The extent of liquid immiscibility in planetesimal cores
Affiliations | Corresponding Author | Cite as | Funding information- Share this article
-
Article views:7Cumulative count of HTML views and PDF downloads.
- Download Citation
- Rights & Permissions
top
Abstract
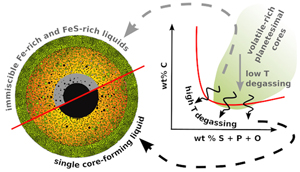
Figures
![]() Figure 1 (a) Liquid compositions in the system Fe0.9Ni0.1, S, P, C, O at 0.5 (red)/1.0 GPa (black), and calculated bulk compositions for immiscible liquids. Tie-lines connect compositions of coexisting immiscible liquids. Insert: examples of run products (see Fig. S-1 for details). (b) Comparison with C-saturated/C-rich experiments, Green: Dasgupta et al. (2009) Fe, S, C system at 2 GPa, Light blue: Corgne et al. (2008) model chondritic system, and their miscibility curve at 4 GPa. Blue line: miscibility curve at 1 bar/1400 °C in the system Fe-S-C calculated using thermodynamic model of Tafwidli and Kang (2017); insert additionally shows fields of C-saturation. Dashed black line: pressure-independent C solubility in Fe/Ni-S liquid at 2–7 GPa (Zhang et al., 2018). | ![]() Figure 2 Model planetesimal core compositions; data from Figure 1b shown in light grey for clarity. (a) Blue circles: magmatic iron meteorite ‘parent core’ compositions from Hilton et al. (2022) with C contents from Grewal and Asimow (2023). (b) Red circles: modelled planetesimal core compositions based on metal/silicate differentiation of averaged chondrite compositions, after Bercovici et al. (2022). Error bars are propagated from uncertainties in S and P contents. Open circles: corresponding ‘minimum’ C core compositions for each chondrite group based on variability in chondrite C contents for petrological types 3–6 (Fig. 3). (c) Blue arrows: modelled liquid compositions with progressive crystallisation of solid Fe/Ni. | ![]() Figure 3 Compositions of carbonaceous and ordinary chondrites as a function of petrological type (left), and for specific groups (right), after Hirschmann et al. (2021). Within each group, colour symbols denote petrological type. Small, black symbols are all other ordinary and carbonaceous chondrite data, for comparison. Red dashed line is a 1:1 fit to data (types 3–6). Compositions from the Metbase (2024) database for samples with combined bulk C and S, and clear petrologic type (http://www.metbase.org, GeoPlatform UG, Germany). |
Figure 1 | Figure 2 | Figure 3 |
top
Introduction
Separation of Fe-rich, core-forming liquids from silicate was a defining process in planetary formation in the inner solar system, and left a geochemical signature on the cores and mantles of rocky planets (Wood, 2008
Wood, B.J. (2008) Accretion and core formation: constraints from metal-silicate partitioning. Philosophical Transactions of the Royal Society a-Mathematical Physical and Engineering Sciences 366, 4339–4355. https://doi.org/10.1098/rsta.2008.0115
). Differentiated asteroids, such as 4-Vesta, and the existence of iron meteorites, imply that core formation also occurred in a proportion of smaller bodies, possibly ranging down to a few 100 km in diameter (McCoy et al., 2006McCoy, T.J., Mittlefehldt, D.W., Wilson, L. (2006) Asteroid Differentiation. In: Dante, S.L., McSween, H.Y. (Eds.) Meteorites and the Early Solar System II. University of Arizona Press, 733-746. https://doi.org/10.2307/j.ctv1v7zdmm.40
; Goldstein et al., 2009Goldstein, J.I., Scott, E.R.D., Chabot, N.L. (2009) Iron meteorites: Crystallization, thermal history, parent bodies, and origin. Geochemistry 69, 293–325. https://doi.org/10.1016/j.chemer.2009.01.002
). Intriguingly, isotopic data from iron meteorites imply formation ages within a few Myr of the onset of accretion in the solar system (Kleine et al., 2009Kleine, T., Touboul, M., Bourdon, B., Nimmo, F., Mezger, K., Palme, H., Jacobsen, S.B., Yin, Q.Z., Halliday, A.N. (2009) Hf-W chronology of the accretion and early evolution of asteroids and terrestrial planets. Geochimica et Cosmochimica Acta 73, 5150–5188. https://doi.org/10.1016/j.gca.2008.11.047
). As such, building blocks of planets such as Earth likely consisted of a proportion of differentiated planetesimals, i.e. small rocky bodies with compositions modified by high temperature processes.In addition to Fe and Ni, planetary cores contain variable concentrations of light elements: S, P, O, C, Si and H (Wood, 2008
Wood, B.J. (2008) Accretion and core formation: constraints from metal-silicate partitioning. Philosophical Transactions of the Royal Society a-Mathematical Physical and Engineering Sciences 366, 4339–4355. https://doi.org/10.1098/rsta.2008.0115
; Goldstein et al., 2009Goldstein, J.I., Scott, E.R.D., Chabot, N.L. (2009) Iron meteorites: Crystallization, thermal history, parent bodies, and origin. Geochemistry 69, 293–325. https://doi.org/10.1016/j.chemer.2009.01.002
). Towards lower pressures, mixing in Fe-rich liquids becomes increasingly non-ideal, resulting in 2-liquid fields in which immiscible O-poor/O-rich or S-poor/S-rich liquids coexist (Urakawa et al., 1987Urakawa, S., Kato, M., Kumazawa, M. (1987) Experimental Study on the Phase Relations in the System Fe-Ni-O-S Up to 15 GPa. In: Manghnani, M.H. and Syono, Y. (Eds.) High‐Pressure Research in Mineral Physics: A Volume in Honor of Syun‐iti Akimoto. American Geophysical Union (AGU), 95–111. https://doi.org/10.1029/GM039p0095
; Chabot and Drake, 2000Chabot, N.L., Drake, M.J. (2000) Crystallization of magmatic iron meteorites: The effects of phosphorus and liquid immiscibility. Meteoritics and Planetary Science 35, 807–816. https://doi.org/10.1111/j.1945-5100.2000.tb01464.x
; Corgne et al., 2008Corgne, A., Wood, B.J., Fei, Y. (2008) C- and S-rich molten alloy immiscibility and core formation of planetesimals. Geochimica et Cosmochimica Acta 72, 2409–2416. https://doi.org/10.1016/j.gca.2008.03.001
; Dasgupta et al., 2009Dasgupta, R., Buono, A., Whelan, G., Walker, D. (2009) High-pressure melting relations in Fe-C-S systems: Implications for formation, evolution, and structure of metallic cores in planetary bodies. Geochimica et Cosmochimica Acta 73, 6678–6691. https://doi.org/10.1016/j.gca.2009.08.001
). Liquid immiscibility affects the composition of the cores of rocky bodies up to the size of Earth’s Moon, would result in core stratification (Dasgupta et al., 2009Dasgupta, R., Buono, A., Whelan, G., Walker, D. (2009) High-pressure melting relations in Fe-C-S systems: Implications for formation, evolution, and structure of metallic cores in planetary bodies. Geochimica et Cosmochimica Acta 73, 6678–6691. https://doi.org/10.1016/j.gca.2009.08.001
), and might influence core segregation processes (Bromiley, 2023Bromiley, G.D. (2023) The Geochemical Legacy of Low-Temperature, Percolation-Driven Core Formation in Planetesimals. Earth, Moon, and Planets 127, 4. https://doi.org/10.1007/s11038-023-09552-2
). However, constraints on the extent of core immiscibility are limited, with most previous experimental work confined to simplified systems and/or conducted at pressures less relevant to planetesimal interiors (>3 GPa). In addition, although C has been shown to have a key role in reducing miscibility in Fe-rich liquids (Corgne et al., 2008Corgne, A., Wood, B.J., Fei, Y. (2008) C- and S-rich molten alloy immiscibility and core formation of planetesimals. Geochimica et Cosmochimica Acta 72, 2409–2416. https://doi.org/10.1016/j.gca.2008.03.001
; Dasgupta et al., 2009Dasgupta, R., Buono, A., Whelan, G., Walker, D. (2009) High-pressure melting relations in Fe-C-S systems: Implications for formation, evolution, and structure of metallic cores in planetary bodies. Geochimica et Cosmochimica Acta 73, 6678–6691. https://doi.org/10.1016/j.gca.2009.08.001
), these studies were conducted in C-saturated or C-rich systems, i.e. in systems not directly comparable to volatile-depleted planetesimals (Hirschmann et al., 2021Hirschmann, M.M., Bergin, E.A., Blake, G.A., Ciesla, F.J., Li, J. (2021) Early volatile depletion on planetesimals inferred from C-S systematics of iron meteorite parent bodies. Proceedings of the National Academy of Sciences 118, e2026779118. https://doi.org/10.1073/pnas.2026779118
).Here, we describe superliquidus experiments which constrain liquid immiscibility in the system Fe, Ni, S, P, C, O from 0.5 to 1 GPa, at 1673 K, i.e. under conditions relevant to differentiation in planetesimals a few 100 km in diameter (Grewal and Asimow, 2023
Grewal, D.S., Asimow, P.D. (2023) Origin of the superchondritic carbon/nitrogen ratio of the bulk silicate Earth – an outlook from iron meteorites. Geochimica et Cosmochimica Acta 344, 146–159. https://doi.org/10.1016/j.gca.2023.01.012
). Using constraints on the compositions of planetesimal cores from meteorite data, we then assess the importance of immiscibility in planetesimal cores.top
Liquid Immiscibility in the System Fe, Ni, S, P, C, O
Starting mixes were prepared from high purity reagents, with variable S, P and C, based on a fixed Fe0.9Ni0.1 ratio, broadly representative of a range of planetesimal cores (Goldstein et al., 2009
Goldstein, J.I., Scott, E.R.D., Chabot, N.L. (2009) Iron meteorites: Crystallization, thermal history, parent bodies, and origin. Geochemistry 69, 293–325. https://doi.org/10.1016/j.chemer.2009.01.002
). Incorporation of minor O into run products is expected depending on oxygen fugacity, fO2, within experiments (Corgne et al., 2008Corgne, A., Wood, B.J., Fei, Y. (2008) C- and S-rich molten alloy immiscibility and core formation of planetesimals. Geochimica et Cosmochimica Acta 72, 2409–2416. https://doi.org/10.1016/j.gca.2008.03.001
; Dasgupta et al., 2009Dasgupta, R., Buono, A., Whelan, G., Walker, D. (2009) High-pressure melting relations in Fe-C-S systems: Implications for formation, evolution, and structure of metallic cores in planetary bodies. Geochimica et Cosmochimica Acta 73, 6678–6691. https://doi.org/10.1016/j.gca.2009.08.001
). Starting mixes were loaded into graphite (C-saturated), MgO or BN capsules (C-undersaturated), and inserted into piston cylinder assemblies. Experiments at 0.5 GPa exhibited significant P and to a lesser degree S loss. A second series of experiments conducted at 1 GPa exhibited only minor volatile loss, but with liquid compositions consistent with 0.5 GPa experiments (Fig. 1a, Fig. S-2a, Tables S-1 and S-2). For 1.0 GPa experiments, rapid capsule recrystallisation during annealing and heating inhibited volatile loss. For 0.5 GPa experiments, it is likely that greater volatility resulted in volatile loss during initial stages of experiments. Capsule recrystallisation at 1673 K likely inhibited further volatile loss, followed by re-equilibration across sample volumes (see Supplementary Information for discussion). Rapid equilibration in Fe-rich liquids suggests that chemical equilibration would have been attained in experiments with several minutes, even with early volatile loss.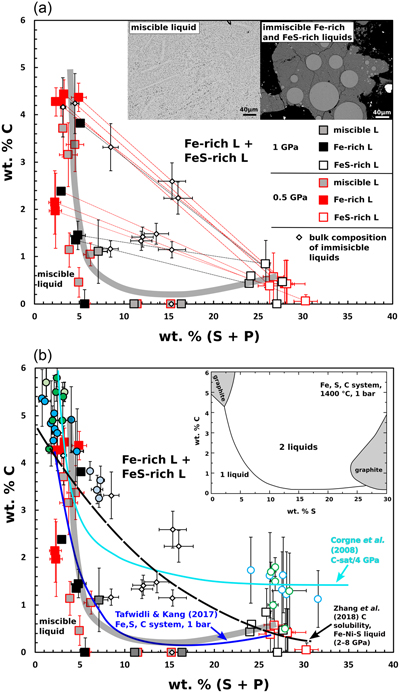
Figure 1 (a) Liquid compositions in the system Fe0.9Ni0.1, S, P, C, O at 0.5 (red)/1.0 GPa (black), and calculated bulk compositions for immiscible liquids. Tie-lines connect compositions of coexisting immiscible liquids. Insert: examples of run products (see Fig. S-1 for details). (b) Comparison with C-saturated/C-rich experiments, Green: Dasgupta et al. (2009)
Dasgupta, R., Buono, A., Whelan, G., Walker, D. (2009) High-pressure melting relations in Fe-C-S systems: Implications for formation, evolution, and structure of metallic cores in planetary bodies. Geochimica et Cosmochimica Acta 73, 6678–6691. https://doi.org/10.1016/j.gca.2009.08.001
Fe, S, C system at 2 GPa, Light blue: Corgne et al. (2008)Corgne, A., Wood, B.J., Fei, Y. (2008) C- and S-rich molten alloy immiscibility and core formation of planetesimals. Geochimica et Cosmochimica Acta 72, 2409–2416. https://doi.org/10.1016/j.gca.2008.03.001
model chondritic system, and their miscibility curve at 4 GPa. Blue line: miscibility curve at 1 bar/1400 °C in the system Fe-S-C calculated using thermodynamic model of Tafwidli and Kang (2017)Tafwidli, F., Kang, Y. (2017) Thermodynamic Modeling of Fe–C–S Ternary System. Isij International 57, 782–790. https://doi.org/10.2355/isijinternational.ISIJINT-2016-672
; insert additionally shows fields of C-saturation. Dashed black line: pressure-independent C solubility in Fe/Ni-S liquid at 2–7 GPa (Zhang et al., 2018Zhang, Z., Hastings, P., Von der Handt, A., Hirschmann, M.M. (2018) Experimental determination of carbon solubility in Fe-Ni-S melts. Geochimica et Cosmochimica Acta 225, 66–79. https://doi.org/10.1016/j.gca.2018.01.009
).As expected, light element content controls liquid stability in our experiments, driving immiscibility and separation of what we term Fe-rich (low S, high P, high C, lower O) and FeS-rich (high S, low P, low C, higher O) liquids. Figure 1b shows comparison of our results with higher pressure C-saturated experiments (Corgne et al., 2008
Corgne, A., Wood, B.J., Fei, Y. (2008) C- and S-rich molten alloy immiscibility and core formation of planetesimals. Geochimica et Cosmochimica Acta 72, 2409–2416. https://doi.org/10.1016/j.gca.2008.03.001
; Dasgupta et al., 2009Dasgupta, R., Buono, A., Whelan, G., Walker, D. (2009) High-pressure melting relations in Fe-C-S systems: Implications for formation, evolution, and structure of metallic cores in planetary bodies. Geochimica et Cosmochimica Acta 73, 6678–6691. https://doi.org/10.1016/j.gca.2009.08.001
) and the calculated 1 bar Fe-S-C phase diagram (Tafwidli and Kang, 2017Tafwidli, F., Kang, Y. (2017) Thermodynamic Modeling of Fe–C–S Ternary System. Isij International 57, 782–790. https://doi.org/10.2355/isijinternational.ISIJINT-2016-672
). Under conditions of planetesimal interiors (i.e. <1 GPa), miscibility in core-forming liquids is restricted to volatile-poor compositions. C is particular important in defining the extent of immiscibility in core-forming liquids; e.g., <1 wt. % C results in immiscibility in otherwise miscible liquids at S contents >5 wt. % S. This mirrors the compositional dependence of C solubility in Fe/Ni/S liquids, which is markedly lower at higher S contents (Zhang et al., 2018Zhang, Z., Hastings, P., Von der Handt, A., Hirschmann, M.M. (2018) Experimental determination of carbon solubility in Fe-Ni-S melts. Geochimica et Cosmochimica Acta 225, 66–79. https://doi.org/10.1016/j.gca.2018.01.009
; Fig. 1b). Conversely, C solubility increases with increasing Ni/Fe ratio, implying that the miscibility field in Figure 1 expands with increasing Ni content. P also reduces miscibility in Fe-S liquids (Chabot and Drake, 2000Chabot, N.L., Drake, M.J. (2000) Crystallization of magmatic iron meteorites: The effects of phosphorus and liquid immiscibility. Meteoritics and Planetary Science 35, 807–816. https://doi.org/10.1111/j.1945-5100.2000.tb01464.x
), although to a lesser extent than C (Fig. S-2b). Our data cannot differentiate the influence of P and O on miscibility. However, coincidence of our Fe0.9Ni0.1 miscibility curve at 0.5/1 GPa with the 1 bar Fe-S-C miscibility curve from Tafwidli and Kang (2017)Tafwidli, F., Kang, Y. (2017) Thermodynamic Modeling of Fe–C–S Ternary System. Isij International 57, 782–790. https://doi.org/10.2355/isijinternational.ISIJINT-2016-672
, suggests that wt. % concentrations of P and O signficantly reduce miscibility. By extension, in planetesimals with interior pressures <0.5 GPa, the miscibility field in core-forming liquids is likely further reduced compared to that shown in Figure 1.top
Discussion
At pressures up to 1 GPa, miscibility in core-forming liquids is limited to Fe-rich liquids with low light element contents and/or high Ni contents. With increasing planetesimal size and internal pressure, immiscibility is restricted to increasingly light element-rich core compositions. The most significant uncertainty in assessing the importance of immiscibility in planetesimals is the light element content of their metallic cores.
Volatile-poor planetesimal cores: iron meteorite parent liquids. Magmatic iron meteorites are believed to have originated as the cores of differentiated, rocky planetesimals (Goldstein et al., 2009
Goldstein, J.I., Scott, E.R.D., Chabot, N.L. (2009) Iron meteorites: Crystallization, thermal history, parent bodies, and origin. Geochemistry 69, 293–325. https://doi.org/10.1016/j.chemer.2009.01.002
), and as they date from a few Myr after the onset of solar system accretion (Kleine et al., 2009Kleine, T., Touboul, M., Bourdon, B., Nimmo, F., Mezger, K., Palme, H., Jacobsen, S.B., Yin, Q.Z., Halliday, A.N. (2009) Hf-W chronology of the accretion and early evolution of asteroids and terrestrial planets. Geochimica et Cosmochimica Acta 73, 5150–5188. https://doi.org/10.1016/j.gca.2008.11.047
; Spitzer et al., 2021Spitzer, F., Burkhardt, C., Nimmo, F., Kleine, T. (2021) Nucleosynthetic Pt isotope anomalies and the Hf-W chronology of core formation in inner and outer solar system planetesimals. Earth and Planetary Science Letters 576, 117211. https://doi.org/10.1016/j.epsl.2021.117211
), provide insight into the early stages of planetary formation (e.g., Chabot and Drake, 2000Chabot, N.L., Drake, M.J. (2000) Crystallization of magmatic iron meteorites: The effects of phosphorus and liquid immiscibility. Meteoritics and Planetary Science 35, 807–816. https://doi.org/10.1111/j.1945-5100.2000.tb01464.x
; Hirschmann et al., 2021Hirschmann, M.M., Bergin, E.A., Blake, G.A., Ciesla, F.J., Li, J. (2021) Early volatile depletion on planetesimals inferred from C-S systematics of iron meteorite parent bodies. Proceedings of the National Academy of Sciences 118, e2026779118. https://doi.org/10.1073/pnas.2026779118
; Grewal and Asimow, 2023Grewal, D.S., Asimow, P.D. (2023) Origin of the superchondritic carbon/nitrogen ratio of the bulk silicate Earth – an outlook from iron meteorites. Geochimica et Cosmochimica Acta 344, 146–159. https://doi.org/10.1016/j.gca.2023.01.012
; Grewal et al., 2024Grewal, D.S., Nie, N.X., Zhang, B., Izidoro, A., Asimow, P.D. (2024) Accretion of the earliest inner Solar System planetesimals beyond the water snowline. Nature Astronomy 8, 290–297. https://doi.org/10.1038/s41550-023-02172-w
). However, as magmatic iron meteorites formed by near-fractional crystallisation, the composition of liquids from which they crystallised must be inferred. There are various models for reconstructing compositions of magmatic iron meteorite parental liquids (IMPLs), although results from these are broadly consistent, at least for elements such as S, which have a strong influence on element partitioning (e.g., Goldstein et al., 2009Goldstein, J.I., Scott, E.R.D., Chabot, N.L. (2009) Iron meteorites: Crystallization, thermal history, parent bodies, and origin. Geochemistry 69, 293–325. https://doi.org/10.1016/j.chemer.2009.01.002
; Hilton et al., 2022Hilton, C.D., Ash, R.D., Walker, R.J. (2022) Chemical characteristics of iron meteorite parent bodies. Geochimica et Cosmochimica Acta 318, 112–125. https://doi.org/10.1016/j.gca.2021.11.035
; Zhang et al., 2024Zhang, B., Chabot, N.L., Rubin, A.E. (2024) Compositions of iron-meteorite parent bodies constrain the structure of the protoplanetary disk. Proceedings of the National Academy of Sciences 121, e2306995121. https://doi.org/10.1073/pnas.2306995121
). As shown in Figures 2a and S-2b, IMPL compositions are light element-poor and, as a consequence, except for IIC (Fig. S-2b), single liquids regardless of planetesimal size. However, the possibility that other IMPLs were low C/moderate S immiscible liquids cannot be entirely dismissed.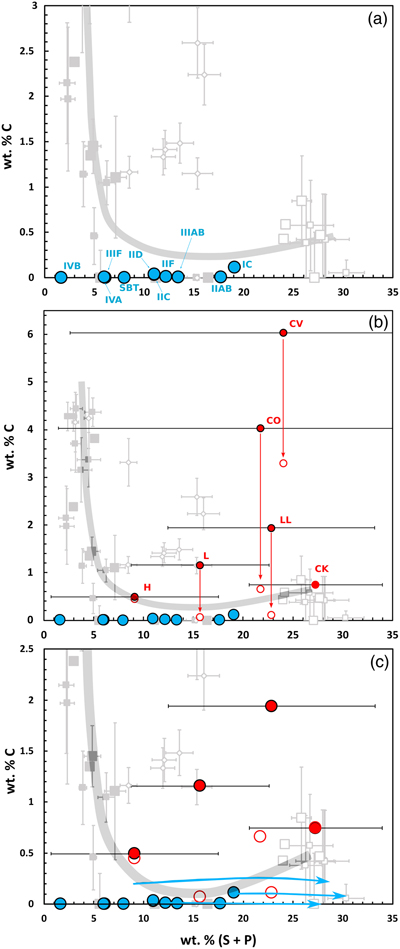
Figure 2 Model planetesimal core compositions; data from Figure 1b shown in light grey for clarity. (a) Blue circles: magmatic iron meteorite ‘parent core’ compositions from Hilton et al. (2022)
Hilton, C.D., Ash, R.D., Walker, R.J. (2022) Chemical characteristics of iron meteorite parent bodies. Geochimica et Cosmochimica Acta 318, 112–125. https://doi.org/10.1016/j.gca.2021.11.035
with C contents from Grewal and Asimow (2023)Grewal, D.S., Asimow, P.D. (2023) Origin of the superchondritic carbon/nitrogen ratio of the bulk silicate Earth – an outlook from iron meteorites. Geochimica et Cosmochimica Acta 344, 146–159. https://doi.org/10.1016/j.gca.2023.01.012
. (b) Red circles: modelled planetesimal core compositions based on metal/silicate differentiation of averaged chondrite compositions, after Bercovici et al. (2022)Bercovici, H.L., Elkins-Tanton, L.T., O’Rourke, J.G., Schaefer, L. (2022) The effects of bulk composition on planetesimal core sulfur content and size. Icarus 380, 114976. https://doi.org/10.1016/j.icarus.2022.114976
. Error bars are propagated from uncertainties in S and P contents. Open circles: corresponding ‘minimum’ C core compositions for each chondrite group based on variability in chondrite C contents for petrological types 3–6 (Fig. 3). (c) Blue arrows: modelled liquid compositions with progressive crystallisation of solid Fe/Ni.IMPLs are parental liquids which solidified to form magmatic iron meteorites. Although they originated as core-forming liquids in planetesimals, the extent to which they evidence the original light element content of planetesimal cores is unclear, as they could have been modified by degassing, i.e. loss of volatile elements, during and after planetesimal disruption. Hirschmann et al. (2021)
Hirschmann, M.M., Bergin, E.A., Blake, G.A., Ciesla, F.J., Li, J. (2021) Early volatile depletion on planetesimals inferred from C-S systematics of iron meteorite parent bodies. Proceedings of the National Academy of Sciences 118, e2026779118. https://doi.org/10.1073/pnas.2026779118
argued that compositions of IMPLs largely reflect the primary volatile content of planetesimal cores. Specifically, they noted that low C/S in IMPLs mirrors trends in log C/S vs. log C in chondrites, which they identify as a signature of metamorphic degassing in rocky planetesimals. They argued that degassing in planetesimals during subsequent melting and differentiation would further reduce C/S to values recorded in IMPL compositions. By contrast, volatile loss from unshielded planetesimal cores, i.e. following planetesimal disruption, would result in preferential S loss and an increase in C/S (Hirschmann et al., 2021Hirschmann, M.M., Bergin, E.A., Blake, G.A., Ciesla, F.J., Li, J. (2021) Early volatile depletion on planetesimals inferred from C-S systematics of iron meteorite parent bodies. Proceedings of the National Academy of Sciences 118, e2026779118. https://doi.org/10.1073/pnas.2026779118
).Re-examination of a larger dataset of chondritic compositions casts doubt on this interpretation (Fig. 3). There are marked trends in log C/S versus log C in both carbonaceous and non-carbonaceous (ordinary) chondrite compositions, but no systematic trends with petrological type, a proxy for peak metamorphic temperature. In contrast, (1) there is greater variability within petrological type than between types for ordinary chondrites, (2) type 3 chondrites, which represent the mineralogically most ‘pristine’ material, span the full range of C and C/S, and (3) variations for types 3–6 across both populations are statistically indistinguishable from a 1:1 relationship, i.e. a 2 order of magnitude variation in C at constant S. Instead, variability of C contents in chondrites is largely independent of variability in S, and, therefore, not reflective of the extent of degassing during metamorphism.
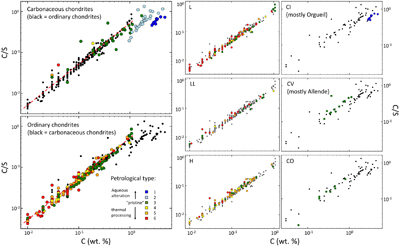
Figure 3 Compositions of carbonaceous and ordinary chondrites as a function of petrological type (left), and for specific groups (right), after Hirschmann et al. (2021)
Hirschmann, M.M., Bergin, E.A., Blake, G.A., Ciesla, F.J., Li, J. (2021) Early volatile depletion on planetesimals inferred from C-S systematics of iron meteorite parent bodies. Proceedings of the National Academy of Sciences 118, e2026779118. https://doi.org/10.1073/pnas.2026779118
. Within each group, colour symbols denote petrological type. Small, black symbols are all other ordinary and carbonaceous chondrite data, for comparison. Red dashed line is a 1:1 fit to data (types 3–6). Compositions from the Metbase (2024) database for samples with combined bulk C and S, and clear petrologic type (http://www.metbase.org, GeoPlatform UG, Germany).Volatile concentrations in chondrites partly mirror variability in moderately volatile element (MVE) contents, which reflect nebular processes such as incomplete condensation and mixing between distinct reservoirs (Braukmüller et al., 2018
Braukmüller, N., Wombacher, F., Hezel, D.C., Escoube, R., Münker, C. (2018) The chemical composition of carbonaceous chondrites: Implications for volatile element depletion, complementarity and alteration. Geochimica et Cosmochimica Acta 239, 17–48. https://doi.org/10.1016/j.gca.2018.07.023
). Subsequent variation in C reflects low temperature rather than high temperature degassing processes. This is supported by step heating experiments (Court and Sephton, 2014Court, R.W., Sephton, M.A. (2014) New estimates of the production of volatile gases from ablating carbonaceous micrometeoroids at Earth and Mars during an E-belt-type Late Heavy Bombardment. Geochimica et Cosmochimica Acta 145, 175–205. https://doi.org/10.1016/j.gca.2014.09.010
) which demonstrate that substantial C loss and minor S loss only occurs at low temperatures. Similarly, correlations between bulk C and N in chondrites and peak metamorphic temperatures have been argued to imply that C loss occurred at low temperatures (<200 °C) (Grewal, 2022Grewal, D.S. (2022) Origin of nitrogen isotopic variations in the rocky bodies of the solar system. The Astrophysical Journal 937, 123. https://doi.org/10.3847/1538-4357/ac8eb4
; Grewal et al. 2022Grewal, D.S., Seales, J.D., Dasgupta, R. (2022) Internal or external magma oceans in the earliest protoplanets – Perspectives from nitrogen and carbon fractionation. Earth and Planetary Science Letters 598, 117847. https://doi.org/10.1016/j.epsl.2022.117847
).Chondrite compositions cannot be used to infer a single high temperature degassing process. By extension, although IMPLs have low C/S compared to chondrites, no simple inferences can be drawn regarding the relative timing of volatile loss. Iron meteorites evidence a family of light element-poor, largely single liquids. However, cooling rates, isotope systematics and MVE contents suggest that, at least, some groups evidence degassing after core segregation and following planetesimal disruption (e.g., Yang et al., 2007
Yang, J., Goldstein, J.I., Scott, E.R.D. (2007) Iron meteorite evidence for early formation and catastrophic disruption of protoplanets. Nature 446, 888–891. https://doi.org/10.1038/nature05735
; Matthes et al., 2018Matthes, M., Fischer-Gödde, M., Kruijer, T.S., Kleine, T. (2018) Pd-Ag chronometry of IVA iron meteorites and the crystallization and cooling of a protoplanetary core. Geochimica et Cosmochimica Acta 220, 82–95. https://doi.org/10.1016/j.gca.2017.09.009
; Hunt et al., 2022Hunt, A.C., Theis, K.J., Rehkämper, M., Benedix, G.K., Andreasen, R., Schönbächler, M. (2022) The dissipation of the solar nebula constrained by impacts and core cooling in planetesimals. Nature Astronomy 6, 812–818. https://doi.org/10.1038/s41550-022-01675-2
; Rubin et al., 2022Rubin, A.E., Zhang, B., Chabot, N.L. (2022) IVA iron meteorites as late-stage crystallization products affected by multiple collisional events. Geochimica et Cosmochimica Acta 331, 1–17. https://doi.org/10.1016/j.gca.2022.05.020
; Zhang et al., 2024Zhang, B., Chabot, N.L., Rubin, A.E. (2024) Compositions of iron-meteorite parent bodies constrain the structure of the protoplanetary disk. Proceedings of the National Academy of Sciences 121, e2306995121. https://doi.org/10.1073/pnas.2306995121
). Absolute volatile contents in IMPL might not, therefore, fully reflect initial planetesimal core compositions.Volatile-rich planetesimal cores: modelling chondrite differentiation. Variability in the C content of chondrites reflects low temperature degassing processes. However, as some ordinary chondrites evidence peak metamorphic temperatures up to the onset of FeS melting close to 1000 °C (Mare et al., 2014
Mare, E.R., Tomkins, A.G., Godel, B.M. (2014) Restriction of parent body heating by metal-troilite melting: Thermal models for the ordinary chondrites. Meteoritics and Planetary Science 49, 636–651. https://doi.org/10.1111/maps.12280
), chondrite compositions are useful in assessing total volatile content in planetesimals prior to/at the onset of core formation. This can be more meaningfully compared to IMPL compositions by calculating hypothetical planetsimal core compositions based on chondrite data. Bercovici et al. (2022)Bercovici, H.L., Elkins-Tanton, L.T., O’Rourke, J.G., Schaefer, L. (2022) The effects of bulk composition on planetesimal core sulfur content and size. Icarus 380, 114976. https://doi.org/10.1016/j.icarus.2022.114976
modelled core/mantle differentiation for 8 averaged chondritic compositions to constrain S content of differentiated planetesimal cores. Here, we use the same approach to calculate S, C and P contents of planetesimal cores based on average chondrite compositions. However, as C contents of chondrites are highly variable and reflect low temperature processes, we additionally calculate core compositions based on minimum C contents in chondrites; i.e. we calculate a lower bound of C for each average chondrite composition based on the full variability of C contents shown in Figure 3 (see Supplementary Information for full details).Calculated core compositions based on averaged chondrite data (filled red circles, Fig. 2b) have S contents of 9–37 %, and P core contents of 0.5–2.5 % (Table S-4). ‘Minimum C’ models give a more meaningful assessment of core C contents, as the large variability in C contents in chondrites could indicate later C-enrichment in parent bodies; minimum C core contents (open circles) range from <0.1 to 3.3 %. Core compositions extend across the miscibility curve in Figure 2b; however, S and P contents of all except those based on average H and L chondrites are high enough to result in immisciblity excluding any influence of C (Fig. S-2b). Large uncertainties in modelled core compositions arise from signficant variations in volatile contents in chondrite compositions. Regardless, in comparison IMPL compositions are volatile-poor (Hilton et al., 2022
Hilton, C.D., Ash, R.D., Walker, R.J. (2022) Chemical characteristics of iron meteorite parent bodies. Geochimica et Cosmochimica Acta 318, 112–125. https://doi.org/10.1016/j.gca.2021.11.035
; Grewal and Asimow, 2023Grewal, D.S., Asimow, P.D. (2023) Origin of the superchondritic carbon/nitrogen ratio of the bulk silicate Earth – an outlook from iron meteorites. Geochimica et Cosmochimica Acta 344, 146–159. https://doi.org/10.1016/j.gca.2023.01.012
), and record signficant C loss, and moderate S and P loss compared to model chondrite core compositions. This is consistent with multiple processes of element depletion, both before and after differentiation and core formation.Our modelled chondrite core compositions provide upper bounds on volatile content of planetesimal cores, as chondrite compositions do not record degassing during planetesimal differentiation. The extent of planetesimal degassing will depend on thermal history and differentiation processes (e.g., Kaminski et al., 2020
Kaminski, E., Limare, A., Kenda, B., Chaussidon, M. (2020) Early accretion of planetesimals unraveled by the thermal evolution of the parent bodies of magmatic iron meteorites. Earth and Planetary Science Letters 548, 116496. https://doi.org/10.1016/j.epsl.2020.116469
). Significant volatile loss would occur on planetesimals which underwent largescale melting, particularly for those with external as opposed to internal magma oceans (Hirschmann et al., 2021Hirschmann, M.M., Bergin, E.A., Blake, G.A., Ciesla, F.J., Li, J. (2021) Early volatile depletion on planetesimals inferred from C-S systematics of iron meteorite parent bodies. Proceedings of the National Academy of Sciences 118, e2026779118. https://doi.org/10.1073/pnas.2026779118
; Grewal et al., 2022Grewal, D.S., Seales, J.D., Dasgupta, R. (2022) Internal or external magma oceans in the earliest protoplanets – Perspectives from nitrogen and carbon fractionation. Earth and Planetary Science Letters 598, 117847. https://doi.org/10.1016/j.epsl.2022.117847
). However, some thermal modelling suggests that silicate melting in planetesimals would be limited in extent and duration (Kaminski et al., 2020Kaminski, E., Limare, A., Kenda, B., Chaussidon, M. (2020) Early accretion of planetesimals unraveled by the thermal evolution of the parent bodies of magmatic iron meteorites. Earth and Planetary Science Letters 548, 116496. https://doi.org/10.1016/j.epsl.2020.116469
; see Supplementary Information), and it is argued that core formation in some planetesimals could proceed without large-scale silicate melting (e.g., Bromiley, 2023Bromiley, G.D. (2023) The Geochemical Legacy of Low-Temperature, Percolation-Driven Core Formation in Planetesimals. Earth, Moon, and Planets 127, 4. https://doi.org/10.1007/s11038-023-09552-2
). As such, the extent of degassing in planetesimals during core formation could have been variable. In turn, the importance of immiscibility in core-forming liquids, which is a function of degassing and planetesimal thermal history, is difficult to constrain. However, based on meteorite data (Figure 2 and S-2), it is clear that evidence for or against immiscibility can provide useful constraints on the extent of degassing prior to and during planetary differentiation, and immiscibility probably played an important role in the evolution of some differentiating bodies. One pertinent illustration of this is segregation processes in icy/rocky bodies in the outer solar system. It has been suggested (e.g., Melwani Daswani et al., 2021Melwani Daswani, M., Vance, S.D., Mayne, M.J., Glein, C.R. (2021) A Metamorphic Origin for Europa’s Ocean. Geophysical Research Letters 48, e2021GL094143. https://doi.org/10.1029/2021GL094143
) that some icy moons have bulk compositions close to those of carbonaceous chondrites, and formed following slow heating and segregation of rock/ice/metal over 10s–100s Myr timescales. Slow differentiation and retention of volatiles in these bodies explain the presence and composition of thick cryospheres. Under these conditions, immiscibility in core-forming liquids may be inevitable for bodies with internal pressures <5–8 GPa.Immiscibility during core solidification. It is often assumed that solidification of planetesimal cores would progressively enrich remaining liquid in light elements, eventually resulting in immiscibility (e.g., Chabot and Drake, 2000
Chabot, N.L., Drake, M.J. (2000) Crystallization of magmatic iron meteorites: The effects of phosphorus and liquid immiscibility. Meteoritics and Planetary Science 35, 807–816. https://doi.org/10.1111/j.1945-5100.2000.tb01464.x
; Corgne et al., 2008Corgne, A., Wood, B.J., Fei, Y. (2008) C- and S-rich molten alloy immiscibility and core formation of planetesimals. Geochimica et Cosmochimica Acta 72, 2409–2416. https://doi.org/10.1016/j.gca.2008.03.001
; Goldstein et al., 2009Goldstein, J.I., Scott, E.R.D., Chabot, N.L. (2009) Iron meteorites: Crystallization, thermal history, parent bodies, and origin. Geochemistry 69, 293–325. https://doi.org/10.1016/j.chemer.2009.01.002
; Bercovici et al., 2022Bercovici, H.L., Elkins-Tanton, L.T., O’Rourke, J.G., Schaefer, L. (2022) The effects of bulk composition on planetesimal core sulfur content and size. Icarus 380, 114976. https://doi.org/10.1016/j.icarus.2022.114976
). Although there is a lack of experimental data on solid/liquid element partitioning in complex systems, compositional controls on light element partitioning can be used to assess changes in liquid composition during core solidification. Here, we use data from Grewal and Asimow (2023)Grewal, D.S., Asimow, P.D. (2023) Origin of the superchondritic carbon/nitrogen ratio of the bulk silicate Earth – an outlook from iron meteorites. Geochimica et Cosmochimica Acta 344, 146–159. https://doi.org/10.1016/j.gca.2023.01.012
and Zhai et al. (2022)Zhai, K., Yin, Y., Zhai, S. (2022) New constraints on the abundances of phosphorus and sulfur in the lunar core: High-pressure and high-temperature experimental study of the FeSP ternary system. Geochimica et Cosmochimica Acta 334, 1–13. https://doi.org/10.1016/j.gca.2022.07.024
to model incremental extraction of small batches of solid Fe, Ni from single liquid planetesimal core-forming liquids (Fig. 2c; see Supplementary Information for details). Blue arrows denote modelled liquid compositions during crystallisation of 3 core-forming liquids. Due to the highly incompatible nature of S (e.g., Dasgupta et al., 2009Dasgupta, R., Buono, A., Whelan, G., Walker, D. (2009) High-pressure melting relations in Fe-C-S systems: Implications for formation, evolution, and structure of metallic cores in planetary bodies. Geochimica et Cosmochimica Acta 73, 6678–6691. https://doi.org/10.1016/j.gca.2009.08.001
), liquids become progressively S-rich with crystallisation. However, as P and C partitioning is strongly dependent on liquid S content, concentrations of both initially increase in liquids during crystallisation, but ultimately decrease at high liquid S contents (Fig. 2c). Consequentially, liquid compositions evolve towards FeS rather than becoming light-element enriched and moving into the 2-liquid field. Progression in liquid compositions is model dependent, and the approach used here assumes no control of temperature or cross-compositional terms for C and P. Regardless, an inevitable consequence of non-ideal mixing of C, P and S is that liquids must progress towards S-rich, C-poor and P-poor compositions during crystallisation. Some single liquid compositions remain in the 1-liquid stability field. Other liquids can progress into the 2-liquid field, and depending on extent of liquid segregation, may then eventually evolve back towards single liquids. As such, immiscibility is not simply ascribable to core solidification, but, again, is largely a function of planetesimal composition, size and differentiation processes.top
Acknowledgements
Authors acknowledge financial support of the Royal Society (International Exchange grant IEC\R3\213008) and Leverhulme Trust (RPG-2021-015). GDB thanks Dr. Nicola Cayzer for advice and assistance in coating samples and performing SEM analysis. This manuscript benefited greatly from thoughtful and constructive reviews by Damanveer Grewal and an anonymous reviewer.
Editor: Rául Fonseca
top
References
Bercovici, H.L., Elkins-Tanton, L.T., O’Rourke, J.G., Schaefer, L. (2022) The effects of bulk composition on planetesimal core sulfur content and size. Icarus 380, 114976. https://doi.org/10.1016/j.icarus.2022.114976

(b) Red circles: modelled planetesimal core compositions based on metal/silicate differentiation of averaged chondrite compositions, after Bercovici et al. (2022).
View in article
Bercovici et al. (2022) modelled core/mantle differentiation for 8 averaged chondritic compositions to constrain S content of differentiated planetesimal cores.
View in article
It is often assumed that solidification of planetesimal cores would progressively enrich remaining liquid in light elements, eventually resulting in immiscibility (e.g., Chabot and Drake, 2000; Corgne et al., 2008; Goldstein et al., 2009; Bercovici et al., 2022).
View in article
Braukmüller, N., Wombacher, F., Hezel, D.C., Escoube, R., Münker, C. (2018) The chemical composition of carbonaceous chondrites: Implications for volatile element depletion, complementarity and alteration. Geochimica et Cosmochimica Acta 239, 17–48. https://doi.org/10.1016/j.gca.2018.07.023

Volatile concentrations in chondrites partly mirror variability in moderately volatile element (MVE) contents, which reflect nebular processes such as incomplete condensation and mixing between distinct reservoirs (Braukmüller et al., 2018).
View in article
Bromiley, G.D. (2023) The Geochemical Legacy of Low-Temperature, Percolation-Driven Core Formation in Planetesimals. Earth, Moon, and Planets 127, 4. https://doi.org/10.1007/s11038-023-09552-2

Liquid immiscibility affects the composition of the cores of rocky bodies up to the size of Earth’s Moon, would result in core stratification (Dasgupta et al., 2009), and might influence core segregation processes (Bromiley, 2023).
View in article
However, some thermal modelling suggests that silicate melting in planetesimals would be limited in extent and duration (Kaminski et al., 2020; see Supplementary Information), and it is argued that core formation in some planetesimals could proceed without large-scale silicate melting (e.g., Bromiley, 2023).
View in article
Chabot, N.L., Drake, M.J. (2000) Crystallization of magmatic iron meteorites: The effects of phosphorus and liquid immiscibility. Meteoritics and Planetary Science 35, 807–816. https://doi.org/10.1111/j.1945-5100.2000.tb01464.x

Towards lower pressures, mixing in Fe-rich liquids becomes increasingly non-ideal, resulting in 2-liquid fields in which immiscible O-poor/O-rich or S-poor/S-rich liquids coexist (Urakawa et al., 1987; Chabot and Drake, 2000; Corgne et al., 2008; Dasgupta et al., 2009).
View in article
Conversely, C solubility increases with increasing Ni/Fe ratio, implying that the miscibility field in Figure 1 expands with increasing Ni content. P also reduces miscibility in Fe-S liquids (Chabot and Drake, 2000), although to a lesser extent than C (Fig. S-2b).
View in article
Magmatic iron meteorites are believed to have originated as the cores of differentiated, rocky planetesimals (Goldstein et al., 2009), and as they date from a few Myr after the onset of solar system accretion (Kleine et al., 2009; Spitzer et al., 2021), provide insight into the early stages of planetary formation (e.g., Chabot and Drake, 2000; Hirschmann et al., 2021; Grewal and Asimow, 2023; Grewal et al., 2024).
View in article
It is often assumed that solidification of planetesimal cores would progressively enrich remaining liquid in light elements, eventually resulting in immiscibility (e.g., Chabot and Drake, 2000; Corgne et al., 2008; Goldstein et al., 2009; Bercovici et al., 2022).
View in article
Corgne, A., Wood, B.J., Fei, Y. (2008) C- and S-rich molten alloy immiscibility and core formation of planetesimals. Geochimica et Cosmochimica Acta 72, 2409–2416. https://doi.org/10.1016/j.gca.2008.03.001

Towards lower pressures, mixing in Fe-rich liquids becomes increasingly non-ideal, resulting in 2-liquid fields in which immiscible O-poor/O-rich or S-poor/S-rich liquids coexist (Urakawa et al., 1987; Chabot and Drake, 2000; Corgne et al., 2008; Dasgupta et al., 2009).
View in article
In addition, although C has been shown to have a key role in reducing miscibility in Fe-rich liquids (Corgne et al., 2008; Dasgupta et al., 2009), these studies were conducted in C-saturated or C-rich systems, i.e. in systems not directly comparable to volatile-depleted planetesimals (Hirschmann et al., 2021).
View in article
Incorporation of minor O into run products is expected depending on oxygen fugacity, fO2, within experiments (Corgne et al., 2008; Dasgupta et al., 2009).
View in article
(b) Comparison with C-saturated/C-rich experiments, Green: Dasgupta et al. (2009) Fe, S, C system at 2 GPa, Light blue: Corgne et al. (2008) model chondritic system, and their miscibility curve at 4 GPa. Blue line: miscibility curve at 1 bar/1400 °C in the system Fe-S-C calculated using thermodynamic model of Tafwidli and Kang (2017); insert additionally shows fields of C-saturation.
View in article
Figure 1b shows comparison of our results with higher pressure C-saturated experiments (Corgne et al., 2008; Dasgupta et al., 2009) and the calculated 1 bar Fe-S-C phase diagram (Tafwidli and Kang, 2017).
View in article
It is often assumed that solidification of planetesimal cores would progressively enrich remaining liquid in light elements, eventually resulting in immiscibility (e.g., Chabot and Drake, 2000; Corgne et al., 2008; Goldstein et al., 2009; Bercovici et al., 2022).
View in article
Court, R.W., Sephton, M.A. (2014) New estimates of the production of volatile gases from ablating carbonaceous micrometeoroids at Earth and Mars during an E-belt-type Late Heavy Bombardment. Geochimica et Cosmochimica Acta 145, 175–205. https://doi.org/10.1016/j.gca.2014.09.010

This is supported by step heating experiments (Court and Sephton, 2014) which demonstrate that substantial C loss and minor S loss only occurs at low temperatures.
View in article
Dasgupta, R., Buono, A., Whelan, G., Walker, D. (2009) High-pressure melting relations in Fe-C-S systems: Implications for formation, evolution, and structure of metallic cores in planetary bodies. Geochimica et Cosmochimica Acta 73, 6678–6691. https://doi.org/10.1016/j.gca.2009.08.001

Towards lower pressures, mixing in Fe-rich liquids becomes increasingly non-ideal, resulting in 2-liquid fields in which immiscible O-poor/O-rich or S-poor/S-rich liquids coexist (Urakawa et al., 1987; Chabot and Drake, 2000; Corgne et al., 2008; Dasgupta et al., 2009).
View in article
Liquid immiscibility affects the composition of the cores of rocky bodies up to the size of Earth’s Moon, would result in core stratification (Dasgupta et al., 2009), and might influence core segregation processes (Bromiley, 2023).
View in article
In addition, although C has been shown to have a key role in reducing miscibility in Fe-rich liquids (Corgne et al., 2008; Dasgupta et al., 2009), these studies were conducted in C-saturated or C-rich systems, i.e. in systems not directly comparable to volatile-depleted planetesimals (Hirschmann et al., 2021).
View in article
Incorporation of minor O into run products is expected depending on oxygen fugacity, fO2, within experiments (Corgne et al., 2008; Dasgupta et al., 2009).
View in article
(b) Comparison with C-saturated/C-rich experiments, Green: Dasgupta et al. (2009) Fe, S, C system at 2 GPa, Light blue: Corgne et al. (2008) model chondritic system, and their miscibility curve at 4 GPa. Blue line: miscibility curve at 1 bar/1400 °C in the system Fe-S-C calculated using thermodynamic model of Tafwidli and Kang (2017); insert additionally shows fields of C-saturation.
View in article
Figure 1b shows comparison of our results with higher pressure C-saturated experiments (Corgne et al., 2008; Dasgupta et al., 2009) and the calculated 1 bar Fe-S-C phase diagram (Tafwidli and Kang, 2017).
View in article
Blue arrows denote modelled liquid compositions during crystallisation of 3 core-forming liquids. Due to the highly incompatible nature of S (e.g., Dasgupta et al., 2009), liquids become progressively S-rich with crystallisation.
View in article
Goldstein, J.I., Scott, E.R.D., Chabot, N.L. (2009) Iron meteorites: Crystallization, thermal history, parent bodies, and origin. Geochemistry 69, 293–325. https://doi.org/10.1016/j.chemer.2009.01.002

Differentiated asteroids, such as 4-Vesta, and the existence of iron meteorites, imply that core formation also occurred in a proportion of smaller bodies, possibly ranging down to a few 100 km in diameter (McCoy et al., 2006; Goldstein et al., 2009).
View in article
In addition to Fe and Ni, planetary cores contain variable concentrations of light elements: S, P, O, C, Si and H (Wood, 2008; Goldstein et al., 2009).
View in article
Starting mixes were prepared from high purity reagents, with variable S, P and C, based on a fixed Fe0.9Ni0.1 ratio, broadly representative of a range of planetesimal cores (Goldstein et al., 2009).
View in article
Magmatic iron meteorites are believed to have originated as the cores of differentiated, rocky planetesimals (Goldstein et al., 2009), and as they date from a few Myr after the onset of solar system accretion (Kleine et al., 2009; Spitzer et al., 2021), provide insight into the early stages of planetary formation (e.g., Chabot and Drake, 2000; Hirschmann et al., 2021; Grewal and Asimow, 2023; Grewal et al., 2024).
View in article
There are various models for reconstructing compositions of magmatic iron meteorite parental liquids (IMPLs), although results from these are broadly consistent, at least for elements such as S, which have a strong influence on element partitioning (e.g., Goldstein et al., 2009; Hilton et al., 2022; Zhang et al., 2024).
View in article
It is often assumed that solidification of planetesimal cores would progressively enrich remaining liquid in light elements, eventually resulting in immiscibility (e.g., Chabot and Drake, 2000; Corgne et al., 2008; Goldstein et al., 2009; Bercovici et al., 2022).
View in article
Grewal, D.S. (2022) Origin of nitrogen isotopic variations in the rocky bodies of the solar system. The Astrophysical Journal 937, 123. https://doi.org/10.3847/1538-4357/ac8eb4

Similarly, correlations between bulk C and N in chondrites and peak metamorphic temperatures have been argued to imply that C loss occurred at low temperatures (<200 °C) (Grewal, 2022; Grewal et al. 2022).
View in article
Grewal, D.S., Seales, J.D., Dasgupta, R. (2022) Internal or external magma oceans in the earliest protoplanets – Perspectives from nitrogen and carbon fractionation. Earth and Planetary Science Letters 598, 117847. https://doi.org/10.1016/j.epsl.2022.117847

Similarly, correlations between bulk C and N in chondrites and peak metamorphic temperatures have been argued to imply that C loss occurred at low temperatures (<200 °C) (Grewal, 2022; Grewal et al. 2022).
View in article
Significant volatile loss would occur on planetesimals which underwent largescale melting, particularly for those with external as opposed to internal magma oceans (Hirschmann et al., 2021; Grewal et al., 2022).
View in article
Grewal, D.S., Asimow, P.D. (2023) Origin of the superchondritic carbon/nitrogen ratio of the bulk silicate Earth – an outlook from iron meteorites. Geochimica et Cosmochimica Acta 344, 146–159. https://doi.org/10.1016/j.gca.2023.01.012

Here, we describe superliquidus experiments which constrain liquid immiscibility in the system Fe, Ni, S, P, C, O from 0.5 to 1 GPa, at 1673 K, i.e. under conditions relevant to differentiation in planetesimals a few 100 km in diameter (Grewal and Asimow, 2023).
View in article
Magmatic iron meteorites are believed to have originated as the cores of differentiated, rocky planetesimals (Goldstein et al., 2009), and as they date from a few Myr after the onset of solar system accretion (Kleine et al., 2009; Spitzer et al., 2021), provide insight into the early stages of planetary formation (e.g., Chabot and Drake, 2000; Hirschmann et al., 2021; Grewal and Asimow, 2023; Grewal et al., 2024).
View in article
(a) Blue circles: magmatic iron meteorite ‘parent core’ compositions from Hilton et al. (2022) with C contents from Grewal and Asimow (2023).
View in article
Regardless, in comparison IMPL compositions are volatile-poor (Hilton et al., 2022; Grewal and Asimow, 2023), and record signficant C loss, and moderate S and P loss compared to model chondrite core compositions.
View in article
Here, we use data from Grewal and Asimow (2023) and Zhai et al. (2022) to model incremental extraction of small batches of solid Fe, Ni from single liquid planetesimal core-forming liquids (Fig. 2c; see Supplementary Information for details).
View in article
Grewal, D.S., Nie, N.X., Zhang, B., Izidoro, A., Asimow, P.D. (2024) Accretion of the earliest inner Solar System planetesimals beyond the water snowline. Nature Astronomy 8, 290–297. https://doi.org/10.1038/s41550-023-02172-w

Magmatic iron meteorites are believed to have originated as the cores of differentiated, rocky planetesimals (Goldstein et al., 2009), and as they date from a few Myr after the onset of solar system accretion (Kleine et al., 2009; Spitzer et al., 2021), provide insight into the early stages of planetary formation (e.g., Chabot and Drake, 2000; Hirschmann et al., 2021; Grewal and Asimow, 2023; Grewal et al., 2024).
View in article
Hilton, C.D., Ash, R.D., Walker, R.J. (2022) Chemical characteristics of iron meteorite parent bodies. Geochimica et Cosmochimica Acta 318, 112–125. https://doi.org/10.1016/j.gca.2021.11.035

There are various models for reconstructing compositions of magmatic iron meteorite parental liquids (IMPLs), although results from these are broadly consistent, at least for elements such as S, which have a strong influence on element partitioning (e.g., Goldstein et al., 2009; Hilton et al., 2022; Zhang et al., 2024).
View in article
(a) Blue circles: magmatic iron meteorite ‘parent core’ compositions from Hilton et al. (2022) with C contents from Grewal and Asimow (2023).
View in article
Regardless, in comparison IMPL compositions are volatile-poor (Hilton et al., 2022; Grewal and Asimow, 2023), and record signficant C loss, and moderate S and P loss compared to model chondrite core compositions.
View in article
Hirschmann, M.M., Bergin, E.A., Blake, G.A., Ciesla, F.J., Li, J. (2021) Early volatile depletion on planetesimals inferred from C-S systematics of iron meteorite parent bodies. Proceedings of the National Academy of Sciences 118, e2026779118. https://doi.org/10.1073/pnas.2026779118

In addition, although C has been shown to have a key role in reducing miscibility in Fe-rich liquids (Corgne et al., 2008; Dasgupta et al., 2009), these studies were conducted in C-saturated or C-rich systems, i.e. in systems not directly comparable to volatile-depleted planetesimals (Hirschmann et al., 2021).
View in article
Magmatic iron meteorites are believed to have originated as the cores of differentiated, rocky planetesimals (Goldstein et al., 2009), and as they date from a few Myr after the onset of solar system accretion (Kleine et al., 2009; Spitzer et al., 2021), provide insight into the early stages of planetary formation (e.g., Chabot and Drake, 2000; Hirschmann et al., 2021; Grewal and Asimow, 2023; Grewal et al., 2024).
View in article
Hirschmann et al. (2021) argued that compositions of IMPLs largely reflect the primary volatile content of planetesimal cores.
View in article
By contrast, volatile loss from unshielded planetesimal cores, i.e. following planetesimal disruption, would result in preferential S loss and an increase in C/S (Hirschmann et al., 2021).
View in article
Compositions of carbonaceous and ordinary chondrites as a function of petrological type (left), and for specific groups (right), after Hirschmann et al. (2021).
View in article
Significant volatile loss would occur on planetesimals which underwent largescale melting, particularly for those with external as opposed to internal magma oceans (Hirschmann et al., 2021; Grewal et al., 2022).
View in article
Hunt, A.C., Theis, K.J., Rehkämper, M., Benedix, G.K., Andreasen, R., Schönbächler, M. (2022) The dissipation of the solar nebula constrained by impacts and core cooling in planetesimals. Nature Astronomy 6, 812–818. https://doi.org/10.1038/s41550-022-01675-2

However, cooling rates, isotope systematics and MVE contents suggest that, at least, some groups evidence degassing after core segregation and following planetesimal disruption (e.g., Yang et al., 2007; Matthes et al., 2018; Hunt et al., 2022; Rubin et al., 2022; Zhang et al., 2024).
View in article
Kaminski, E., Limare, A., Kenda, B., Chaussidon, M. (2020) Early accretion of planetesimals unraveled by the thermal evolution of the parent bodies of magmatic iron meteorites. Earth and Planetary Science Letters 548, 116496. https://doi.org/10.1016/j.epsl.2020.116469

The extent of planetesimal degassing will depend on thermal history and differentiation processes (e.g., Kaminski et al., 2020).
View in article
However, some thermal modelling suggests that silicate melting in planetesimals would be limited in extent and duration (Kaminski et al., 2020; see Supplementary Information), and it is argued that core formation in some planetesimals could proceed without large-scale silicate melting (e.g., Bromiley, 2023).
View in article
Kleine, T., Touboul, M., Bourdon, B., Nimmo, F., Mezger, K., Palme, H., Jacobsen, S.B., Yin, Q.Z., Halliday, A.N. (2009) Hf-W chronology of the accretion and early evolution of asteroids and terrestrial planets. Geochimica et Cosmochimica Acta 73, 5150–5188. https://doi.org/10.1016/j.gca.2008.11.047

Intriguingly, isotopic data from iron meteorites imply formation ages within a few Myr of the onset of accretion in the solar system (Kleine et al., 2009).
View in article
Magmatic iron meteorites are believed to have originated as the cores of differentiated, rocky planetesimals (Goldstein et al., 2009), and as they date from a few Myr after the onset of solar system accretion (Kleine et al., 2009; Spitzer et al., 2021), provide insight into the early stages of planetary formation (e.g., Chabot and Drake, 2000; Hirschmann et al., 2021; Grewal and Asimow, 2023; Grewal et al., 2024).
View in article
Mare, E.R., Tomkins, A.G., Godel, B.M. (2014) Restriction of parent body heating by metal-troilite melting: Thermal models for the ordinary chondrites. Meteoritics and Planetary Science 49, 636–651. https://doi.org/10.1111/maps.12280

Variability in the C content of chondrites reflects low temperature degassing processes. However, as some ordinary chondrites evidence peak metamorphic temperatures up to the onset of FeS melting close to 1000 °C (Mare et al., 2014), chondrite compositions are useful in assessing total volatile content in planetesimals prior to/at the onset of core formation.
View in article
Matthes, M., Fischer-Gödde, M., Kruijer, T.S., Kleine, T. (2018) Pd-Ag chronometry of IVA iron meteorites and the crystallization and cooling of a protoplanetary core. Geochimica et Cosmochimica Acta 220, 82–95. https://doi.org/10.1016/j.gca.2017.09.009

However, cooling rates, isotope systematics and MVE contents suggest that, at least, some groups evidence degassing after core segregation and following planetesimal disruption (e.g., Yang et al., 2007; Matthes et al., 2018; Hunt et al., 2022; Rubin et al., 2022; Zhang et al., 2024).
View in article
McCoy, T.J., Mittlefehldt, D.W., Wilson, L. (2006) Asteroid Differentiation. In: Dante, S.L., McSween, H.Y. (Eds.) Meteorites and the Early Solar System II. University of Arizona Press, 733-746. https://doi.org/10.2307/j.ctv1v7zdmm.40

Differentiated asteroids, such as 4-Vesta, and the existence of iron meteorites, imply that core formation also occurred in a proportion of smaller bodies, possibly ranging down to a few 100 km in diameter (McCoy et al., 2006; Goldstein et al., 2009).
View in article
Melwani Daswani, M., Vance, S.D., Mayne, M.J., Glein, C.R. (2021) A Metamorphic Origin for Europa’s Ocean. Geophysical Research Letters 48, e2021GL094143. https://doi.org/10.1029/2021GL094143

One pertinent illustration of this is segregation processes in icy/rocky bodies in the outer solar system. It has been suggested (e.g., Melwani Daswani et al., 2021) that some icy moons have bulk compositions close to those of carbonaceous chondrites, and formed following slow heating and segregation of rock/ice/metal over 10s–100s Myr timescales.
View in article
Rubin, A.E., Zhang, B., Chabot, N.L. (2022) IVA iron meteorites as late-stage crystallization products affected by multiple collisional events. Geochimica et Cosmochimica Acta 331, 1–17. https://doi.org/10.1016/j.gca.2022.05.020

However, cooling rates, isotope systematics and MVE contents suggest that, at least, some groups evidence degassing after core segregation and following planetesimal disruption (e.g., Yang et al., 2007; Matthes et al., 2018; Hunt et al., 2022; Rubin et al., 2022; Zhang et al., 2024).
View in article
Spitzer, F., Burkhardt, C., Nimmo, F., Kleine, T. (2021) Nucleosynthetic Pt isotope anomalies and the Hf-W chronology of core formation in inner and outer solar system planetesimals. Earth and Planetary Science Letters 576, 117211. https://doi.org/10.1016/j.epsl.2021.117211

Magmatic iron meteorites are believed to have originated as the cores of differentiated, rocky planetesimals (Goldstein et al., 2009), and as they date from a few Myr after the onset of solar system accretion (Kleine et al., 2009; Spitzer et al., 2021), provide insight into the early stages of planetary formation (e.g., Chabot and Drake, 2000; Hirschmann et al., 2021; Grewal and Asimow, 2023; Grewal et al., 2024).
View in article
Tafwidli, F., Kang, Y. (2017) Thermodynamic Modeling of Fe–C–S Ternary System. Isij International 57, 782–790. https://doi.org/10.2355/isijinternational.ISIJINT-2016-672

(b) Comparison with C-saturated/C-rich experiments, Green: Dasgupta et al. (2009) Fe, S, C system at 2 GPa, Light blue: Corgne et al. (2008) model chondritic system, and their miscibility curve at 4 GPa. Blue line: miscibility curve at 1 bar/1400 °C in the system Fe-S-C calculated using thermodynamic model of Tafwidli and Kang (2017); insert additionally shows fields of C-saturation.
View in article
Figure 1b shows comparison of our results with higher pressure C-saturated experiments (Corgne et al., 2008; Dasgupta et al., 2009) and the calculated 1 bar Fe-S-C phase diagram (Tafwidli and Kang, 2017).
View in article
However, coincidence of our Fe0.9Ni0.1 miscibility curve at 0.5/1 GPa with the 1 bar Fe-S-C miscibility curve from Tafwidli and Kang (2017), suggests that wt. % concentrations of P and O signficantly reduce miscibility.
View in article
Urakawa, S., Kato, M., Kumazawa, M. (1987) Experimental Study on the Phase Relations in the System Fe-Ni-O-S Up to 15 GPa. In: Manghnani, M.H. and Syono, Y. (Eds.) High‐Pressure Research in Mineral Physics: A Volume in Honor of Syun‐iti Akimoto. American Geophysical Union (AGU), 95–111. https://doi.org/10.1029/GM039p0095

Towards lower pressures, mixing in Fe-rich liquids becomes increasingly non-ideal, resulting in 2-liquid fields in which immiscible O-poor/O-rich or S-poor/S-rich liquids coexist (Urakawa et al., 1987; Chabot and Drake, 2000; Corgne et al., 2008; Dasgupta et al., 2009).
View in article
Wood, B.J. (2008) Accretion and core formation: constraints from metal-silicate partitioning. Philosophical Transactions of the Royal Society a-Mathematical Physical and Engineering Sciences 366, 4339–4355. https://doi.org/10.1098/rsta.2008.0115

Separation of Fe-rich, core-forming liquids from silicate was a defining process in planetary formation in the inner solar system, and left a geochemical signature on the cores and mantles of rocky planets (Wood, 2008).
View in article
In addition to Fe and Ni, planetary cores contain variable concentrations of light elements: S, P, O, C, Si and H (Wood, 2008; Goldstein et al., 2009).
View in article
Yang, J., Goldstein, J.I., Scott, E.R.D. (2007) Iron meteorite evidence for early formation and catastrophic disruption of protoplanets. Nature 446, 888–891. https://doi.org/10.1038/nature05735

However, cooling rates, isotope systematics and MVE contents suggest that, at least, some groups evidence degassing after core segregation and following planetesimal disruption (e.g., Yang et al., 2007; Matthes et al., 2018; Hunt et al., 2022; Rubin et al., 2022; Zhang et al., 2024).
View in article
Zhai, K., Yin, Y., Zhai, S. (2022) New constraints on the abundances of phosphorus and sulfur in the lunar core: High-pressure and high-temperature experimental study of the FeSP ternary system. Geochimica et Cosmochimica Acta 334, 1–13. https://doi.org/10.1016/j.gca.2022.07.024

Here, we use data from Grewal and Asimow (2023) and Zhai et al. (2022) to model incremental extraction of small batches of solid Fe, Ni from single liquid planetesimal core-forming liquids (Fig. 2c; see Supplementary Information for details).
View in article
Zhang, Z., Hastings, P., Von der Handt, A., Hirschmann, M.M. (2018) Experimental determination of carbon solubility in Fe-Ni-S melts. Geochimica et Cosmochimica Acta 225, 66–79. https://doi.org/10.1016/j.gca.2018.01.009

Dashed black line: pressure-independent C solubility in Fe/Ni-S liquid at 2–7 GPa (Zhang et al., 2018).
View in article
This mirrors the compositional dependence of C solubility in Fe/Ni/S liquids, which is markedly lower at higher S contents (Zhang et al., 2018; Fig. 1b).
View in article
Zhang, B., Chabot, N.L., Rubin, A.E. (2024) Compositions of iron-meteorite parent bodies constrain the structure of the protoplanetary disk. Proceedings of the National Academy of Sciences 121, e2306995121. https://doi.org/10.1073/pnas.2306995121

There are various models for reconstructing compositions of magmatic iron meteorite parental liquids (IMPLs), although results from these are broadly consistent, at least for elements such as S, which have a strong influence on element partitioning (e.g., Goldstein et al., 2009; Hilton et al., 2022; Zhang et al., 2024).
View in article
However, cooling rates, isotope systematics and MVE contents suggest that, at least, some groups evidence degassing after core segregation and following planetesimal disruption (e.g., Yang et al., 2007; Matthes et al., 2018; Hunt et al., 2022; Rubin et al., 2022; Zhang et al., 2024).
View in article
top
Supplementary Information
The Supplementary Information includes:
- Methodology
- Results from super-liquidus experiments
- Modelling planetesimal core compositions
- Tables S-1 to S-4
- Figure S-1 and S-2
- Supplementary Information References
Download the Supplementary Information (PDF)
Figures
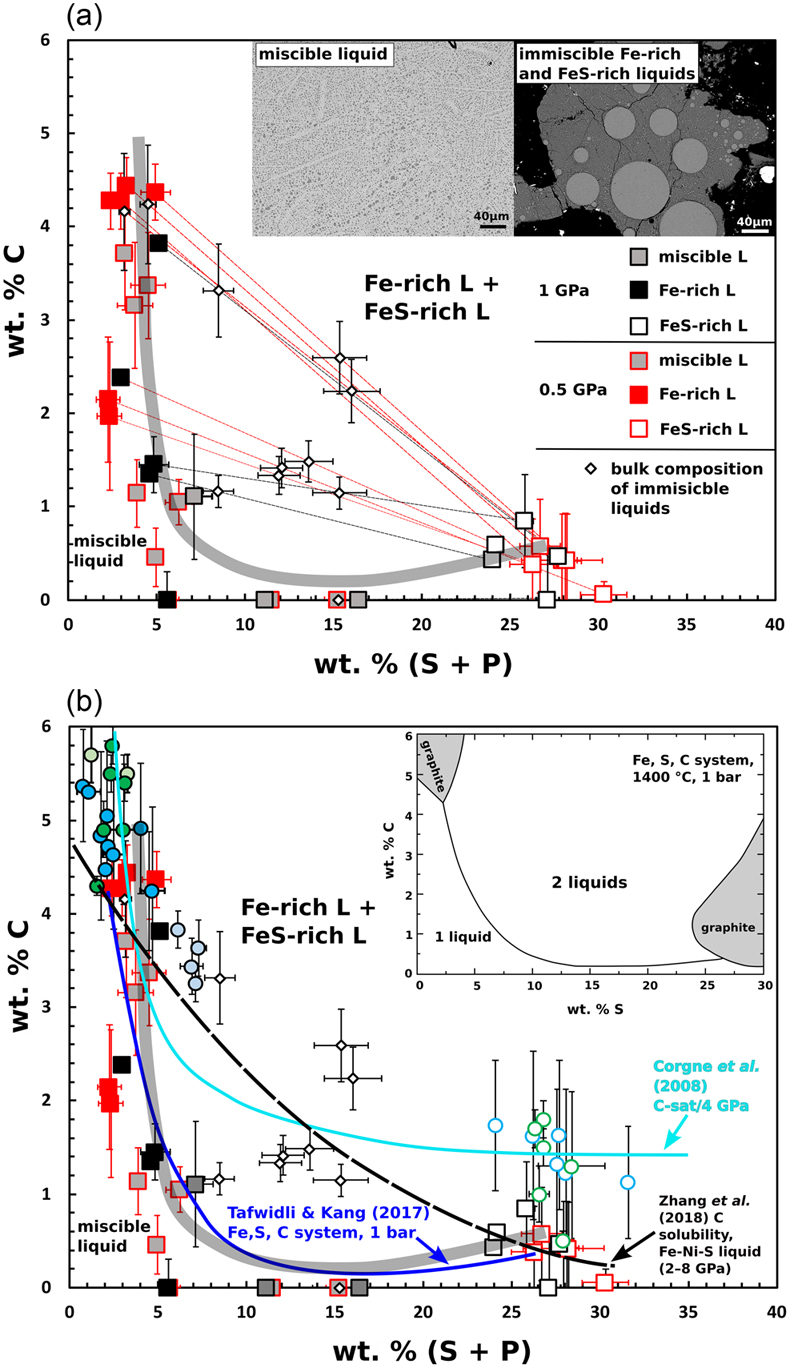
Figure 1 (a) Liquid compositions in the system Fe0.9Ni0.1, S, P, C, O at 0.5 (red)/1.0 GPa (black), and calculated bulk compositions for immiscible liquids. Tie-lines connect compositions of coexisting immiscible liquids. Insert: examples of run products (see Fig. S-1 for details). (b) Comparison with C-saturated/C-rich experiments, Green: Dasgupta et al. (2009)
Dasgupta, R., Buono, A., Whelan, G., Walker, D. (2009) High-pressure melting relations in Fe-C-S systems: Implications for formation, evolution, and structure of metallic cores in planetary bodies. Geochimica et Cosmochimica Acta 73, 6678–6691. https://doi.org/10.1016/j.gca.2009.08.001
Fe, S, C system at 2 GPa, Light blue: Corgne et al. (2008)Corgne, A., Wood, B.J., Fei, Y. (2008) C- and S-rich molten alloy immiscibility and core formation of planetesimals. Geochimica et Cosmochimica Acta 72, 2409–2416. https://doi.org/10.1016/j.gca.2008.03.001
model chondritic system, and their miscibility curve at 4 GPa. Blue line: miscibility curve at 1 bar/1400 °C in the system Fe-S-C calculated using thermodynamic model of Tafwidli and Kang (2017)Tafwidli, F., Kang, Y. (2017) Thermodynamic Modeling of Fe–C–S Ternary System. Isij International 57, 782–790. https://doi.org/10.2355/isijinternational.ISIJINT-2016-672
; insert additionally shows fields of C-saturation. Dashed black line: pressure-independent C solubility in Fe/Ni-S liquid at 2–7 GPa (Zhang et al., 2018Zhang, Z., Hastings, P., Von der Handt, A., Hirschmann, M.M. (2018) Experimental determination of carbon solubility in Fe-Ni-S melts. Geochimica et Cosmochimica Acta 225, 66–79. https://doi.org/10.1016/j.gca.2018.01.009
).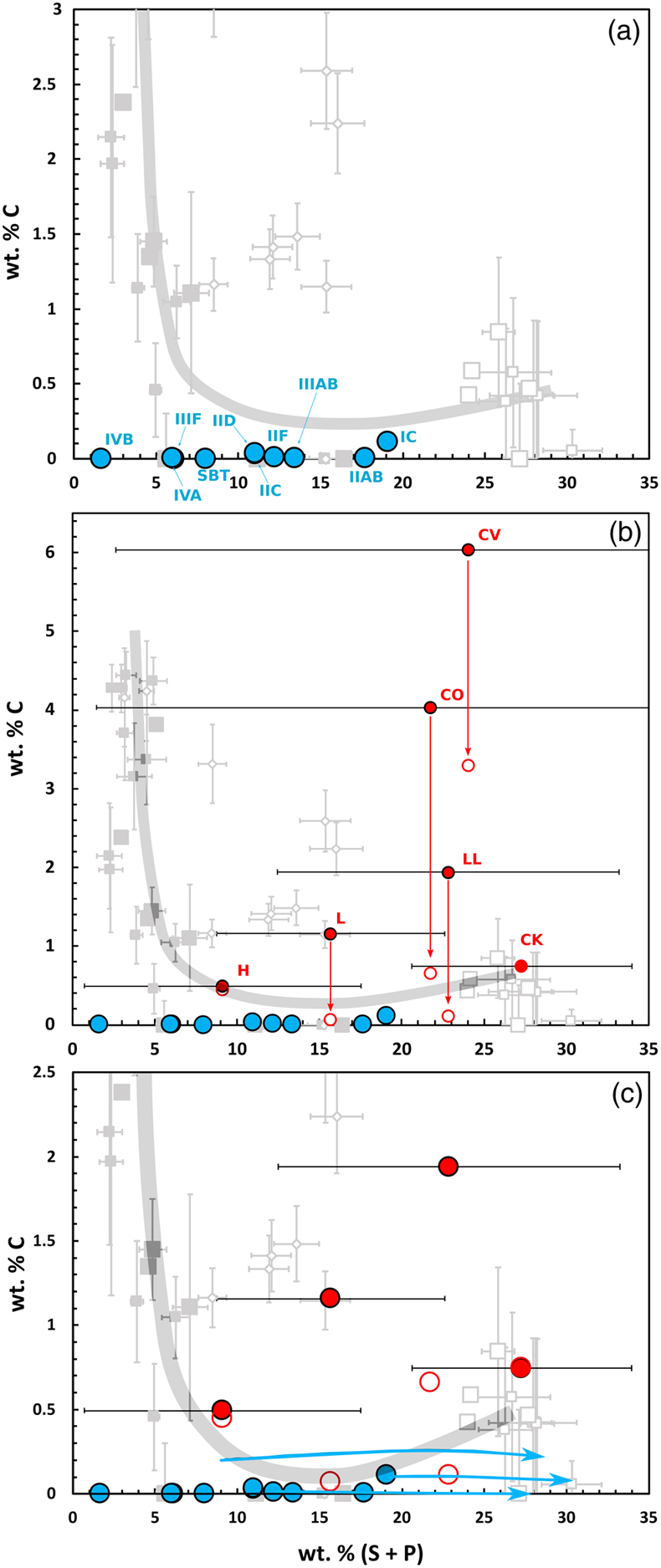
Figure 2 Model planetesimal core compositions; data from Figure 1b shown in light grey for clarity. (a) Blue circles: magmatic iron meteorite ‘parent core’ compositions from Hilton et al. (2022)
Hilton, C.D., Ash, R.D., Walker, R.J. (2022) Chemical characteristics of iron meteorite parent bodies. Geochimica et Cosmochimica Acta 318, 112–125. https://doi.org/10.1016/j.gca.2021.11.035
with C contents from Grewal and Asimow (2023)Grewal, D.S., Asimow, P.D. (2023) Origin of the superchondritic carbon/nitrogen ratio of the bulk silicate Earth – an outlook from iron meteorites. Geochimica et Cosmochimica Acta 344, 146–159. https://doi.org/10.1016/j.gca.2023.01.012
. (b) Red circles: modelled planetesimal core compositions based on metal/silicate differentiation of averaged chondrite compositions, after Bercovici et al. (2022)Bercovici, H.L., Elkins-Tanton, L.T., O’Rourke, J.G., Schaefer, L. (2022) The effects of bulk composition on planetesimal core sulfur content and size. Icarus 380, 114976. https://doi.org/10.1016/j.icarus.2022.114976
. Error bars are propagated from uncertainties in S and P contents. Open circles: corresponding ‘minimum’ C core compositions for each chondrite group based on variability in chondrite C contents for petrological types 3–6 (Fig. 3). (c) Blue arrows: modelled liquid compositions with progressive crystallisation of solid Fe/Ni.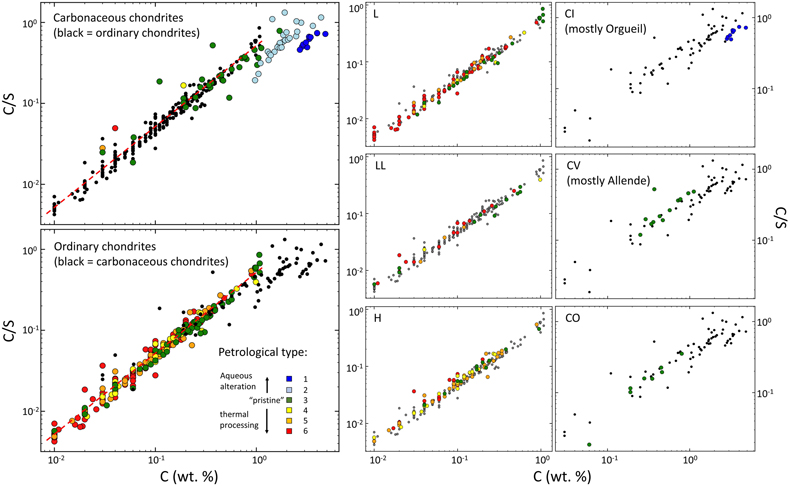
Figure 3 Compositions of carbonaceous and ordinary chondrites as a function of petrological type (left), and for specific groups (right), after Hirschmann et al. (2021)
Hirschmann, M.M., Bergin, E.A., Blake, G.A., Ciesla, F.J., Li, J. (2021) Early volatile depletion on planetesimals inferred from C-S systematics of iron meteorite parent bodies. Proceedings of the National Academy of Sciences 118, e2026779118. https://doi.org/10.1073/pnas.2026779118
. Within each group, colour symbols denote petrological type. Small, black symbols are all other ordinary and carbonaceous chondrite data, for comparison. Red dashed line is a 1:1 fit to data (types 3–6). Compositions from the Metbase (2024) database for samples with combined bulk C and S, and clear petrologic type (http://www.metbase.org, GeoPlatform UG, Germany).