, s.e.—standard error, Mid. Mioc.—middle Miocene, Stratigr. col.—stratigraphic column. | ![]() Figure 2 Pore water signatures of silica diagenesis at Site 794. (a) DSi profile closely corresponds to opal-A spatial distribution, and (b) pore water undersaturation with biosilica but supersaturation with diagenetic opal, and sediment textural evolution (detailed by cristobalite diffraction intensities and photomicrographs). Refer to Table S-1 for the sample identification. The saturation profiles were modified from Varkouhi et al. (2020b). The volcanic glass depth profile was based upon IODP (2014) smear slide data. α-Crs—α-cristobalite, α-Trd—α-tridymite, An—anorthite, C—clay, Gp—gypsum, Hl—halite, Hul—heulandite, Ill—illite, Opq—opaque, Phg—phengite, Qtz—quartz. | ![]() Figure 3 Pore volume drop due to sediment framework compactional collapse (red arrows) via pore water expulsion, documented by shifts in DSi gradient, oxygen isotopic composition, and temperatures of the TZA/CT sediment; Site 794. The inverse DSi gradient indicates its removal, while highly reduced diffusivities occur, at the TZA/CT. The physical property profiles were modified from IODP (2014). The DSi gradient was estimated as concentration differences through each pore water sampling interval, and the sediment diffusivity as Φ2 × D, where Φ is the porosity and D (5.5 × 10–6 cm2/s; Dixit and Van Cappellen, 2003) the free solution silica diffusivity. The δ18O values used to assess the isotopic temperature of opal-CT (see Table S-2) were computed by following Kita et al. (1985). Amb. temp.—ambient temperature, conc. grad.—concentration gradient, GG—geothermal gradient, Isot. temp.—isotopic temperature. | ![]() Figure 4 Compactional degradation of biosilica to opal-CT in hemipelagic deposits investigated. Silica diagenesis begins chemically near the seabed, but is not expressed as petrophysical changes until the reaction develops through opal-A dissolution and the removal of DSi by vertical/lateral pore water escape. Opal-CT production, retarded by reverse weathering, partially affects the porosity. |
Figure 1 | Figure 2 | Figure 3 | Figure 4 |
top
Introduction
Conventional perspectives on the compaction of deep water deposits deal with petrophysical properties (e.g., porosity, density, and compressional velocity) as gently evolving traits with depth (Wrona et al., 2017a
Wrona, T., Jackson, C.A.-L., Huuse, M., Taylor, K.G. (2017a) Silica diagenesis in Cenozoic mudstones of the North Viking Graben: physical properties and basin modelling. Basin Research 29, 556–575. https://doi.org/10.1111/bre.12168
). Nevertheless, siliceous hemipelagic sediments penetrated during the Deep Sea Drilling Project (DSDP) and Ocean Drilling Program (ODP) have undergone pronounced physical property variations (anomalous compaction) that typically accompany biogenic silica (opal-A) alteration toward opal-CT (α-cristobalite/α-tridymite; Varkouhi et al., 2020aVarkouhi, S., Cartwright, J.A., Tosca, N.J. (2020a) Anomalous compaction due to silica diagenesis: textural and mineralogical evidence from hemipelagic deep-sea sediments of the Japan Sea. Marine Geology 426, 106204. https://doi.org/10.1016/j.margeo.2020.106204
). These petrophysical shifts occur through an early burial dissolution-redeposition process whose development is primarily regulated by temperature and time, with lesser controls from siliceous surface area, host lithofacies, and interstitial water chemistry (Williams et al., 1985Williams, L.A., Parks, G.A., Crerar, D.A. (1985) Silica diagenesis, I. Solubility controls. Journal of Sedimentary Petrology 55, 301–311.
). Prominent opal-A content reductions ascribed to its dissolution, and the subsequent opal-CT precipitation compact the sediment framework and impose a porosity drop on the host lithology (Wrona et al., 2017bWrona, T., Taylor, K.G., Jackson, C.A.-L., Huuse, M., Najorka, J., Pan, I. (2017b) Impact of silica diagenesis on the porosity of fine-grained strata: an analysis of Cenozoic mudstones from the North Sea. Geochemistry, Geophysics, Geosystems 18, 1537–1549. https://doi.org/10.1002/2016GC006482
). Distinct sediment density increases occur as porosity declines because pore volume decreases compress the solid matrix by densifying its constituents (Volpi et al., 2003Volpi, V., Camerlenghi, A., Hillenbrand, C.-D., Rebesco, M., Ivaldi, R. (2003) Effects of biogenic silica on sediment compaction and slope stability on the Pacific margin of the Antarctic Peninsula. Basin Research 15, 339–363. https://doi.org/10.1046/j.1365-2117.2003.00210.x
).The drastic changes in petrophysics of opal-A deposits commonly generate a discrete reflector mainly identifiable on seismic sections when it cross cuts the neighbouring strata (Davies and Cartwright, 2002
Davies, R.J., Cartwright, J. (2002) A fossilized Opal A to Opal C/T transformation on the northeast Atlantic margin: Support for a significantly elevated palaeogeothermal gradient during the Neogene? Basin Research 14, 467–486. https://doi.org/10.1046/j.1365-2117.2002.00184.x
). This profound reflection marks the position of a transition zone, across which biosilica appreciably dissolves and is replaced with opal-CT (TZA/CT; Varkouhi et al., 2020aVarkouhi, S., Cartwright, J.A., Tosca, N.J. (2020a) Anomalous compaction due to silica diagenesis: textural and mineralogical evidence from hemipelagic deep-sea sediments of the Japan Sea. Marine Geology 426, 106204. https://doi.org/10.1016/j.margeo.2020.106204
). The narrow (≤50 m thick) TZA/CT hosts both opal-A and opal-CT, whilst the latter phase dominates (Varkouhi et al., 2021Varkouhi, S., Tosca, N.J., Cartwright, J.A. (2021) Temperature-time relationships and their implications for thermal history and modelling of silica diagenesis in deep-sea sediments. Marine Geology 439, 106541. http://dx.doi.org/10.1016/j.margeo.2021.106541
). Investigations into silica diagenesis and TZA/CT characterisation in sub-seabed sediments have been built upon 2D and 3D seismic stratigraphy, scientific drillings, and on-land surveys (Varkouhi et al., 2022Varkouhi, S., Cartwright, J.A., Tosca, N.J., Papineau, D. (2022) Arrested versus active silica diagenesis reaction boundaries—a review of seismic diagnostic criteria. Basin Research 34, 640–661. https://doi.org/10.1111/bre.12634
). Integration of these procedures with interstitial water chemistry has been applied to the current thermochemical state of biosilica diagenesis across the anomalous compaction section, i.e. active versus fossilised TZA/CT (Varkouhi et al., 2020bVarkouhi, S., Tosca, N.J., Cartwright, J.A. (2020b) Pore water chemistry — A proxy for tracking the signature of ongoing silica diagenesis. Journal of Sedimentary Research 90, 1037–1067. https://doi.org/10.2110/jsr.2020.56
). Following the original works on oxygen isotope relations among opaline polymorphs involved in silica diagenesis (Knauth and Epstein, 1976Knauth, L.P., Epstein, S. (1976) Hydrogen and oxygen isotope ratios in nodular and bedded cherts. Geochimica et Cosmochimica Acta 40, 1095–1108. https://doi.org/10.1016/0016-7037(76)90051-X
; Murata et al., 1977Murata, K.J., Friedman, I., Gleason, J.D. (1977) Oxygen isotope relations between diagenetic silica minerals in Monterey Shale, Temblor Range, California. American Journal of Science 277, 259–272. https://doi.org/10.2475/ajs.277.3.259
; Pisciotto et al., 1992Pisciotto, K.A., Murray, R.W., Brumsack, H.J. (1992) Thermal History of Japan Sea Sediments from Isotopic Studies of Diagenetic Silica and Associated Pore Waters. In: Pisciotto, K.A., Ingle, J.C., Jr., von Breymann, M.T., Barron, J., et al. (Eds.) Proceedings of the Ocean Drilling Program. Scientific Results 127/128 (Pt. 1). Ocean Drilling Program, College Station, Texas, 49–56. https://doi.org/10.2973/odp.proc.sr.127128-1.113.1992
), Yanchinila et al. (2020Yanchilina, A.G., Yam, R., Kolodny, Y., Shemesh, A. (2020) From diatom opal-A δ18O to chert δ18O in deep sea sediments. Geochimica et Cosmochimica Acta 268, 368–382. https://doi.org/10.1016/j.gca.2019.10.018
, 2021)Shipboard Scientific Party (1987) Site 642: Norwegian Sea. In: Eldholm, O., Thiede, J., Taylor, E., et al. (Eds.) Proceedings of the Ocean Drilling Program. Initial Reports 104. Ocean Drilling Program, College Station, Texas, 53–453. https://doi.org/10.2973/odp.proc.ir.104.104.1987
and Ibarra et al. (2022)Ibarra, D.E., Yanchilina, A.G., Lloyd, M.K., Methner, K.A., Chamberlain, C.P., Yam, R., Shemesh, A., Stolper, D.A. (2022) Triple oxygen isotope systematics of diagenetic recrystallization of diatom opal-A to opal-CT to microquartz in deep sea sediments. Geochimica et Cosmochimica Acta 320, 304–323. https://doi.org/10.1016/j.gca.2021.11.027
provided constraints on temperatures of biosilica transformation into opal-CT in deep water cherts using high resolution characterisation of oxygen-18 isotopic compositions. The alteration of opal-A to diagenetic opal (opal-CT) ceases in fossilised transitions, signifying that the TZA/CT is not at the phase predominance boundary between these silica polymorphs (Neagu et al., 2010Neagu, R.C., Cartwright, J., Davies, R.J., Jensen, L. (2010) Fossilisation of a silica diagenesis reaction front on the mid-Norwegian margin. Marine and Petroleum Geology 27, 2141–2155. http://dx.doi.org/10.1016/j.marpetgeo.2010.09.003
). Biosilica diagenesis progresses through an active TZA/CT instead. The view of an active silica diagenesis reaction front is based on the assumption that the TZA/CT has a regional sea bottom simulating geometry, which cross cuts the nearby stratigraphy, and is likely to be presently advancing upwards (Varkouhi et al., 2022Varkouhi, S., Cartwright, J.A., Tosca, N.J., Papineau, D. (2022) Arrested versus active silica diagenesis reaction boundaries—a review of seismic diagnostic criteria. Basin Research 34, 640–661. https://doi.org/10.1111/bre.12634
).Despite research on the TZA/CT structure and thermodynamics (e.g., Davies and Cartwright, 2002
Davies, R.J., Cartwright, J. (2002) A fossilized Opal A to Opal C/T transformation on the northeast Atlantic margin: Support for a significantly elevated palaeogeothermal gradient during the Neogene? Basin Research 14, 467–486. https://doi.org/10.1046/j.1365-2117.2002.00184.x
; Varkouhi et al., 2020bVarkouhi, S., Tosca, N.J., Cartwright, J.A. (2020b) Pore water chemistry — A proxy for tracking the signature of ongoing silica diagenesis. Journal of Sedimentary Research 90, 1037–1067. https://doi.org/10.2110/jsr.2020.56
), the silica dissolution/precipitation kinetics driving petrophysical contrasts between porous opal-A deposits and more condensed opal-CT units is poorly understood. While Varkouhi et al. (2020b)Varkouhi, S., Tosca, N.J., Cartwright, J.A. (2020b) Pore water chemistry — A proxy for tracking the signature of ongoing silica diagenesis. Journal of Sedimentary Research 90, 1037–1067. https://doi.org/10.2110/jsr.2020.56
describe diagenetic states of the TZA/CT using pore fluid chemical proxies, controls from pore water thermodynamics and kinetic modes of ion transport on petrophysics during silica diagenesis are inconclusive in their work. Elucidating the anomalous compaction drivers is crucial to understanding structural/thermal evolution in basin fills, and plays a role in petroleum systems as the TZA/CT contributes to drilling risks by often comprising hard cherts (Neagu et al., 2010Neagu, R.C., Cartwright, J., Davies, R.J., Jensen, L. (2010) Fossilisation of a silica diagenesis reaction front on the mid-Norwegian margin. Marine and Petroleum Geology 27, 2141–2155. http://dx.doi.org/10.1016/j.marpetgeo.2010.09.003
).This manuscript aims to link the sudden compaction of deep marine biosiliceous deposits that have undergone silica diagenesis to processes that transport dissolved phases in pore water through this reaction. To fulfil this objective, signatures of variations in interstitial water composition are combined with records of physical property changes and textural, mineralogical, and oxygen isotopic features of sediments subjected to biosilica diagenesis in Site 794 of the ODP Leg 127, the Japan Sea (Fig. S-1a–c). Interstitial water chemical constraints, including saturation states with silica polymorphs and dissolved silicon (DSi) and major ions concentration gradients indicate that opal-A transformation is active over the TZA/CT in this site and other borehole sites drilling the same transition zone (Varkouhi et al., 2020b
Varkouhi, S., Tosca, N.J., Cartwright, J.A. (2020b) Pore water chemistry — A proxy for tracking the signature of ongoing silica diagenesis. Journal of Sedimentary Research 90, 1037–1067. https://doi.org/10.2110/jsr.2020.56
). The pore fluid chemical variations can map the position of opal-A removal by dissolution and the resultant petrophysical shifts, pointing to where the diagenetic reaction remains active as diffusion is too slow to erase these chemical signals.top
Materials and Methods
Essential data for this work come from the results of interstitial water analyses (Fig. S-2) and physical property measurements for Site 794 available through the International Ocean Discovery Program (IODP) database. Complementary mineralogical, textural, and chemical constraints were furnished with analyses of cored samples from this site. Optical petrography was conducted on smear slides with a Leica DM750 P microscope to describe the sediment biogenic and lithogenic components. Abundances of opal-CT were determined from X-ray diffraction (XRD) scans acquired using a PANalytical Empyrean diffractometer. The software Match! 3.16 identified the crystalline phases and determined their abundance in the sediments. The Reference Intensity Ratio (RIR) method, which scales diffraction data to the diffraction of reference material (corundum), was employed to quantify crystalline components in the samples with multiple phases. Distribution of frustules in the sediment, their dissolution and re-precipitation as opal-CT, and fabric evolution were characterised under a FEI Quanta 650 scanning electron microscope (SEM). Palaeo-temperatures of sediments with precipitated opal-CT were derived from δ18O values to trace opal-A inversion toward opal-CT through the anomalously compacted sediment interval. The operating procedures for these methods are detailed in the Supplementary Information.
top
Mineralogical and Textural Make-Up
Biosilica, mainly diatoms, is the sole major biogenic component, while clay-sized materials constitute the leading siliciclastic component of the sediment sequence in Site 794 (Fig. S-3a–e, b–h). The relative extent of opal-A assessed using visual estimation scheme of Marsaglia et al. (2013)
Marsaglia, K.M., Milliken, K., Doran, L. (2013) Smear Slides of Marine Mud for IODP Core Description: Part I Methodology and Atlas of Siliciclastic and Volcanogenic Components. International Ocean Discovery Program Technical Note 1, 263p. https://doi.org/10.2204/iodp.tn.1.2013
records a peak of 72 % at ∼100 mbsf for a diatom ooze, mixed with fine volcanic glass, sealing the TZA/CT reflector of petrophysical changes (Figs. 1a–e, 2a and S-3a). The clayey fraction averaged at ∼58 % in the opal-A sediment reaches 93 % within the opal-CT pile. The relative abundance of opal-CT was identified by peak intensities of planar spacing (d) (101) α-cristobalite at 26.18–25.14° 2θ and d(110) α-tridymite grid at 23.98° 2θ in the XRD record of erratically aligned bulk samples (Fig. 2b). The XRD peaks of clayey and fine volcanic materials in multi-phase sediments overprint the diffraction signs of biosilica; making opal-A undetectable using this process. The intensity-depth profile of α-cristobalite as the opal-CT proxy (Damby et al., 2014Damby, D.E., Llewellin, E.W., Horwell, C.J., Williamson, B.J., Najorka, J., Cressey, G., Carpenter, M.A. (2014) The α–β phase transition in volcanic cristobalite. Journal of Applied Crystallography 47, 1205–1215. https://doi.org/10.1107/S160057671401070X
) displays the initial formation of this phase in the sequence over the TZA/CT as a weak intensity diffraction set, while its intensity in the TZA/CT largely varies between 0 and 10260 cts/s (Fig. 2b). The XRD detected normalised quantities of α-cristobalite vary between ∼2 and ∼27 wt. % and from 3.5 to ∼31 wt. % for α-tridymite, with a mean value rise in the opal-CT-rich section to nearly three times that of the opal-A unit (Table S-1). The percentage of anorthite drops when shifting from the opal-A succession to the TZA/CT, but the mean quartz and phengite contents in the opal-CT pile remain close to that of the opal-A section. The siliceous textures in Site 794 exhibit a change in diatom content of the sediment with depth from significant to none (Fig. S-4a–h). The siliceous frustules are largely fragmented and dissolved even at shallow burial depths (Figs. 2a,b, S-4a), and their dissolution degree varies from moderately dissolved (for depths near the seabed) to low close to the base of opal-A interval (Fig. S-4b–e). The microfabric within the TZA/CT and its underlying unit is featured by rather perfect biosilica dissolution and its replacement by growth of opal-CT lepispheres in a condensed clayey matrix with a reduced porosity (Fig. S-4f–h).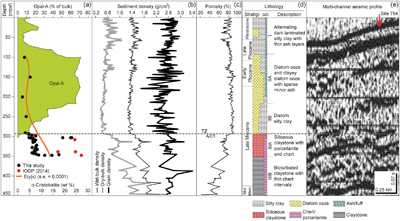
Figure 1 Integration of mineralogical and petrophysical measurements with seismic profiling, Site 794. (a) Reduced biosilica extents (green area) correlated with rising opal-CT contents (black and red circles), (b,c) anomalous density and porosity changes, and (d) onset of chert/porcellanite production across the TZA/CT at ∼293.5 mbsf are tied to (e) exhibition of a high amplitude reflector ∼0.37 s below the seafloor. The petrophysical profiles were modified from IODP (2014)
International Ocean Discovery Program (IODP) (2014) Retrieved from http://web.iodp.tamu.edu/
, and the biosilica distribution pattern with depth from Varkouhi et al. (2020aVarkouhi, S., Cartwright, J.A., Tosca, N.J. (2020a) Anomalous compaction due to silica diagenesis: textural and mineralogical evidence from hemipelagic deep-sea sediments of the Japan Sea. Marine Geology 426, 106204. https://doi.org/10.1016/j.margeo.2020.106204
, 2020b)Varkouhi, S., Tosca, N.J., Cartwright, J.A. (2020b) Pore water chemistry — A proxy for tracking the signature of ongoing silica diagenesis. Journal of Sedimentary Research 90, 1037–1067. https://doi.org/10.2110/jsr.2020.56
. E(y|x)—non-linear regression, s.e.—standard error, Mid. Mioc.—middle Miocene, Stratigr. col.—stratigraphic column.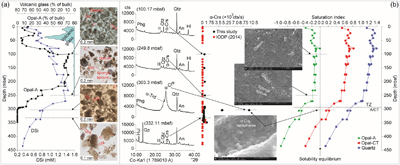
Figure 2 Pore water signatures of silica diagenesis at Site 794. (a) DSi profile closely corresponds to opal-A spatial distribution, and (b) pore water undersaturation with biosilica but supersaturation with diagenetic opal, and sediment textural evolution (detailed by cristobalite diffraction intensities and photomicrographs). Refer to Table S-1 for the sample identification. The saturation profiles were modified from Varkouhi et al. (2020b)
Varkouhi, S., Tosca, N.J., Cartwright, J.A. (2020b) Pore water chemistry — A proxy for tracking the signature of ongoing silica diagenesis. Journal of Sedimentary Research 90, 1037–1067. https://doi.org/10.2110/jsr.2020.56
. The volcanic glass depth profile was based upon IODP (2014)International Ocean Discovery Program (IODP) (2014) Retrieved from http://web.iodp.tamu.edu/
smear slide data. α-Crs—α-cristobalite, α-Trd—α-tridymite, An—anorthite, C—clay, Gp—gypsum, Hl—halite, Hul—heulandite, Ill—illite, Opq—opaque, Phg—phengite, Qtz—quartz.top
Ionic Transport Modes in Biogenic Silica Diagenesis
Biogenic silica diagenesis in Site 794 has crucially governed the pore water chemistry, particularly the gradient of major ions (Figs. 2a, 3 and S-2). Notable DSi and lithium releases to the interstitial water above, and removal from the pore solution in the TZA/CT, imply that this transition behaves as a diffuse boundary that develops concentration gradients downwards below it and upwards into the ocean water (Varkouhi et al., 2020b
Varkouhi, S., Tosca, N.J., Cartwright, J.A. (2020b) Pore water chemistry — A proxy for tracking the signature of ongoing silica diagenesis. Journal of Sedimentary Research 90, 1037–1067. https://doi.org/10.2110/jsr.2020.56
; Figs. 2a, S-2). Because the sediment diffusivity changes with burial (Fig. 3), the profiles linking ionic concentrations above and below the TZA/CT are non-linear. The burial depth reliance of diffusivity is expressed by its connection to pore structures (Yong et al., 2014Yong, Y., Lou, X., Li, S., Yang, C., Yin, X. (2014) Direct simulation of the influence of the pore structure on the diffusion process in porous media. Computers and Mathematics with Applications 67, 412–423, http://dx.doi.org/10.1016/j.camwa.2013.08.032
), indicating the more reduced diffusion in lower porosity opal-CT sediments than in biosiliceous deposits (Fig. 3).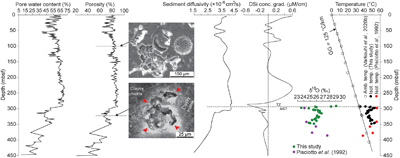
Figure 3 Pore volume drop due to sediment framework compactional collapse (red arrows) via pore water expulsion, documented by shifts in DSi gradient, oxygen isotopic composition, and temperatures of the TZA/CT sediment; Site 794. The inverse DSi gradient indicates its removal, while highly reduced diffusivities occur, at the TZA/CT. The physical property profiles were modified from IODP (2014)
International Ocean Discovery Program (IODP) (2014) Retrieved from http://web.iodp.tamu.edu/
. The DSi gradient was estimated as concentration differences through each pore water sampling interval, and the sediment diffusivity as Φ2 × D, where Φ is the porosity and D (5.5 × 10–6 cm2/s; Dixit and Van Cappellen, 2003Dixit, S., Van Cappellen, P. (2003) Predicting benthic fluxes of silicic acid from deep-sea sediments. Journal of Geophysical Research: Oceans 108 (C10), 3334. http://dx.doi.org/10.1029/2002JC001309
) the free solution silica diffusivity. The δ18O values used to assess the isotopic temperature of opal-CT (see Table S-2) were computed by following Kita et al. (1985)Kita, I., Taguchi, S., Matsubaya, O. (1985) Oxygen isotope fractionation between amorphous silica and water at 34°-93°C. Nature 314, 83–84. https://doi.org/10.1038/314083a0
. Amb. temp.—ambient temperature, conc. grad.—concentration gradient, GG—geothermal gradient, Isot. temp.—isotopic temperature.The exceedingly low permeability of biosiliceous deposits (5.35 × 10–20 to 1.97 × 10–14 m2; Holler, 1992
Holler, P.R. (1992) Consolidation Characteristics and Permeabilities of Sediments from the Japan Sea (Sites 798 and 799) In: Tamaki, K., Suyehiro, K., Allan, J., McWilliams, M., et al. (Eds.) Proceedings of the Ocean Drilling Program. Scientific Results 127/128 (Pt. 2). Ocean Drilling Program, College Station, Texas, 1123–1133. https://doi.org/10.2973/odp.proc.sr.127128-2.232.1992
) contributes to their greatly lowered sediment diffusivity with depth, especially in the TZA/CT and deeper deposits at Site 794 (Fig. 3). Consequently, diffusive flows are negligible drivers for DSi removal from the pore water and bypassing of opal-CT documented across the TZA/CT (Figs. 1a, 2a,b).Conversely, a water content depletion coincident with the porosity shifts from biosilica oozes to opal-CT sediments (Fig. 3) supports the argument that DSi and other ions have not diffused in the sediment at rates comparable to those by advective ion transport modes. The opal-CT-rich sediment is thus more compacted than opal-A deposits because its transformation from biosilica involves pore volume reduction when pore water is expelled. The low permeable overburden sealing the TZA/CT may impede the interstitial fluid expulsion because advection depends on higher permeability rather than diffusive fluxes (Kwon et al., 2004
Kwon, O., Mobley, K., Carlson, R.L. (2004) Measured permeabilities of diatomaceous sediments and pelagic clay from the northwest Pacific, ODP Site 1179. In: Sager, W.W., Kanazawa, T., Escutia, C., (Eds.) Proceedings of the Ocean Drilling Program. Scientific Results 191. Ocean Drilling Program, College Station, Texas, 1–16. http://dx.doi.org/10.2973/odp.proc.sr.191.005.2004
). However, the pore fluid release when opal-A converts into opal-CT is influenced by over pressure, and the low permeability biosilica section above the TZA/CT allows elevation and perpetuation of the over pressure that drives fluid outfluxes (Davies et al., 2008Davies, R.J., Goulty, N.R., Meadows, D. (2008) Fluid flow due to the advance of basin-scale silica reaction zones. Geological Society of America Bulletin 120, 195–206. https://doi.org/10.1130/b26099.1
).top
Thermodynamic Indications of Active Silica Diagenesis
The ongoing opal-CT production is evident from highly undersaturated pore water state with biosilica while supersaturated with diagenetic silica polymorphs, including opal-CT and quartz along the sediment unit and the TZA/CT in Site 794 (Figs. 1a, 2b). Consequently, biosilica dissolves throughout the sediment section, but synchronously the opal-CT supersaturated pore fluid in the biosiliceous unit reaches solubility equilibrium with this phase in the TZA/CT (Fig. 2b). The solubility control is thus reached with opal-CT and yet this polymorph precipitates across the TZA/CT, where it has been largely produced since the late Miocene onset of the transition advancement (Varkouhi et al., 2021
Varkouhi, S., Tosca, N.J., Cartwright, J.A. (2021) Temperature-time relationships and their implications for thermal history and modelling of silica diagenesis in deep-sea sediments. Marine Geology 439, 106541. http://dx.doi.org/10.1016/j.margeo.2021.106541
).This further argues for dominance of pore fluid advection with minor influences from diffusion across the interval of significant opal-CT production. The TZA/CT coincides with the depth of DSi sink, where aqueous DSi leaves the pore water system through huge volumes of the interstitial water expelled, and thus a porosity decrease (Figs. 2a, 3). Co-occurrence of the DSi sink, the sharp physical property variations, and marked opal-CT production in Site 794 TZA/CT (Figs. 1a–c, 2a,b) demonstrates that this DSi drop is essentially governed by active biosilica diagenesis. Under slow hemipelagic sedimentation (1–104 m/Myr; Varkouhi et al., 2021
Varkouhi, S., Tosca, N.J., Cartwright, J.A. (2021) Temperature-time relationships and their implications for thermal history and modelling of silica diagenesis in deep-sea sediments. Marine Geology 439, 106541. http://dx.doi.org/10.1016/j.margeo.2021.106541
), advection and diffusion potentially erase signatures of non-steady state DSi concentration drops unless diagenesis still continues or occurred recently (the 10–50 m/Myr accumulation rate in Site 794 falls within slow rate ranges; Shipboard Scientific Party, 1990Shipboard Scientific Party (1990) Site 794. In: Tamaki, K., Pisciotto, K., Allan, J., et al. (Eds.) Proceedings of the Ocean Drilling Program. Initial Reports 127. Ocean Drilling Program, College Station, Texas, 71–167. https://doi.org/10.2973/odp.proc.ir.127.104.1990
). If solubility equilibrium with opal-CT and its production had ceased at Site 794, the late Miocene chemical signals of the last phase of silica diagenesis would have been erased in the present concentration profile under advective or even highly reduced diffusive rates.The solubility equilibration at the boundary of diagenetic silica formation from dissolved opal-A conforms to temperature increases with depth as the assessment of saturation state involves temperature constraints. Hence, opal-CT equilibrates with the pore water as temperature increases with burial, highly suggesting that active opal-CT production in Site 794 links to the present day thermal gradient (Fig. 3).
Clustering of isotopic temperatures for the TZA/CT sediment section and its underlying unit, extracted from the opal-CT δ18O using the fractionation relation of Kita et al. (1985)
Kita, I., Taguchi, S., Matsubaya, O. (1985) Oxygen isotope fractionation between amorphous silica and water at 34°-93°C. Nature 314, 83–84. https://doi.org/10.1038/314083a0
, near the present temperatures (Fig. 3, Table S-2) supports the notion that biosilica diagenesis has thermodynamically equilibrated with the modern thermal gradient at Site 794 (Pisciotto et al., 1992Pisciotto, K.A., Murray, R.W., Brumsack, H.J. (1992) Thermal History of Japan Sea Sediments from Isotopic Studies of Diagenetic Silica and Associated Pore Waters. In: Pisciotto, K.A., Ingle, J.C., Jr., von Breymann, M.T., Barron, J., et al. (Eds.) Proceedings of the Ocean Drilling Program. Scientific Results 127/128 (Pt. 1). Ocean Drilling Program, College Station, Texas, 49–56. https://doi.org/10.2973/odp.proc.sr.127128-1.113.1992
). Accordingly, the lack of signatures of sudden δ18O changes with depth in the opal-CT-rich interval, i.e. a ∼5.5 ‰ range of δ18O variations maintained throughout the section (Fig. 3), implies that diagenetic opal has acquired these δ18O records via equilibrium with its solubility in the pore water; an indication for the presently active state of the Site 794 TZA/CT.top
Anomalous Compaction in Biosilica Deposits - A Synoptic Model
The chemical transition from biosilica to opal-CT concomitant with a porosity drop of ∼15 % (from ∼80 to ∼65 %; Fig. 1c) in the TZA/CT at Site 794 is closely analogous to those formerly approximated for hemipelagic oozes from geographically diverse basins. For instance, biosilica diagenesis has led to 16 % pore volume reduction in the early Miocene sediment of Norwegian Sea (Shipboard Scientific Party, 1987
Shipboard Scientific Party (1987) Site 642: Norwegian Sea. In: Eldholm, O., Thiede, J., Taylor, E., et al. (Eds.) Proceedings of the Ocean Drilling Program. Initial Reports 104. Ocean Drilling Program, College Station, Texas, 53–453. https://doi.org/10.2973/odp.proc.ir.104.104.1987
) and 15 % in the Neogene claystones of New Jersey Atlantic continental slope (Shipboard Scientific Party, 1994Shipboard Scientific Party (1994) Site 902. In: Mountain, G.S., Miller, K.G., Blum, P., et al. (Eds.) Proceedings of the Ocean Drilling Program. Initial Reports 150. Ocean Drilling Program, College Station, Texas, 63–127. https://doi.org/10.2973/odp.proc.ir.150.106.1994
). The porosity decline due to opal-A dissolution and consumption underlined here and in comparable works corresponds with other petrophysical shifts, e.g., density and velocity increases and pore water release (Figs. 1b, 3). The association of these petrophysical contrasts with silica diagenetic transformation is described by a conceptual model shown in Figure 4.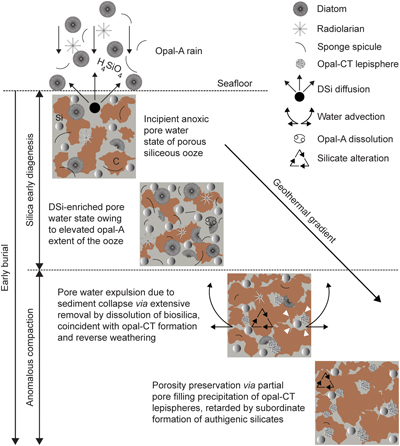
Figure 4 Compactional degradation of biosilica to opal-CT in hemipelagic deposits investigated. Silica diagenesis begins chemically near the seabed, but is not expressed as petrophysical changes until the reaction develops through opal-A dissolution and the removal of DSi by vertical/lateral pore water escape. Opal-CT production, retarded by reverse weathering, partially affects the porosity.
Following our model, under oxygen depleted conditions, the pore water DSi extent has been continuously fed by dissolution of biosilica buried below the seabed. Despite low sedimentation rates in Site 794, elevated primary productivity has been able to sustain a continuous opal-A rain to the seabed, and hence is proportional to biosilica burial and DSi recycling (von Breymann et al., 1992
von Breymann, M.T., Brumsack, H., Emeis, K.C. (1992) Depositional and diagenetic behaviour of barium in the Japan Sea. In: Pisciotto, K.A., Ingle, J.C., Jr., von Breymann, M.T., Barron, J., et al. (Eds.) Proceedings of the Ocean Drilling Program. Scientific Results 127/128 (Pt. 1). Ocean Drilling Program, College Station, Texas, 651–665. https://doi.org/10.2973/odp.proc.sr.127128-1.168.1992
). The volcaniclastics (e.g., volcanic glass) admixed with biosilica may impart DSi to the pore fluid in quantities near those of opal-A dissolution. The low volcaniclastic content of the biosilica sequence and its negligible distribution in the TZA/CT however, display notably less DSi release to the pore solution through volcanic glass alteration than biosilica dissolution (Fig. 2a; Varkouhi et al., 2020bVarkouhi, S., Tosca, N.J., Cartwright, J.A. (2020b) Pore water chemistry — A proxy for tracking the signature of ongoing silica diagenesis. Journal of Sedimentary Research 90, 1037–1067. https://doi.org/10.2110/jsr.2020.56
).The increasing DSi level with depth in the siliceous ooze implies the onset of biosilica early diagenesis (Fig. 2a), but is not expressed as pore fluid expulsion and porosity decreases until the reaction is complete via pore water DSi removal and subordinate opal-CT formation (Fig. 4). The anomalous compressional changes when opal-A undergoes appreciable dissolution happen abruptly (Wrona et al., 2017b
Wrona, T., Taylor, K.G., Jackson, C.A.-L., Huuse, M., Najorka, J., Pan, I. (2017b) Impact of silica diagenesis on the porosity of fine-grained strata: an analysis of Cenozoic mudstones from the North Sea. Geochemistry, Geophysics, Geosystems 18, 1537–1549. https://doi.org/10.1002/2016GC006482
). Disintegration and significant dissolution of biosilica destabilise the sediment structure and lead to the collapse of intergranular matrix (Fig. 3). Elevated pressures generated during opal-A dissolution and sediment compaction, and sustained by low permeability of clay-rich overburden, drive pore water escape from the compacted opal-CT sediment (Fig. 4).Although opal-CT cements in the sediment fabric affect the porosity (Fig. S-4f–h), their impact on pore volume decrease is subordinate to precursor opal-A dissolution (Wrona et al., 2017b
Wrona, T., Taylor, K.G., Jackson, C.A.-L., Huuse, M., Najorka, J., Pan, I. (2017b) Impact of silica diagenesis on the porosity of fine-grained strata: an analysis of Cenozoic mudstones from the North Sea. Geochemistry, Geophysics, Geosystems 18, 1537–1549. https://doi.org/10.1002/2016GC006482
). This can be attributed to authigenesis of aluminosilicates, i.e. reverse weathering (Krissansen-Totton and Catling, 2020Krissansen-Totton, J., Catling, D.C. (2020) A coupled carbon-silicon cycle model over Earth history: Reverse weathering as a possible explanation of a warm mid-Proterozoic climate. Earth and Planetary Science Letters 537, 116181. https://doi.org/10.1016/j.epsl.2020.116181
), which is associated with opal-CT production in deep marine deposits. These reactions compete with opal-A diagenesis for uptake of some ions, as evidenced by Si, Li, K, and Mg removal from interstitial waters in Site 794 TZA/CT, but Ca and Sr additions through silicate alteration (Fig. S-2). Opal-CT precipitates in the TZA/CT, but its solubility equilibrium is slowly reached across this boundary (Fig. 2b) likely because of the secondary clay/zeolite formation. Reverse weathering restricts massive opal-CT cementation in the anomalous compaction interval, so that the diagenetic opal sediment retains appreciable porosities (∼65 % in average; Figs. 1c, 3). The retardation in silica diagenesis by secondary silicate formation is barely assisted by the precipitation of minor opaque minerals, pyrite, and gypsum (Figs. S-3a–h, S-4d).top
Synthesis
In line with recent research on geochemistry of transitions from biogenic to diagenetic opal, this study developed chemical criteria for thermodynamic state of silica diagenesis at pore fluid scales. This high resolution approach relates the anomalous petrophysical shifts across the TZA/CT in Site 794 to pore fluid variations of DSi and major ions, oxygen-18 composition of opal-CT, and saturation states with biogenic and diagenetic silica. Accordingly, the transformation of opal-CT from biosilica has not been fossilised since the late Miocene initiation of the advance of petrophysical TZA/CT at this site, as pore fluid advection (and diffusion) has not erased the signatures of active diagenesis. Given the implication of anomalous compaction dimensions for basin evolution future thermodynamic research may construct more inclusive pictures showing how silica diagenesis modifies the petrophysics of sediments in other basins of different thermal and geochemical histories.
top
Acknowledgements
This study used data derived from the ODP and IODP database and the IODP provided core samples. We would like to thank the two anonymous reviewers, whose comments greatly improved the paper, and Claudine Stirling for her work as editor.
Editor: Claudine Stirling
top
References
Damby, D.E., Llewellin, E.W., Horwell, C.J., Williamson, B.J., Najorka, J., Cressey, G., Carpenter, M.A. (2014) The α–β phase transition in volcanic cristobalite. Journal of Applied Crystallography 47, 1205–1215. https://doi.org/10.1107/S160057671401070X

The XRD peaks of clayey and fine volcanic materials in multi-phase sediments overprint the diffraction signs of biosilica; making opal-A undetectable using this process. The intensity-depth profile of α-cristobalite as the opal-CT proxy (Damby et al., 2014) displays the initial formation of this phase in the sequence over the TZA/CT as a weak intensity diffraction set, while its intensity in the TZA/CT largely varies between 0 and 10260 cts/s (Fig. 2b).
View in article
Davies, R.J., Cartwright, J. (2002) A fossilized Opal A to Opal C/T transformation on the northeast Atlantic margin: Support for a significantly elevated palaeogeothermal gradient during the Neogene? Basin Research 14, 467–486. https://doi.org/10.1046/j.1365-2117.2002.00184.x

The drastic changes in petrophysics of opal-A deposits commonly generate a discrete reflector mainly identifiable on seismic sections when it cross cuts the neighbouring strata (Davies and Cartwright, 2002).
View in article
Despite research on the TZA/CT structure and thermodynamics (e.g., Davies and Cartwright, 2002; Varkouhi et al., 2020b), the silica dissolution/precipitation kinetics driving petrophysical contrasts between porous opal-A deposits and more condensed opal-CT units is poorly understood.
View in article
Davies, R.J., Goulty, N.R., Meadows, D. (2008) Fluid flow due to the advance of basin-scale silica reaction zones. Geological Society of America Bulletin 120, 195–206. https://doi.org/10.1130/b26099.1

However, the pore fluid release when opal-A converts into opal-CT is influenced by over pressure, and the low permeability biosilica section above the TZA/CT allows elevation and perpetuation of the over pressure that drives fluid outfluxes (Davies et al., 2008).
View in article
Dixit, S., Van Cappellen, P. (2003) Predicting benthic fluxes of silicic acid from deep-sea sediments. Journal of Geophysical Research: Oceans 108 (C10), 3334. https://dx.doi.org/10.1029/2002JC001309

The DSi gradient was estimated as concentration differences through each pore water sampling interval, and the sediment diffusivity as Φ2 × D, where Φ is the porosity and D (5.5 × 10–6 cm2/s; Dixit and Van Cappellen, 2003) the free solution silica diffusivity.
View in article
Holler, P.R. (1992) Consolidation Characteristics and Permeabilities of Sediments from the Japan Sea (Sites 798 and 799) In: Tamaki, K., Suyehiro, K., Allan, J., McWilliams, M., et al. (Eds.) Proceedings of the Ocean Drilling Program. Scientific Results 127/128 (Pt. 2). Ocean Drilling Program, College Station, Texas, 1123–1133. https://doi.org/10.2973/odp.proc.sr.127128-2.232.1992

The exceedingly low permeability of biosiliceous deposits (5.35 × 10–20 to 1.97 × 10–14 m2; Holler, 1992) contributes to their greatly lowered sediment diffusivity with depth, especially in the TZA/CT and deeper deposits at Site 794 (Fig. 3).
View in article
Ibarra, D.E., Yanchilina, A.G., Lloyd, M.K., Methner, K.A., Chamberlain, C.P., Yam, R., Shemesh, A., Stolper, D.A. (2022) Triple oxygen isotope systematics of diagenetic recrystallization of diatom opal-A to opal-CT to microquartz in deep sea sediments. Geochimica et Cosmochimica Acta 320, 304–323. https://doi.org/10.1016/j.gca.2021.11.027

Following the original works on oxygen isotope relations among opaline polymorphs involved in silica diagenesis (Knauth and Epstein, 1976; Murata et al., 1977; Pisciotto et al., 1992), Yanchinila et al. (2020, 2021) and Ibarra et al. (2022) provided constraints on temperatures of biosilica transformation into opal-CT in deep water cherts using high resolution characterisation of oxygen-18 isotopic compositions.
View in article
International Ocean Discovery Program (IODP) (2014) Retrieved from http://web.iodp.tamu.edu/

The petrophysical profiles were modified from IODP (2014), and the biosilica distribution pattern with depth from Varkouhi et al. (2020a, 2020b).
View in article
The volcanic glass depth profile was based upon IODP (2014) smear slide data.
View in article
The inverse DSi gradient indicates its removal, while highly reduced diffusivities occur, at the TZA/CT. The physical property profiles were modified from IODP (2014).
View in article
Kita, I., Taguchi, S., Matsubaya, O. (1985) Oxygen isotope fractionation between amorphous silica and water at 34°-93°C. Nature 314, 83–84. https://doi.org/10.1038/314083a0

The δ18O values used to assess the isotopic temperature of opal-CT (see Table S-2) were computed by following Kita et al. (1985).
View in article
Clustering of isotopic temperatures for the TZA/CT sediment section and its underlying unit, extracted from the opal-CT δ18O using the fractionation relation of Kita et al. (1985), near the present temperatures (Fig. 3, Table S-2) supports the notion that biosilica diagenesis has thermodynamically equilibrated with the modern thermal gradient at Site 794 (Pisciotto et al., 1992).
View in article
Knauth, L.P., Epstein, S. (1976) Hydrogen and oxygen isotope ratios in nodular and bedded cherts. Geochimica et Cosmochimica Acta 40, 1095–1108. https://doi.org/10.1016/0016-7037(76)90051-X

Following the original works on oxygen isotope relations among opaline polymorphs involved in silica diagenesis (Knauth and Epstein, 1976; Murata et al., 1977; Pisciotto et al., 1992), Yanchinila et al. (2020, 2021) and Ibarra et al. (2022) provided constraints on temperatures of biosilica transformation into opal-CT in deep water cherts using high resolution characterisation of oxygen-18 isotopic compositions.
View in article
Krissansen-Totton, J., Catling, D.C. (2020) A coupled carbon-silicon cycle model over Earth history: Reverse weathering as a possible explanation of a warm mid-Proterozoic climate. Earth and Planetary Science Letters 537, 116181. https://doi.org/10.1016/j.epsl.2020.116181

This can be attributed to authigenesis of aluminosilicates, i.e. reverse weathering (Krissansen-Totton and Catling, 2020), which is associated with opal-CT production in deep marine deposits.
View in article
Kwon, O., Mobley, K., Carlson, R.L. (2004) Measured permeabilities of diatomaceous sediments and pelagic clay from the northwest Pacific, ODP Site 1179. In: Sager, W.W., Kanazawa, T., Escutia, C., (Eds.) Proceedings of the Ocean Drilling Program. Scientific Results 191. Ocean Drilling Program, College Station, Texas, 1–16. https://dx.doi.org/10.2973/odp.proc.sr.191.005.2004

The low permeable overburden sealing the TZA/CT may impede the interstitial fluid expulsion because advection depends on higher permeability rather than diffusive fluxes (Kwon et al., 2004).
View in article
Marsaglia, K.M., Milliken, K., Doran, L. (2013) Smear Slides of Marine Mud for IODP Core Description: Part I Methodology and Atlas of Siliciclastic and Volcanogenic Components. International Ocean Discovery Program Technical Note 1, 263p. https://doi.org/10.2204/iodp.tn.1.2013

The relative extent of opal-A assessed using visual estimation scheme of Marsaglia et al. (2013) records a peak of 72 % at ∼100 mbsf for a diatom ooze, mixed with fine volcanic glass, sealing the TZA/CT reflector of petrophysical changes (Figs. 1a–e, 2a and S-3a).
View in article
The solubility control is thus reached with opal-CT and yet this polymorph precipitates across the TZA/CT, where it has been largely produced since the late Miocene onset of the transition advancement (Varkouhi et al., 2021).
View in article
Murata, K.J., Friedman, I., Gleason, J.D. (1977) Oxygen isotope relations between diagenetic silica minerals in Monterey Shale, Temblor Range, California. American Journal of Science 277, 259–272. https://doi.org/10.2475/ajs.277.3.259

Following the original works on oxygen isotope relations among opaline polymorphs involved in silica diagenesis (Knauth and Epstein, 1976; Murata et al., 1977; Pisciotto et al., 1992), Yanchinila et al. (2020, 2021) and Ibarra et al. (2022) provided constraints on temperatures of biosilica transformation into opal-CT in deep water cherts using high resolution characterisation of oxygen-18 isotopic compositions.
View in article
Neagu, R.C., Cartwright, J., Davies, R.J., Jensen, L. (2010) Fossilisation of a silica diagenesis reaction front on the mid-Norwegian margin. Marine and Petroleum Geology 27, 2141–2155. https://dx.doi.org/10.1016/j.marpetgeo.2010.09.003

The alteration of opal-A to diagenetic opal (opal-CT) ceases in fossilised transitions, signifying that the TZA/CT is not at the phase predominance boundary between these silica polymorphs (Neagu et al., 2010).
View in article
Elucidating the anomalous compaction drivers is crucial to understanding structural/thermal evolution in basin fills, and plays a role in petroleum systems as the TZA/CT contributes to drilling risks by often comprising hard cherts (Neagu et al., 2010).
View in article
Pisciotto, K.A., Murray, R.W., Brumsack, H.J. (1992) Thermal History of Japan Sea Sediments from Isotopic Studies of Diagenetic Silica and Associated Pore Waters. In: Pisciotto, K.A., Ingle, J.C., Jr., von Breymann, M.T., Barron, J., et al. (Eds.) Proceedings of the Ocean Drilling Program. Scientific Results 127/128 (Pt. 1). Ocean Drilling Program, College Station, Texas, 49–56. https://doi.org/10.2973/odp.proc.sr.127128-1.113.1992

Following the original works on oxygen isotope relations among opaline polymorphs involved in silica diagenesis (Knauth and Epstein, 1976; Murata et al., 1977; Pisciotto et al., 1992), Yanchinila et al. (2020, 2021) and Ibarra et al. (2022) provided constraints on temperatures of biosilica transformation into opal-CT in deep water cherts using high resolution characterisation of oxygen-18 isotopic compositions.
View in article
Clustering of isotopic temperatures for the TZA/CT sediment section and its underlying unit, extracted from the opal-CT δ18O using the fractionation relation of Kita et al. (1985), near the present temperatures (Fig. 3, Table S-2) supports the notion that biosilica diagenesis has thermodynamically equilibrated with the modern thermal gradient at Site 794 (Pisciotto et al., 1992).
View in article
Shipboard Scientific Party (1987) Site 642: Norwegian Sea. In: Eldholm, O., Thiede, J., Taylor, E., et al. (Eds.) Proceedings of the Ocean Drilling Program. Initial Reports 104. Ocean Drilling Program, College Station, Texas, 53–453. https://doi.org/10.2973/odp.proc.ir.104.104.1987

For instance, biosilica diagenesis has led to 16 % pore volume reduction in the early Miocene sediment of Norwegian Sea (Shipboard Scientific Party, 1987) and 15 % in the Neogene claystones of New Jersey Atlantic continental slope (Shipboard Scientific Party, 1994).
View in article
Shipboard Scientific Party (1990) Site 794. In: Tamaki, K., Pisciotto, K., Allan, J., et al. (Eds.) Proceedings of the Ocean Drilling Program. Initial Reports 127. Ocean Drilling Program, College Station, Texas, 71–167. https://doi.org/10.2973/odp.proc.ir.127.104.1990

Under slow hemipelagic sedimentation (1–104 m/Myr; Varkouhi et al., 2021), advection and diffusion potentially erase signatures of non-steady state DSi concentration drops unless diagenesis still continues or occurred recently (the 10–50 m/Myr accumulation rate in Site 794 falls within slow rate ranges; Shipboard Scientific Party, 1990).
View in article
Shipboard Scientific Party (1994) Site 902. In: Mountain, G.S., Miller, K.G., Blum, P., et al. (Eds.) Proceedings of the Ocean Drilling Program. Initial Reports 150. Ocean Drilling Program, College Station, Texas, 63–127. https://doi.org/10.2973/odp.proc.ir.150.106.1994

For instance, biosilica diagenesis has led to 16 % pore volume reduction in the early Miocene sediment of Norwegian Sea (Shipboard Scientific Party, 1987) and 15 % in the Neogene claystones of New Jersey Atlantic continental slope (Shipboard Scientific Party, 1994).
View in article
Varkouhi, S., Cartwright, J.A., Tosca, N.J. (2020a) Anomalous compaction due to silica diagenesis: textural and mineralogical evidence from hemipelagic deep-sea sediments of the Japan Sea. Marine Geology 426, 106204. https://doi.org/10.1016/j.margeo.2020.106204

Nevertheless, siliceous hemipelagic sediments penetrated during the Deep Sea Drilling Project (DSDP) and Ocean Drilling Program (ODP) have undergone pronounced physical property variations (anomalous compaction) that typically accompany biogenic silica (opal-A) alteration toward opal-CT (α-cristobalite/α-tridymite; Varkouhi et al., 2020a).
View in article
This profound reflection marks the position of a transition zone, across which biosilica appreciably dissolves and is replaced with opal-CT (TZA/CT; Varkouhi et al., 2020a).
View in article
The petrophysical profiles were modified from IODP (2014), and the biosilica distribution pattern with depth from Varkouhi et al. (2020a, 2020b).
View in article
Varkouhi, S., Tosca, N.J., Cartwright, J.A. (2020b) Pore water chemistry — A proxy for tracking the signature of ongoing silica diagenesis. Journal of Sedimentary Research 90, 1037–1067. https://doi.org/10.2110/jsr.2020.56

Integration of these procedures with interstitial water chemistry has been applied to the current thermochemical state of biosilica diagenesis across the anomalous compaction section, i.e. active versus fossilised TZA/CT (Varkouhi et al., 2020b).
View in article
Despite research on the TZA/CT structure and thermodynamics (e.g., Davies and Cartwright, 2002; Varkouhi et al., 2020b), the silica dissolution/precipitation kinetics driving petrophysical contrasts between porous opal-A deposits and more condensed opal-CT units is poorly understood.
View in article
While Varkouhi et al. (2020b) describe diagenetic states of the TZA/CT using pore fluid chemical proxies, controls from pore water thermodynamics and kinetic modes of ion transport on petrophysics during silica diagenesis are inconclusive in their work.
View in article
Interstitial water chemical constraints, including saturation states with silica polymorphs and dissolved silicon (DSi) and major ions concentration gradients indicate that opal-A transformation is active over the TZA/CT in this site and other borehole sites drilling the same transition zone (Varkouhi et al., 2020b).
View in article
The petrophysical profiles were modified from IODP (2014), and the biosilica distribution pattern with depth from Varkouhi et al. (2020a, 2020b).
View in article
The saturation profiles were modified from Varkouhi et al. (2020b).
View in article
Notable DSi and lithium releases to the interstitial water above, and removal from the pore solution in the TZA/CT, imply that this transition behaves as a diffuse boundary that develops concentration gradients downwards below it and upwards into the ocean water (Varkouhi et al., 2020b; Figs. 2a, S-2). Because the sediment diffusivity changes with burial (Fig. 3), the profiles linking ionic concentrations above and below the TZA/CT are non-linear.
View in article
The low volcaniclastic content of the biosilica sequence and its negligible distribution in the TZA/CT however, display notably less DSi release to the pore solution through volcanic glass alteration than biosilica dissolution (Fig. 2a; Varkouhi et al., 2020b).
View in article
Varkouhi, S., Tosca, N.J., Cartwright, J.A. (2021) Temperature-time relationships and their implications for thermal history and modelling of silica diagenesis in deep-sea sediments. Marine Geology 439, 106541. https://dx.doi.org/10.1016/j.margeo.2021.106541

The narrow (≤50 m thick) TZA/CT hosts both opal-A and opal-CT, whilst the latter phase dominates (Varkouhi et al., 2021).
View in article
Under slow hemipelagic sedimentation (1–104 m/Myr; Varkouhi et al., 2021), advection and diffusion potentially erase signatures of non-steady state DSi concentration drops unless diagenesis still continues or occurred recently (the 10–50 m/Myr accumulation rate in Site 794 falls within slow rate ranges; Shipboard Scientific Party, 1990).
View in article
Varkouhi, S., Cartwright, J.A., Tosca, N.J., Papineau, D. (2022) Arrested versus active silica diagenesis reaction boundaries—a review of seismic diagnostic criteria. Basin Research 34, 640–661. https://doi.org/10.1111/bre.12634

Investigations into silica diagenesis and TZA/CT characterisation in sub-seabed sediments have been built upon 2D and 3D seismic stratigraphy, scientific drillings, and on-land surveys (Varkouhi et al., 2022).
View in article
Biosilica diagenesis progresses through an active TZA/CT instead. The view of an active silica diagenesis reaction front is based on the assumption that the TZA/CT has a regional sea bottom simulating geometry, which cross cuts the nearby stratigraphy, and is likely to be presently advancing upwards (Varkouhi et al., 2022).
View in article
Volpi, V., Camerlenghi, A., Hillenbrand, C.-D., Rebesco, M., Ivaldi, R. (2003) Effects of biogenic silica on sediment compaction and slope stability on the Pacific margin of the Antarctic Peninsula. Basin Research 15, 339–363. https://doi.org/10.1046/j.1365-2117.2003.00210.x

Distinct sediment density increases occur as porosity declines because pore volume decreases compress the solid matrix by densifying its constituents (Volpi et al., 2003).
View in article
von Breymann, M.T., Brumsack, H., Emeis, K.C. (1992) Depositional and diagenetic behaviour of barium in the Japan Sea. In: Pisciotto, K.A., Ingle, J.C., Jr., von Breymann, M.T., Barron, J., et al. (Eds.) Proceedings of the Ocean Drilling Program. Scientific Results 127/128 (Pt. 1). Ocean Drilling Program, College Station, Texas, 651–665. https://doi.org/10.2973/odp.proc.sr.127128-1.168.1992

Despite low sedimentation rates in Site 794, elevated primary productivity has been able to sustain a continuous opal-A rain to the seabed, and hence is proportional to biosilica burial and DSi recycling (von Breymann et al., 1992).
View in article
Williams, L.A., Parks, G.A., Crerar, D.A. (1985) Silica diagenesis, I. Solubility controls. Journal of Sedimentary Petrology 55, 301–311.

These petrophysical shifts occur through an early burial dissolution-redeposition process whose development is primarily regulated by temperature and time, with lesser controls from siliceous surface area, host lithofacies, and interstitial water chemistry (Williams et al., 1985).
View in article
Wrona, T., Jackson, C.A.-L., Huuse, M., Taylor, K.G. (2017a) Silica diagenesis in Cenozoic mudstones of the North Viking Graben: physical properties and basin modelling. Basin Research 29, 556–575. https://doi.org/10.1111/bre.12168

Conventional perspectives on the compaction of deep water deposits deal with petrophysical properties (e.g., porosity, density, and compressional velocity) as gently evolving traits with depth (Wrona et al., 2017a).
View in article
Wrona, T., Taylor, K.G., Jackson, C.A.-L., Huuse, M., Najorka, J., Pan, I. (2017b) Impact of silica diagenesis on the porosity of fine-grained strata: an analysis of Cenozoic mudstones from the North Sea. Geochemistry, Geophysics, Geosystems 18, 1537–1549. https://doi.org/10.1002/2016GC006482

Prominent opal-A content reductions ascribed to its dissolution, and the subsequent opal-CT precipitation compact the sediment framework and impose a porosity drop on the host lithology (Wrona et al., 2017b).
View in article
The anomalous compressional changes when opal-A undergoes appreciable dissolution happen abruptly (Wrona et al., 2017b).
View in article
Although opal-CT cements in the sediment fabric affect the porosity (Fig. S-4f–h), their impact on pore volume decrease is subordinate to precursor opal-A dissolution (Wrona et al., 2017b).
View in article
Yanchilina, A.G., Yam, R., Kolodny, Y., Shemesh, A. (2020) From diatom opal-A δ18O to chert δ18O in deep sea sediments. Geochimica et Cosmochimica Acta 268, 368–382. https://doi.org/10.1016/j.gca.2019.10.018

Following the original works on oxygen isotope relations among opaline polymorphs involved in silica diagenesis (Knauth and Epstein, 1976; Murata et al., 1977; Pisciotto et al., 1992), Yanchinila et al. (2020, 2021) and Ibarra et al. (2022) provided constraints on temperatures of biosilica transformation into opal-CT in deep water cherts using high resolution characterisation of oxygen-18 isotopic compositions.
View in article
Yanchilina, A.G., Yam, R., Shemesh, A. (2021) The effect of sediment lithology on oxygen isotope composition and phase transformation of marine biogenic opal. Chemical Geology 570, 120175. https://doi.org/10.1016/j.chemgeo.2021.120175

Following the original works on oxygen isotope relations among opaline polymorphs involved in silica diagenesis (Knauth and Epstein, 1976; Murata et al., 1977; Pisciotto et al., 1992), Yanchinila et al. (2020, 2021) and Ibarra et al. (2022) provided constraints on temperatures of biosilica transformation into opal-CT in deep water cherts using high resolution characterisation of oxygen-18 isotopic compositions.
View in article
Yong, Y., Lou, X., Li, S., Yang, C., Yin, X. (2014) Direct simulation of the influence of the pore structure on the diffusion process in porous media. Computers and Mathematics with Applications 67, 412–423, https://dx.doi.org/10.1016/j.camwa.2013.08.032

The burial depth reliance of diffusivity is expressed by its connection to pore structures (Yong et al., 2014), indicating the more reduced diffusion in lower porosity opal-CT sediments than in biosiliceous deposits (Fig. 3).
View in article
top
Supplementary Information
The Supplementary Information includes:
- Study Site — Geological Setting and Stratigraphy
- Sample Preparation and Analytical Methods
- Tables S-1 to S-2
- Figures S-1 to S-4
- Supplementary Information References
Download the Supplementary Information (PDF)