Evidence of sub-arc mantle oxidation by sulphur and carbon
Affiliations | Corresponding Author | Cite as | Funding informationKeywords: oxygen fugacity, XANES, sub-arc mantle, sulphur, carbon, porphyry
- Share this article
Article views:11,074Cumulative count of HTML views and PDF downloads.
- Download Citation
- Rights & Permissions
Abstract

Figures and Tables
![]() Figure 1 Example of studied metasomatised harzburgite from the Bardane peridotite. (a) Scanned thin section of sample BDN03 showing metasomatic garnet (Grt), orthopyroxene (Opx), phlogopite (Phl) and clinopyroxene (Cpx). (b) Photomicrograph showing exsolved needles of Cpx and Opx in the garnet crystal, testifying to a majoritic precursor. | ![]() Figure 2 Multi-phase solid inclusions in BDN03 garnet. Back scattered electron (BSE) images showing: (a) MSI containing phlogopite and barite; (b) MSI containing magnesite, phlogopite, apatite and monazite; (c) pentlandite inclusions in garnet; (d) garnet-enclosed graphite. | ![]() Figure 3 Comparison between metasomatic garnet from Bardane (BDN03) and garnet from Ugelvik (UGL01). (a) Compositional map of sample UGL01 showing variations in the concentration of K (red; not detected), Ba (green; not detected) and Mn (blue; 0 to 0.46 wt. % ± 133 ppm), indicating a lack of metasomatic minerals. Green spots represent the sites chosen for the acquisition of XANES spectra. (b) Results of XANES analysis comparing the Fe3+/Fetot ratio in primary garnet in UGL01 with metasomatic garnet in BDN03. Error bar is shown in the lower right-hand corner. (c) Compositional map of sample BDN03 showing variations in the concentration of K (red; 0 to 14.1 wt. % ± 0.7 wt. %), Ba (green; 0 to 11.6 wt. % ± 0.3 wt. %) and Mn (blue; 0 to 1.0 wt. % ± 0.1 wt. %). Yellow dots are locations where XANES spectra were acquired. Phlogopite is highlighted by elevated K (red), and barite-rich inclusions by elevated Ba (green). The yellow–orange tinted areas highlight the coexistence of barite and phlogopite. | ![]() Figure 4 Oxygen fugacity of orogenic peridotites. Variation in ƒO2 with depth is reported for a model depleted mantle (green line) and the stability of carbonate vs. graphite/diamond is shown by the EMOD/G (blue line). Dashed line is a regression line at pressures where it was not possible to directly calculate ƒO2. ƒO2 for UGL01 is represented by green dots and the green bold line represents the variation range. BDN03 ƒO2 is reported in yellow while in orange is the ƒO2 of metasomatised orogenic peridotites from Malaspina et al. (2009) and Malaspina et al. (2010). Literature data have been recalculated using the Miller et al. (2016) oxybarometer. An error bar for the ƒO2 is reported in the lower right-hand side of the diagram. |
Figure 1 | Figure 2 | Figure 3 | Figure 4 |
Supplementary Figures and Tables
![]() Figure S-1 Compositional maps showing the distribution of barium (green) and manganese (blue) in garnet BDN03. The manganese distribution highlights the garnet crystal whilst barium indicate the presence of barite hosted in the garnet and the high content of Ba in phlogopite, this latter is visible in the upper-right side of the figure. Ba concentration 0–24 wt. % ± 0.4 wt. %, Mn concentration 0–0.87 wt. % ± 178 ppm. | ![]() Figure S-2 Compositional maps showing the distribution of barium (green) and manganese (blue) in garnet UGL01. Mn concentration 0–0.59 wt. % ± 240 ppm. Ba has not been detected. | ![]() Figure S-3 Fe K-edge XANES spectra for BDN03 and UGL01. | ![]() Figure S-4 Calibration line (line of best fit) correlating the intensity ratio of standards at 7138.4 and 7161.7 eV in the XANES spectra with Fe3+/Fetot measured by Mössbauer spectroscopy. | ![]() Table S-1 Major and minor element compositions of garnet (Grt), olivine (Ol) and orthopyroxene (Opx) from samples BDN03 and UGL01. The Fe3+/ Fetot of garnet is also reported, together with the pressure and temperature used for the calculation of ƒO2. | ![]() Table S-2 Results of Fe K-edge XANES spectroscopy analyses. The intensities at 7138.4 eV and 7161.7 eV measured in garnet standards are reported with their ratio and the respective Fe3+/Fetot determined by Mössbauer spectroscopy. Intensities in garnet from samples UGL01 and BDN03 are reported together with their Fe3+/Fetot retrieved using the calibration line in Figure S-4. |
Figure S-1 | Figure S-2 | Figure S-3 | Figure S-4 | Table S-1 | Table S-2 |
top
Letter
Plate tectonics drives recycling of surface material into the Earth’s interior, introducing hydrated and oxidised oceanic lithosphere into the mantle at subduction zones (Wood et al., 1990
Wood, B.J., Bryndzia, L.T., Johnson, E.K. (1990) Mantle oxidation state and its relationship to tectonic environment and fluid speciation. Science 248, 337–345.
; Evans, 2012Evans, K.A. (2012) The redox budget of subduction zones. Earth-Science Reviews 113, 11–32.
). Fluids liberated during dehydration of subducted crust trigger partial melting of the overlying mantle leading to the formation of volcanic arcs, dominated by oxidised rocks (Ballhaus, 1993Ballhaus, C. (1993) Redox states of lithospheric and asthenospheric upper mantle. Contributions to Mineralogy and Petrology 114, 331–348.
; Kelley and Cottrell, 2009Kelley, K.A., Cottrell, E. (2009) Water and oxidation state of subduction zone magmas. Science 325, 605–607.
). Correlation between oxidation of arc lavas and addition of slab material by aqueous fluids to their mantle source has been quantitatively established (Kelley and Cottrell, 2009Kelley, K.A., Cottrell, E. (2009) Water and oxidation state of subduction zone magmas. Science 325, 605–607.
; Brounce et al., 2014Brounce, M.N., Kelley, K.A., Cottrell, E. (2014) Variations in Fe3+/ Fe of Mariana Arc basalts and mantle wedge ƒO2. Journal of Petrology 55, 2513–2536.
), indicating that slab fluids are somehow responsible for the observed oxidation. However, although calculations suggest that fluid-borne oxidised sulphur may be important (Evans and Tomkins, 2011Evans, K.A., Tomkins, A.G. (2011) The relationship between subduction zone redox budget and arc magma fertility. Earth and Planetary Science Letters 308, 401–409.
), the process has not been resolved with physical evidence. Determination of redox processes associated with fluid–mantle interaction cannot be indisputably resolved by studying the product of the arc system (i.e. arc lavas); it is best approached by examining rocks from the deep portion of mantle wedge close to the slab–mantle interface that have been altered by slab fluids, which are preserved as orogenic peridotites.The Western Gneiss Region of Norway hosts several orogenic peridotite bodies that represent a portion of transition zone mantle that upwelled, melted (Stage M1) and accreted to cratonic lithosphere (Stage M2; Spengler et al., 2006
Spengler, D., van Roermund, H.L., Drury, M.R., Ottolini, L., Mason, P.R., Davies, G.R. (2006) Deep origin and hot melting of an Archaean orogenic peridotite massif in Norway. Nature 440, 913–17.
). Subsequently, during the Caledonian orogeny, fluids derived from subducting slab locally promoted metasomatism (Stage M3), as recorded by the Bardane peridotite on Fjørtoft island (Brueckner et al., 2002Brueckner, K.H., Carswell, D.A., Griffin, W.L. (2002) Paleozoic diamonds within a Precambrian peridotite lens in UHP gneisses of the Norwegian Caledonides. Earth and Planetary Science Letters 203, 805–816.
; Van Roermund et al., 2002Van Roermund, H.L.M., Carswell, D.A., Drury, M.R., Heijboer, T.C. (2002) Microdiamonds in megacrystic garnet websterite pod from Bardane on the island of Fjortft, western Norway: Evidence for diamond formation in mantle rocks during deep continental subduction. Geology 30, 959–962.
; Scambelluri et al., 2008Scambelluri, M., Pettke, T., van Roermund, H.L.M. (2008) Majoritic garnets monitor deep subduction fluid flow and mantle dynamics. Geology 36, 59–62.
). Other portions have not been infiltrated by slab fluids and preserve the original mantle assemblage (M1-M2), exemplified by the Ugelvik peridotite on the island of Otrøy (Brueckner et al., 2002Brueckner, K.H., Carswell, D.A., Griffin, W.L. (2002) Paleozoic diamonds within a Precambrian peridotite lens in UHP gneisses of the Norwegian Caledonides. Earth and Planetary Science Letters 203, 805–816.
; Spengler et al., 2006Spengler, D., van Roermund, H.L., Drury, M.R., Ottolini, L., Mason, P.R., Davies, G.R. (2006) Deep origin and hot melting of an Archaean orogenic peridotite massif in Norway. Nature 440, 913–17.
). Although there is debate on the origin of metasomatism at Bardane, the trace element and isotopic signature of the metasomatic assemblage are comparable to the effects of typical subduction zone fluids sensu lato (cf. Brueckner et al., 2002Brueckner, K.H., Carswell, D.A., Griffin, W.L. (2002) Paleozoic diamonds within a Precambrian peridotite lens in UHP gneisses of the Norwegian Caledonides. Earth and Planetary Science Letters 203, 805–816.
; Scambelluri et al., 2008Scambelluri, M., Pettke, T., van Roermund, H.L.M. (2008) Majoritic garnets monitor deep subduction fluid flow and mantle dynamics. Geology 36, 59–62.
). The Bardane peridotite represents the best-known analogue for the study of fluid-related redox processes at sub-arc depth.Previous studies at Bardane focused on a megacrystic garnet–websterite lens (e.g., Brueckner et al., 2002
Brueckner, K.H., Carswell, D.A., Griffin, W.L. (2002) Paleozoic diamonds within a Precambrian peridotite lens in UHP gneisses of the Norwegian Caledonides. Earth and Planetary Science Letters 203, 805–816.
) and showed that interaction between slab fluids and depleted mantle generated metasomatic orthopyroxene, clinopyroxene, spinel, diamond (Van Roermund et al., 2002Van Roermund, H.L.M., Carswell, D.A., Drury, M.R., Heijboer, T.C. (2002) Microdiamonds in megacrystic garnet websterite pod from Bardane on the island of Fjortft, western Norway: Evidence for diamond formation in mantle rocks during deep continental subduction. Geology 30, 959–962.
) and majoritic garnet, which indicate peak conditions of ~6.5 GPa and 800–1000 °C (M3-3 stage; Scambelluri et al., 2008Scambelluri, M., Pettke, T., van Roermund, H.L.M. (2008) Majoritic garnets monitor deep subduction fluid flow and mantle dynamics. Geology 36, 59–62.
). Multi-phase solid inclusions (MSI) are hosted in M3 minerals and are thought to have crystallised from slab fluids that permeated the peridotite and contain phlogopite, spinel, amphibole, dolomite, monazite, magnesite, graphite and diamond (Van Roermund et al., 2002Van Roermund, H.L.M., Carswell, D.A., Drury, M.R., Heijboer, T.C. (2002) Microdiamonds in megacrystic garnet websterite pod from Bardane on the island of Fjortft, western Norway: Evidence for diamond formation in mantle rocks during deep continental subduction. Geology 30, 959–962.
; Malaspina et al., 2010Malaspina, N., Scambelluri, M., Poli, S., Van Roermund, H.L.M., Langenhorst, F. (2010) The oxidation state of mantle wedge majoritic garnet websterites metasomatised by C-bearing subduction fluids. Earth and Planetary Science Letters 298, 417–426.
).We found a new occurrence of majoritic garnet as megacrysts in the Bardane peridotite coexisting with clinopyroxene, orthopyroxene, phlogopite and spinel hosted in harzburgitic domains (Fig. 1a). The garnet megacrysts contain sparse exsolved clinopyroxene and orthopyroxene needles (Fig. 1b) with similar size, abundance and distribution to the exsolution previously reported for M3 garnet (Scambelluri et al., 2008
Scambelluri, M., Pettke, T., van Roermund, H.L.M. (2008) Majoritic garnets monitor deep subduction fluid flow and mantle dynamics. Geology 36, 59–62.
). MSI are abundant in the garnet (Fig. 3c). In addition to the previously reported mineralogy (Van Roermund et al., 2002Van Roermund, H.L.M., Carswell, D.A., Drury, M.R., Heijboer, T.C. (2002) Microdiamonds in megacrystic garnet websterite pod from Bardane on the island of Fjortft, western Norway: Evidence for diamond formation in mantle rocks during deep continental subduction. Geology 30, 959–962.
; Malaspina et al., 2010Malaspina, N., Scambelluri, M., Poli, S., Van Roermund, H.L.M., Langenhorst, F. (2010) The oxidation state of mantle wedge majoritic garnet websterites metasomatised by C-bearing subduction fluids. Earth and Planetary Science Letters 298, 417–426.
) we found MSI containing phlogopite with barite (BaSO4; Figs. 2a, S-1) or magnesite (MgCO3), and phosphate minerals (apatite and monazite; Fig. 2b), as well as various multi-silicate inclusions. Single mineral inclusions of pentlandite (Fig. 2c) and graphite (Fig. 2d) also occur. Because of the similarity in mineralogy to that previously reported, this new-found metasomatic assemblage is considered to be equivalent to the M3-3 stage of Scambelluri et al. (2008)Scambelluri, M., Pettke, T., van Roermund, H.L.M. (2008) Majoritic garnets monitor deep subduction fluid flow and mantle dynamics. Geology 36, 59–62.
.
Figure 1 Example of studied metasomatised harzburgite from the Bardane peridotite. (a) Scanned thin section of sample BDN03 showing metasomatic garnet (Grt), orthopyroxene (Opx), phlogopite (Phl) and clinopyroxene (Cpx). (b) Photomicrograph showing exsolved needles of Cpx and Opx in the garnet crystal, testifying to a majoritic precursor.

Figure 2 Multi-phase solid inclusions in BDN03 garnet. Back scattered electron (BSE) images showing: (a) MSI containing phlogopite and barite; (b) MSI containing magnesite, phlogopite, apatite and monazite; (c) pentlandite inclusions in garnet; (d) garnet-enclosed graphite.
The Ugelvik peridotite records stages M1 and M2 but has not been affected by later metasomatism (Brueckner et al., 2002
Brueckner, K.H., Carswell, D.A., Griffin, W.L. (2002) Paleozoic diamonds within a Precambrian peridotite lens in UHP gneisses of the Norwegian Caledonides. Earth and Planetary Science Letters 203, 805–816.
; Spengler et al., 2006Spengler, D., van Roermund, H.L., Drury, M.R., Ottolini, L., Mason, P.R., Davies, G.R. (2006) Deep origin and hot melting of an Archaean orogenic peridotite massif in Norway. Nature 440, 913–17.
). Compositional mapping of UGL01 garnet, a garnet megacryst from harzburgitic domain in the Ugelvik peridotite, shows an absence of hydrous minerals or MSI (Fig. 3a). An excess of Si indicates a majoritic component in UGL01 garnet (Table S-1), implying an equilibration pressure of ~7.6 GPa (Supplementary Information).
Figure 3 Comparison between metasomatic garnet from Bardane (BDN03) and garnet from Ugelvik (UGL01). (a) Compositional map of sample UGL01 showing variations in the concentration of K (red; not detected), Ba (green; not detected) and Mn (blue; 0 to 0.46 wt. % ± 133 ppm), indicating a lack of metasomatic minerals. Green spots represent the sites chosen for the acquisition of XANES spectra. (b) Results of XANES analysis comparing the Fe3+/Fetot ratio in primary garnet in UGL01 with metasomatic garnet in BDN03. Error bar is shown in the lower right-hand corner. (c) Compositional map of sample BDN03 showing variations in the concentration of K (red; 0 to 14.1 wt. % ± 0.7 wt. %), Ba (green; 0 to 11.6 wt. % ± 0.3 wt. %) and Mn (blue; 0 to 1.0 wt. % ± 0.1 wt. %). Yellow dots are locations where XANES spectra were acquired. Phlogopite is highlighted by elevated K (red), and barite-rich inclusions by elevated Ba (green). The yellow–orange tinted areas highlight the coexistence of barite and phlogopite.
To investigate whether slab-derived fluids promoted oxidation of depleted mantle through metasomatism at Bardane we measured Fe3+/Fetot in garnet from Bardane and Ugelvik using XANES spectroscopy (Supplementary Information). Our results show that the metasomatic garnet from Bardane has higher Fe3+/Fetot compared to the primary garnet from Ugelvik, despite having formed at lower pressure (Fig. 3b; Table S-1; cf. Luth et al., 1990
Luth, R.W., Virgo, D., Boyd, F.R., Wood, B.J. (1990) Ferric iron in mantle-derived garnets. Contributions to Mineralogy and Petrology 104, 56–72.
). Fe3+/Fetot is a proxy for oxidation state such that an increase in Fe3+/Fetot indicates more oxidising conditions (Berry et al., 2010Berry, A.J., Yaxley, G.M., Woodland, A.B., Foran, G.J. (2010) A XANES calibration for determining the oxidation state of iron in mantle garnet. Chemical Geology 278, 31–37.
). Oxygen fugacity (ƒO2) for UGL01 (7.6 GPa; 1572 ˚C), calculated using the Miller et al. (2016)Miller, W.G.R., Holland, T.J.B., Gibson, S.A. (2016) Garnet and Spinel Oxybarometers: New Internally Consistent Multi-equilibria Models with Applications to the Oxidation State of the Lithospheric Mantle. Journal of Petrology 57, 1199–1222.
calibration, ranges from Δlog FMQ -6.36 to -4.75, and for BDN03 (6.3 GPa; 1000 ˚C) from Δlog FMQ -3.47 to -3.16 (Fig. 4; Table S-1; Supplementary Information). It should be noted here that mantle ƒO2 is not buffered by FMQ, but varies relative to FMQ as a function of pressure via Fe2+–Fe3+ exchange between olivine, orthopyroxene and garnet (e.g., Miller et al., 2016Miller, W.G.R., Holland, T.J.B., Gibson, S.A. (2016) Garnet and Spinel Oxybarometers: New Internally Consistent Multi-equilibria Models with Applications to the Oxidation State of the Lithospheric Mantle. Journal of Petrology 57, 1199–1222.
). Figure 4 shows that the metasomatised sample from Bardane, BDN03, has a higher ƒO2 compared to a model depleted mantle (Supplementary Information). This relative oxidation implies that metasomatic fluids introduced oxidised species into the harzburgite (cf. Van Roermund et al., 2002Van Roermund, H.L.M., Carswell, D.A., Drury, M.R., Heijboer, T.C. (2002) Microdiamonds in megacrystic garnet websterite pod from Bardane on the island of Fjortft, western Norway: Evidence for diamond formation in mantle rocks during deep continental subduction. Geology 30, 959–962.
; Scambelluri et al., 2008Scambelluri, M., Pettke, T., van Roermund, H.L.M. (2008) Majoritic garnets monitor deep subduction fluid flow and mantle dynamics. Geology 36, 59–62.
).
Figure 4 Oxygen fugacity of orogenic peridotites. Variation in ƒO2 with depth is reported for a model depleted mantle (green line) and the stability of carbonate vs. graphite/diamond is shown by the EMOD/G (blue line). Dashed line is a regression line at pressures where it was not possible to directly calculate ƒO2. ƒO2 for UGL01 is represented by green dots and the green bold line represents the variation range. BDN03 ƒO2 is reported in yellow while in orange is the ƒO2 of metasomatised orogenic peridotites from Malaspina et al. (2009)
Malaspina, N., Poli, S., Fumagalli, P. (2009) The Oxidation State of Metasomatized Mantle Wedge: Insights from C-O-H-bearing Garnet Peridotite. Journal of Petrology 50, 1533–1552.
and Malaspina et al. (2010)Malaspina, N., Scambelluri, M., Poli, S., Van Roermund, H.L.M., Langenhorst, F. (2010) The oxidation state of mantle wedge majoritic garnet websterites metasomatised by C-bearing subduction fluids. Earth and Planetary Science Letters 298, 417–426.
. Literature data have been recalculated using the Miller et al. (2016)Miller, W.G.R., Holland, T.J.B., Gibson, S.A. (2016) Garnet and Spinel Oxybarometers: New Internally Consistent Multi-equilibria Models with Applications to the Oxidation State of the Lithospheric Mantle. Journal of Petrology 57, 1199–1222.
oxybarometer. An error bar for the ƒO2 is reported in the lower right-hand side of the diagram.Since the MSI are considered to have precipitated from fluids that permeated the peridotite (Van Roermund et al., 2002
Van Roermund, H.L.M., Carswell, D.A., Drury, M.R., Heijboer, T.C. (2002) Microdiamonds in megacrystic garnet websterite pod from Bardane on the island of Fjortft, western Norway: Evidence for diamond formation in mantle rocks during deep continental subduction. Geology 30, 959–962.
; Scambelluri et al., 2008Scambelluri, M., Pettke, T., van Roermund, H.L.M. (2008) Majoritic garnets monitor deep subduction fluid flow and mantle dynamics. Geology 36, 59–62.
), the presence of carbonate and sulphate imply the introduction of oxidised sulphur and carbon during metasomatism. Furthermore, the presence of minerals with reduced sulphur and carbon enclosed in the garnet require that some of the oxidised sulphur and carbon has been reduced to S2- and C0. At present, there are no experimental data on sulphur speciation at the pressure and temperature recorded by the Bardane peridotite; however, oxidation can be achieved by reduction of any oxidised sulphur species (e.g., SO42-, HSO4- or a polysulphide such as S3-) because all require addition of electrons to be reduced to S2- to form sulphide, as shown by Reaction 1 in the case of sulphate, and Reaction 2 for S3-. Carbon in aqueous fluids at elevated ƒO2 and neutral pH is predicted to be predominantly present as CO2 (Sverjensky et al., 2014Sverjensky, D.A., Stagno, V., Huang, F. (2014) Important role for organic carbon in subduction-zone fluids in the deep carbon cycle. Nature Geoscience 7, 909–913.
) and its reduction to form diamond/graphite is illustrated in Reaction 3. Reduction of oxidised sulphur and carbon to sulphide and graphite require the addition of electrons (Reactions 1, 2 and 3), which must come from other multivalent elements in harzburgite minerals, most likely iron. Metasomatism involving these redox equilibria results in net oxidation of the silicate assemblage by conversion of ferrous iron to ferric iron in newly formed metasomatic minerals such as garnet (Reaction 4).Reaction 1
S6+ + 8e- = S2- (sulphide/fluid)
Reaction 2
S3- + 5e- = 3S2-(sulphide/fluid)
Reaction 3
C4+ + 4e- = C0(diamond)
Reaction 4
Fe2+(olivine/orthopyroxene) = Fe3+(garnet) + 1e-
The role of carbon as an oxidant at subduction zones has previously been disregarded because the ƒO2 of the sub-arc mantle was considered to be above the graphite–carbonate buffer; however, the ƒO2 of the sub-arc mantle has been inferred from spinel-bearing xenoliths (cf. Evans and Tomkins, 2011
Evans, K.A., Tomkins, A.G. (2011) The relationship between subduction zone redox budget and arc magma fertility. Earth and Planetary Science Letters 308, 401–409.
) sampling only the shallower portion of the wedge. Geophysical investigations have shown that the depth of the slab–mantle interface below volcanic arcs and the depth of melting lies largely in the garnet stability field (e.g., Syracuse and Abers, 2006Syracuse, E.M., Abers, G.A. (2006) Global compilation of variations in slab depth beneath arc volcanoes and implications. Geochemistry, Geophysics, Geosystems 7, 1–18.
). Accordingly, the portion of mantle wedge that can be directly oxidised by slab fluids, i.e. between the slab and the locus of melting, is mainly confined to the garnet stability field. Figure 4 shows that the ƒO2 of depleted garnet-bearing mantle is within the stability field of elemental carbon, delineated by the EMOD/G line (Luth, 1993Luth, R.W. (1993) Diamonds, Eclogites, and the oxidation state of the Earth's mantle. Science 261, 66–68.
) for the entire range of depths relevant to direct alteration by slab fluids (Supplementary Information). This implies that CO2 introduced by slab fluids in the deeper mantle wedge will be reduced to graphite/diamond during fluid-mantle interaction (Reaction 3), promoting oxidation of ferrous iron (Reaction 4).Further oxidation can be achieved by reduction of oxidised sulphur, which can increase ƒO2 above the EMOD/G curve up to the sulphide–sulphate oxygen buffer (~FMQ +2; Mungall, 2002
Mungall, E.J. (2002) Roasting the mantle: Slab melting and the genesis of major Au and Au-rich Cu deposits. Geology 30, 915–918.
; Jugo et al., 2010Jugo, P.J., Wilke, M., Botcharnikov, R.E. (2010) Sulfur K-edge XANES analysis of natural and synthetic basaltic glasses: Implications for S speciation and S content as function of oxygen fugacity. Geochimica et Cosmochimica Acta 74, 5926–5938.
). BDN03 and other natural samples from the mantle wedge that have been metasomatised by slab fluids display ƒO2 values higher than the depleted mantle curve estimated here (Malaspina et al. 2009Malaspina, N., Poli, S., Fumagalli, P. (2009) The Oxidation State of Metasomatized Mantle Wedge: Insights from C-O-H-bearing Garnet Peridotite. Journal of Petrology 50, 1533–1552.
, 2010Malaspina, N., Scambelluri, M., Poli, S., Van Roermund, H.L.M., Langenhorst, F. (2010) The oxidation state of mantle wedge majoritic garnet websterites metasomatised by C-bearing subduction fluids. Earth and Planetary Science Letters 298, 417–426.
) (Fig. 4). The majority of these samples lie in the elemental carbon field, meaning that, contrary to previous suggestions (e.g., Evans and Tomkins, 2011Evans, K.A., Tomkins, A.G. (2011) The relationship between subduction zone redox budget and arc magma fertility. Earth and Planetary Science Letters 308, 401–409.
), oxidation of the mantle wedge can be achieved by C4+ reduction alone. However, some samples plot on the right side of the EMOD/G curve, implying that sulphur reduction must have taken place in these cases.The clear difference in barite content between UGL01 and BDN03 (cf. Figs. S-1, S-2) indicates that the oxidising fluid was also barium-rich. This observation is consistent with the positive correlation between Ba enrichment and oxidation state seen in arc basalts (e.g., Brounce et al., 2014
Brounce, M.N., Kelley, K.A., Cottrell, E. (2014) Variations in Fe3+/ Fe of Mariana Arc basalts and mantle wedge ƒO2. Journal of Petrology 55, 2513–2536.
), and combined, these observations hint that barite precipitation may have been tied to the oxidation process. Although barite stability has not been investigated experimentally at high pressure and temperature, Ba2+ is immobile in the presence of SO42- at upper crustal conditions, whereas modestly oxidised conditions allow its mobility (more reduced than 1 log unit ƒO2 below the pyrite–haematite and haematite–magnetite buffers; Hanor, 2000Hanor, J.S. (2000) Barite-Celestine Geochemistry and Environments of Formation. Reviews in Mineralogy and Geochemistry 40, 193–275.
). In the sub-arc mantle, the polysulphide ion, S3-, may be dominant at moderate ƒO2 conditions (Pokrovski and Dubessy, 2015Pokrovski, G.S., Dubessy, J. (2015) Stability and abundance of the trisulfur radical ion S3- in hydrothermal fluids. Earth and Planetary Science Letters 411, 298–309.
); the lack of sulphate in solution would allow Ba mobility in typical slab fluids. We suggest that equilibration between S3- and the mantle during metasomatism would allow introduction of Ba via moderately oxidised fluid and formation of both sulphides and barite whilst oxidising Fe2+ to Fe3+ in the silicate assemblage, as illustrated by Reaction 5:Reaction 5
Ca3Al2Si3O12 | + | 7Fe22+SiO4 | + 3MgSiO3 + | 2S3-+Ba2+ | = | Ca3Fe23+Si3O12 | + | Mg3Al2Si3O12 | + 7FeSiO3 + | 5FeS | + | BaSO4 | ||||
garnet | olivine | orthopyroxene | fluid | = | garnet | garnet | orthopyroxene | sulphide | barite |
Luth, R.W., Virgo, D., Boyd, F.R., Wood, B.J. (1990) Ferric iron in mantle-derived garnets. Contributions to Mineralogy and Petrology 104, 56–72.
, which controls the oxygen fugacity of the garnet-bearing mantle. A comparison between the oxygen fugacity of the Bardane metasomatic assemblage and that of depleted mantle (Fig. 4) shows that Reaction 5 is a feasible mechanism for oxidising the sub-arc mantle. Given that sulphur and carbon are the major redox-sensitive elements in aqueous slab fluids (Evans, 2012Evans, K.A. (2012) The redox budget of subduction zones. Earth-Science Reviews 113, 11–32.
), the extent of oxidation achievable by mantle metasomatism is likely to be limited to the sulphide–sulphate transition.Oxidation of the lower mantle wedge by CO2 reduction is supported by the C isotope signature of cratonic diamonds, many of which formed during the Archean (Smart et al., 2016
Smart, K.A., Tappe, S., Stern, R.A., Webb, S.J., Ashwal, L.D. (2016) Early Archaean tectonics and mantle redox recorded in Witwatersrand diamonds. Nature Geoscience 9, 255–259.
), implying that this oxidative process has been ongoing for billions of years. In contrast, S-driven oxidation may have become predominant during the Phanerozoic (Evans and Tomkins, 2011Evans, K.A., Tomkins, A.G. (2011) The relationship between subduction zone redox budget and arc magma fertility. Earth and Planetary Science Letters 308, 401–409.
). Therefore, C may have been more important for long-term redox cycling through arcs, although limiting the magnitude of Precambrian mantle oxidation.Formation of sulphide through reduction of oxidised sulphur provides a sink for chalcophile elements such as Cu and Au, which can be carried in slab fluids. Prolonged metasomatism of the sub-arc mantle by slab fluids would thus form hydrated, oxidised and metal-enriched domains. Because sulphur is an order of magnitude more soluble in oxidised basaltic magma (Jugo et al., 2010
Jugo, P.J., Wilke, M., Botcharnikov, R.E. (2010) Sulfur K-edge XANES analysis of natural and synthetic basaltic glasses: Implications for S speciation and S content as function of oxygen fugacity. Geochimica et Cosmochimica Acta 74, 5926–5938.
) melting of these domains generates H2O-rich and oxidised magma enriched in sulphur and metals. These are characteristics necessary for formation of magmatic-hydrothermal ore deposits at arcs, such as porphyry Cu–Au (Mungall, 2002Mungall, E.J. (2002) Roasting the mantle: Slab melting and the genesis of major Au and Au-rich Cu deposits. Geology 30, 915–918.
).top
Acknowledgements
A. Berry is thanked for provision of the garnet standard for the XANES modelling. The authors acknowledge use of facilities within the Monash Centre for Electron Microscopy. Part of this research was undertaken on the X-ray Fluorescence Microscopy beamline at the Australian Synchrotron, Victoria, Australia. K. Evans and E. Cottrell are thanked for their constructive comments that helped to improve the manuscript. This research was funded by a Monash Research Accelerator Grant to A. Tomkins and by a Society of Economic Geologists (SEG) student research grant to A. Rielli. O. Nebel was supported through an ARC DECRA fellowship (DE120100513).
Editor: Graham Pearson
top
References
Ballhaus, C. (1993) Redox states of lithospheric and asthenospheric upper mantle. Contributions to Mineralogy and Petrology 114, 331–348.

Fluids liberated during dehydration of subducted crust trigger partial melting of the overlying mantle leading to the formation of volcanic arcs, dominated by oxidised rocks (Ballhaus, 1993; Kelley and Cottrell, 2009).
View in article
Berry, A.J., Yaxley, G.M., Woodland, A.B., Foran, G.J. (2010) A XANES calibration for determining the oxidation state of iron in mantle garnet. Chemical Geology 278, 31–37.

Fe3+/Fetot is a proxy for oxidation state such that an increase in Fe3+/Fetot indicates more oxidising conditions (Berry et al., 2010).
View in article
Brounce, M.N., Kelley, K.A., Cottrell, E. (2014) Variations in Fe3+/ Fe of Mariana Arc basalts and mantle wedge ƒO2. Journal of Petrology 55, 2513–2536.

Correlation between oxidation of arc lavas and addition of slab material by aqueous fluids to their mantle source has been quantitatively established (Kelley and Cottrell, 2009; Brounce et al., 2014), indicating that slab fluids are somehow responsible for the observed oxidation.
View in article
This observation is consistent with the positive correlation between Ba enrichment and oxidation state seen in arc basalts (e.g., Brounce et al., 2014), and combined, these observations hint that barite precipitation may have been tied to the oxidation process. View in article
Brueckner, K.H., Carswell, D.A., Griffin, W.L. (2002) Paleozoic diamonds within a Precambrian peridotite lens in UHP gneisses of the Norwegian Caledonides. Earth and Planetary Science Letters 203, 805–816.

Subsequently, during the Caledonian orogeny, fluids derived from subducting slab locally promoted metasomatism (Stage M3), as recorded by the Bardane peridotite on Fjørtoft island (Brueckner et al., 2002; Van Roermund et al., 2002; Scambelluri et al., 2008).
View in article
Other portions have not been infiltrated by slab fluids and preserve the original mantle assemblage (M1-M2), exemplified by the Ugelvik peridotite on the island of Otrøy (Brueckner et al., 2002; Spengler et al., 2006).
View in article
Although there is debate on the origin of metasomatism at Bardane, the trace element and isotopic signature of the metasomatic assemblage are comparable to the effects of typical subduction zone fluids sensu lato (cf. Brueckner et al., 2002; Scambelluri et al., 2008).
View in article
Previous studies at Bardane focused on a megacrystic garnet–websterite lens (e.g., Brueckner et al., 2002) and showed that interaction between slab fluids and depleted mantle generated metasomatic orthopyroxene, clinopyroxene, spinel, diamond (Van Roermund et al., 2002) and majoritic garnet, which indicate peak conditions of ~6.5 GPa and 800–1000 °C (M3-3 stage; Scambelluri et al., 2008).
View in article
The Ugelvik peridotite records stages M1 and M2 but has not been affected by later metasomatism (Brueckner et al., 2002; Spengler et al., 2006).
View in article
Evans, K.A. (2012) The redox budget of subduction zones. Earth-Science Reviews 113, 11–32.

Plate tectonics drives recycling of surface material into the Earth’s interior, introducing hydrated and oxidised oceanic lithosphere into the mantle at subduction zones (Wood et al., 1990; Evans, 2012).
View in article
Given that sulphur and carbon are the major redox-sensitive elements in aqueous slab fluids (Evans, 2012), the extent of oxidation achievable by mantle metasomatism is likely to be limited to the sulphide–sulphate transition.
View in article
Evans, K.A., Tomkins, A.G. (2011) The relationship between subduction zone redox budget and arc magma fertility. Earth and Planetary Science Letters 308, 401–409.

However, although calculations suggest that fluid-borne oxidised sulphur may be important (Evans and Tomkins, 2011), the process has not been resolved with physical evidence.
View in article
The role of carbon as an oxidant at subduction zones has previously been disregarded because the ƒO2 of the sub-arc mantle was considered to be above the graphite–carbonate buffer; however, the ƒO2 of the sub-arc mantle has been inferred from spinel-bearing xenoliths (cf. Evans and Tomkins, 2011) sampling only the shallower portion of the wedge.
View in article
The majority of these samples lie in the elemental carbon field, meaning that, contrary to previous suggestions (e.g., Evans and Tomkins, 2011), oxidation of the mantle wedge can be achieved by C4+ reduction alone.
View in article
In contrast, S-driven oxidation may have become predominant during the Phanerozoic (Evans and Tomkins, 2011).
View in article
Hanor, J.S. (2000) Barite-Celestine Geochemistry and Environments of Formation. Reviews in Mineralogy and Geochemistry 40, 193–275.

Although barite stability has not been investigated experimentally at high pressure and temperature, Ba2+ is immobile in the presence of SO42- at upper crustal conditions, whereas modestly oxidised conditions allow its mobility (more reduced than 1 log unit ƒO2 below the pyrite–haematite and haematite–magnetite buffers; Hanor, 2000).
View in article
Jugo, P.J., Wilke, M., Botcharnikov, R.E. (2010) Sulfur K-edge XANES analysis of natural and synthetic basaltic glasses: Implications for S speciation and S content as function of oxygen fugacity. Geochimica et Cosmochimica Acta 74, 5926–5938.

Further oxidation can be achieved by reduction of oxidised sulphur, which can increase ƒO2 above the EMOD/G curve up to the sulphide–sulphate oxygen buffer (~FMQ +2; Mungall, 2002; Jugo et al., 2010).
View in article
Because sulphur is an order of magnitude more soluble in oxidised basaltic magma (Jugo et al., 2010) melting of these domains generates H2O-rich and oxidised magma enriched in sulphur and metals.
View in article
Kelley, K.A., Cottrell, E. (2009) Water and oxidation state of subduction zone magmas. Science 325, 605–607.

Fluids liberated during dehydration of subducted crust trigger partial melting of the overlying mantle leading to the formation of volcanic arcs, dominated by oxidised rocks (Ballhaus, 1993; Kelley and Cottrell, 2009).
View in article
Correlation between oxidation of arc lavas and addition of slab material by aqueous fluids to their mantle source has been quantitatively established (Kelley and Cottrell, 2009; Brounce et al., 2014), indicating that slab fluids are somehow responsible for the observed oxidation.
View in article
Luth, R.W. (1993) Diamonds, Eclogites, and the oxidation state of the Earth's mantle. Science 261, 66–68.

Figure 4 shows that the ƒO2 of depleted garnet-bearing mantle is within the stability field of elemental carbon, delineated by the EMOD/G line (Luth, 1993) for the entire range of depths relevant to direct alteration by slab fluids (Supplementary Information).
View in article
Luth, R.W., Virgo, D., Boyd, F.R., Wood, B.J. (1990) Ferric iron in mantle-derived garnets. Contributions to Mineralogy and Petrology 104, 56–72.

Our results show that the metasomatic garnet from Bardane has higher Fe3+/Fetot compared to the primary garnet from Ugelvik, despite having formed at lower pressure (Fig. 3b; Table S-1; cf. Luth et al., 1990).
View in article
In this redox equilibrium, Reaction 2 is incorporated to modify the garnet-olivine-orthopyroxene ƒO2 buffer defined by Luth et al. (1990), which controls the oxygen fugacity of the garnet-bearing mantle.
View in article
Malaspina, N., Poli, S., Fumagalli, P. (2009) The Oxidation State of Metasomatized Mantle Wedge: Insights from C-O-H-bearing Garnet Peridotite. Journal of Petrology 50, 1533–1552.

Figure 4 [...] BDN03 ƒO2 is reported in yellow while in orange is the ƒO2 of metasomatised orogenic peridotites from Malaspina et al. (2009) and Malaspina et al. (2010).
View in article
BDN03 and other natural samples from the mantle wedge that have been metasomatised by slab fluids display ƒO2 values higher than the depleted mantle curve estimated here (Malaspina et al., 2009, 2010) (Fig. 4).
View in article
Malaspina, N., Scambelluri, M., Poli, S., Van Roermund, H.L.M., Langenhorst, F. (2010) The oxidation state of mantle wedge majoritic garnet websterites metasomatised by C-bearing subduction fluids. Earth and Planetary Science Letters 298, 417–426.

Multi-phase solid inclusions (MSI) are hosted in M3 minerals and are thought to have crystallised from slab fluids that permeated the peridotite and contain phlogopite, spinel, amphibole, dolomite, monazite, magnesite, graphite and diamond (Van Roermund et al., 2002; Malaspina et al., 2010).
View in article
In addition to the previously reported mineralogy (Van Roermund et al., 2002; Malaspina et al., 2010) we found MSI containing phlogopite with barite (BaSO4; Figs. 2a, S-1) or magnesite (MgCO3), and phosphate minerals (apatite and monazite; Fig. 2b), as well as various multi-silicate inclusions.
View in article
Figure 4 [...] BDN03 ƒO2 is reported in yellow while in orange is the ƒO2 of metasomatised orogenic peridotites from Malaspina et al. (2009) and Malaspina et al. (2010).
View in article
BDN03 and other natural samples from the mantle wedge that have been metasomatised by slab fluids display ƒO2 values higher than the depleted mantle curve estimated here (Malaspina et al., 2009, 2010) (Fig. 4).
View in article
Miller, W.G.R., Holland, T.J.B., Gibson, S.A. (2016) Garnet and Spinel Oxybarometers: New Internally Consistent Multi-equilibria Models with Applications to the Oxidation State of the Lithospheric Mantle. Journal of Petrology 57, 1199–1222.

Oxygen fugacity (ƒO2) for UGL01 (7.6 GPa; 1572 ˚C), calculated using the Miller et al. (2016) calibration, ranges from Δlog FMQ -6.36 to -4.75, and for BDN03 (6.3 GPa; 1000 ˚C) from Δlog FMQ -3.47 to -3.16 (Fig. 4; Table S-1; Supplementary Information).
View in article
It should be noted here that mantle ƒO2 is not buffered by FMQ, but varies relative to FMQ as a function of pressure via Fe2+–Fe3+ exchange between olivine, orthopyroxene and garnet (e.g., Miller et al., 2016).
View in article
Figure 4 [...] Literature data have been recalculated using the Miller et al. (2016) oxybarometer. An error bar for the ƒO2 is reported in the lower right-hand side of the diagram.
View in article
Mungall, E.J. (2002) Roasting the mantle: Slab melting and the genesis of major Au and Au-rich Cu deposits. Geology 30, 915–918.

Further oxidation can be achieved by reduction of oxidised sulphur, which can increase ƒO2 above the EMOD/G curve up to the sulphide–sulphate oxygen buffer (~FMQ +2; Mungall, 2002; Jugo et al., 2010).
View in article
These are characteristics necessary for formation of magmatic-hydrothermal ore deposits at arcs, such as porphyry Cu–Au (Mungall, 2002).
View in article
Pokrovski, G.S., Dubessy, J. (2015) Stability and abundance of the trisulfur radical ion S3- in hydrothermal fluids. Earth and Planetary Science Letters 411, 298–309.

In the sub-arc mantle, the polysulphide ion, S3-, may be dominant at moderate ƒO2 conditions (Pokrovski and Dubessy, 2015); the lack of sulphate in solution would allow Ba mobility in typical slab fluids.
View in article
Scambelluri, M., Pettke, T., van Roermund, H.L.M. (2008) Majoritic garnets monitor deep subduction fluid flow and mantle dynamics. Geology 36, 59–62.

Subsequently, during the Caledonian orogeny, fluids derived from subducting slab locally promoted metasomatism (Stage M3), as recorded by the Bardane peridotite on Fjørtoft island (Brueckner et al., 2002; Van Roermund et al., 2002; Scambelluri et al., 2008).
View in article
Although there is debate on the origin of metasomatism at Bardane, the trace element and isotopic signature of the metasomatic assemblage are comparable to the effects of typical subduction zone fluids sensu lato (cf. Brueckner et al., 2002; Scambelluri et al., 2008).
View in article
Previous studies at Bardane focused on a megacrystic garnet–websterite lens (e.g., Brueckner et al., 2002) and showed that interaction between slab fluids and depleted mantle generated metasomatic orthopyroxene, clinopyroxene, spinel, diamond (Van Roermund et al., 2002) and majoritic garnet, which indicate peak conditions of ~6.5 GPa and 800–1000 °C (M3-3 stage; Scambelluri et al., 2008).
View in article
The garnet megacrysts contain sparse exsolved clinopyroxene and orthopyroxene needles (Fig. 1b) with similar size, abundance and distribution to the exsolution previously reported for M3 garnet (Scambelluri et al., 2008).
View in article
Because of the similarity in mineralogy to that previously reported, this new-found metasomatic assemblage is considered to be equivalent to the M3-3 stage of Scambelluri et al. (2008).
View in article
This relative oxidation implies that metasomatic fluids introduced oxidised species into the harzburgite (cf. Van Roermund et al., 2002; Scambelluri et al., 2008).
View in article
Since the MSI are considered to have precipitated from fluids that permeated the peridotite (Van Roermund et al., 2002; Scambelluri et al., 2008), the presence of carbonate and sulphate imply the introduction of oxidised sulphur and carbon during metasomatism.
View in article
Smart, K.A., Tappe, S., Stern, R.A., Webb, S.J., Ashwal, L.D. (2016) Early Archaean tectonics and mantle redox recorded in Witwatersrand diamonds. Nature Geoscience 9, 255–259.

Oxidation of the lower mantle wedge by CO2 reduction is supported by the C isotope signature of cratonic diamonds, many of which formed during the Archean (Smart et al., 2016), implying that this oxidative process has been ongoing for billions of years.
View in article
Spengler, D., van Roermund, H.L., Drury, M.R., Ottolini, L., Mason, P.R., Davies, G.R. (2006) Deep origin and hot melting of an Archaean orogenic peridotite massif in Norway. Nature 440, 913–17.

The Western Gneiss Region of Norway hosts several orogenic peridotite bodies that represent a portion of transition zone mantle that upwelled, melted (Stage M1) and accreted to cratonic lithosphere (Stage M2; Spengler et al., 2006).
View in article
Other portions have not been infiltrated by slab fluids and preserve the original mantle assemblage (M1-M2), exemplified by the Ugelvik peridotite on the island of Otrøy (Brueckner et al., 2002; Spengler et al., 2006).
View in article
The Ugelvik peridotite records stages M1 and M2 but has not been affected by later metasomatism (Brueckner et al., 2002; Spengler et al., 2006).
View in article
Sverjensky, D.A., Stagno, V., Huang, F. (2014) Important role for organic carbon in subduction-zone fluids in the deep carbon cycle. Nature Geoscience 7, 909–913.

Carbon in aqueous fluids at elevated ƒO2 and neutral pH is predicted to be predominantly present as CO2 (Sverjensky et al., 2014) and its reduction to form diamond/graphite is illustrated in Reaction 3.
View in article
Syracuse, E.M., Abers, G.A. (2006) Global compilation of variations in slab depth beneath arc volcanoes and implications. Geochemistry, Geophysics, Geosystems 7, 1–18.

Geophysical investigations have shown that the depth of the slab–mantle interface below volcanic arcs and the depth of melting lies largely in the garnet stability field (e.g., Syracuse and Abers, 2006).
View in article
Van Roermund, H.L.M., Carswell, D.A., Drury, M.R., Heijboer, T.C. (2002) Microdiamonds in megacrystic garnet websterite pod from Bardane on the island of Fjortft, western Norway: Evidence for diamond formation in mantle rocks during deep continental subduction. Geology 30, 959–962.

Subsequently, during the Caledonian orogeny, fluids derived from subducting slab locally promoted metasomatism (Stage M3), as recorded by the Bardane peridotite on Fjørtoft island (Brueckner et al., 2002; Van Roermund et al., 2002; Scambelluri et al., 2008).
View in article
Previous studies at Bardane focused on a megacrystic garnet–websterite lens (e.g., Brueckner et al., 2002) and showed that interaction between slab fluids and depleted mantle generated metasomatic orthopyroxene, clinopyroxene, spinel, diamond (Van Roermund et al., 2002) and majoritic garnet, which indicate peak conditions of ~6.5 GPa and 800–1000 °C (M3-3 stage; Scambelluri et al., 2008).
View in article
Multi-phase solid inclusions (MSI) are hosted in M3 minerals and are thought to have crystallised from slab fluids that permeated the peridotite and contain phlogopite, spinel, amphibole, dolomite, monazite, magnesite, graphite and diamond (Van Roermund et al., 2002; Malaspina et al., 2010).
View in article
In addition to the previously reported mineralogy (Van Roermund et al., 2002; Malaspina et al., 2010) we found MSI containing phlogopite with barite (BaSO4; Figs. 2a, S-1) or magnesite (MgCO3), and phosphate minerals (apatite and monazite; Fig. 2b), as well as various multi-silicate inclusions.
View in article
This relative oxidation implies that metasomatic fluids introduced oxidised species into the harzburgite (cf. Van Roermund et al., 2002; Scambelluri et al., 2008).
View in article
Since the MSI are considered to have precipitated from fluids that permeated the peridotite (Van Roermund et al., 2002; Scambelluri et al., 2008), the presence of carbonate and sulphate imply the introduction of oxidised sulphur and carbon during metasomatism.
View in article
Wood, B.J., Bryndzia, L.T., Johnson, E.K. (1990) Mantle oxidation state and its relationship to tectonic environment and fluid speciation. Science 248, 337–345.

Plate tectonics drives recycling of surface material into the Earth’s interior, introducing hydrated and oxidised oceanic lithosphere into the mantle at subduction zones (Wood et al., 1990; Evans, 2012).
View in article
top
Supplementary Information
Pressure and Temperature Estimation for UGL01 and BDN03
Garnet megacryst in sample UGL01 has Si cation content larger than 3.00 for a total of 12 oxygen indicating a majoritic composition (Table S-1). Pressure for this sample was calculated using the majorite geobarometer calibrated by Wijbrans et al. (2016). The value considered for the calculation is the one from the core (Grt core_2 in Table S-1). The calculation gives pressure of 7.59 GPa. Temperature has been estimated using a lithospheric geotherm of 50.4 mW m-1 and corresponds to 1572 ˚C.
Pressure for BDN03 has been calculated using the geobarometer of Wijbrans et al. (2016) from the reconstructed majoritic compositon of M3-3 garnet from Scambelluri et al. (2008) and the estimated pressure is 6.3 GPa. This pressure has been used for the recalculation of ƒO2 for M3-3 assemblage from the Bardane peridotite previously published by Malaspina et al. (2010).
Calculation of Depleted Mantle ƒO2
To draw the depleted mantle line in Figure 4, depleted mantle composition in major oxides from Salters and Stracke (2004) has been used as starting material with 0.1 wt. % of Fe2O3. This Fe2O3 value was chosen considering the dataset presented by Canil et al. (1994), who reported Fe2O3 ranging from 0.1 to 0.4 wt. % in a global suite of mantle xenoliths, therefore the 0.1 wt. % Fe2O3 was considered representative for a depleted mantle end-member. Mineral composition has been calculated with THERMOCALC (Powell and Holland, 1988) using the thermodynamic model from Jennings and Holland (2015). Pressure considered ranges from 3 to 6 GPa (maximum pressure for the thermodynamic model used). Temperature has been extrapolated from a regression line of P-T of the orogenic peridotite samples used in Figure 4. Samples are from Ulten (3 GPa – 850 ˚C), Sulu (4 GPa – 900 ˚C; Malaspina et al., 2009) and Bardane (6 GPa – 1000 ˚C; Malaspina et al., 2010). ƒO2 has been calculated using GtƒO2 software from Miller et al. (2016). Because the depleted mantle ƒO2 line is calculated using the same P-T conditions as the samples considered here, a positive shift of ƒO2 from this line represents the effect of metasomatic oxidation.
To investigate the speciation of carbon in equilibrium with depleted mantle as function of ƒO2, we used the equilibrium between carbonate and graphite/diamond in a mantle assemblage expressed as:
MgSiO3 | + | MgCO3 | = | Mg2SiO4 | + | C + O2 |
enstatite | magnesite | olivine | diamond/graphite |
Imaging
Imaging of the polyphase solid inclusions and mineral identification was carried out using a JEOL 7001F FEG-SEM at the Monash Centre for Electronic Microscopy. Elemental maps of samples UGL01 and BDN03 were acquired at the Australian Synchrotron X-ray Fluorescence Microscopy (XFM) beamline (Paterson et al., 2011). Samples were raster scanned through monochromatic 18.5 keV X-rays focussed to 1.5 x 1.5 µm2. A Maia detector (Paterson et al., 2011; Ryan et al., 2014) collected the X-ray fluorescence and scatter emitted with 1.9 millisec/pixel dwell. Data were processed using GeoPIXE II (Ryan et al., 2014), which incorporates a matrix algorithm that unfolds overlaps by fitting all available X-ray emission lines for each element present in the sample and subtracts background and escape peaks.
XANES Measurement
The Fe3+/Fetot ratio of garnet in UGL01 and BDN03 was determined using Fe K-edge XANES spectra (Fig. S-3) collected using a single element Vortex detector at the XFM beamline (Paterson et al., 2011). The data were normalised using the mback software (Weng et al., 2005), which normalises to tabulated mass absorption coefficients providing a robust scheme that is not sensitive to the shape of the background function. We retrieved the Fe3+/Fetot ratios from an empirical curve generated using the normalised intensity at 7138.4 and 7161.7 eV to Fe3+/Fetot in garnet standards determined by Mössbauer spectroscopy (Fig. S-3; Table S-2) following the method of Berry et al. (2010). The precision of the XANES analyses from standard deviation is 0.0037 and the error for the Mössbauer analysis is 0.01. The overall uncertainty in ƒO2 resulting from the error in the XANES measurement (0.01 in Fe3+/Fetot) and the calibration of the oxybarometer, is estimated to range ± 0.6 log units.
Supplementary Figures and Tables

Figure S-1 Compositional maps showing the distribution of barium (green) and manganese (blue) in garnet BDN03. The manganese distribution highlights the garnet crystal whilst barium indicate the presence of barite hosted in the garnet and the high content of Ba in phlogopite, this latter is visible in the upper-right side of the figure. Ba concentration 0–24 wt. % ± 0.4 wt. %, Mn concentration 0–0.87 wt. % ± 178 ppm.
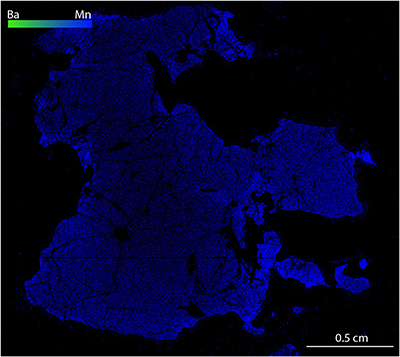
Figure S-2 Compositional maps showing the distribution of barium (green) and manganese (blue) in garnet UGL01. Mn concentration 0–0.59 wt. % ± 240 ppm. Ba has not been detected.

Figure S-3 Fe K-edge XANES spectra for BDN03 and UGL01.
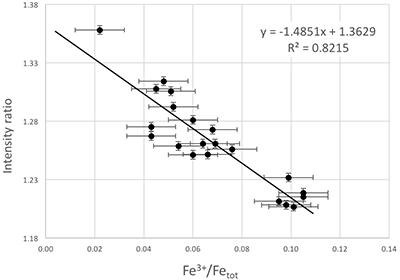
Figure S-4 Calibration line (line of best fit) correlating the intensity ratio of standards at 7138.4 and 7161.7 eV in the XANES spectra with Fe3+/Fetot measured by Mössbauer spectroscopy.
Table S-1 Major and minor element compositions of garnet (Grt), olivine (Ol) and orthopyroxene (Opx) from samples BDN03 and UGL01. The Fe3+/ Fetot of garnet is also reported, together with the pressure and temperature used for the calculation of ƒO2.
BDN03 | UGL01 | ||||||||||||
BDN03_1 | BDN03_2 | BDN03_3 | UGL01_3 | UGL01_1 | UGL01_2 | ||||||||
wt. % | Grt rim | Grt core_1 | Grt core_2 | Ol | Opx | wt. % | Grt rim | Grt core_1 | Grt core_2 | Ol | Opx | ||
SiO2 | 42.06 | 41.78 | 42.16 | 40.96 | 58.25 | SiO2 | 42.18 | 42.83 | 42.78 | 41.52 | 58.52 | ||
TiO2 | 0.05 | 0.05 | 0.04 | 0.00 | 0.03 | TiO2 | 0.01 | 0.03 | 0.00 | 0.01 | 0.01 | ||
Al2O3 | 21.08 | 21.63 | 21.50 | 0.05 | 0.85 | Al2O3 | 19.82 | 20.47 | 20.15 | 0.01 | 0.61 | ||
Cr2O3 | 2.96 | 2.42 | 2.47 | 0.01 | 0.31 | Cr2O3 | 4.16 | 3.63 | 4.06 | 0.01 | 0.25 | ||
Fe2O3 | 0.36 | 0.45 | 0.40 | n.d. | n.d. | Fe2O3 | 0.32 | 0.11 | 0.29 | n.d. | n.d. | ||
FeO | 7.89 | 7.86 | 7.40 | 7.46 | 4.75 | FeO | 8.48 | 6.66 | 6.56 | 7.68 | 4.56 | ||
MnO | 0.54 | 0.41 | 0.36 | 0.14 | 0.11 | MnO | 0.49 | 0.38 | 0.27 | 0.13 | 0.09 | ||
MgO | 20.46 | 20.20 | 20.81 | 50.49 | 35.29 | MgO | 19.57 | 21.56 | 20.67 | 50.49 | 35.73 | ||
CaO | 4.37 | 4.83 | 4.61 | 0.01 | 0.26 | CaO | 5.00 | 4.28 | 4.85 | 0.02 | 0.20 | ||
Na2O | 0.04 | 0.04 | 0.01 | 0.02 | 0.03 | Na2O | 0.02 | 0.00 | 0.04 | 0.01 | 0.03 | ||
NiO | 0.03 | 0.02 | 0.00 | 0.39 | 0.08 | NiO | 0.03 | 0.00 | 0.00 | 0.42 | 0.08 | ||
K2O | 0.00 | 0.02 | 0.00 | 0.01 | 0.01 | K2O | 0.01 | 0.01 | 0.01 | 0.00 | 0.01 | ||
Total | 99.83 | 99.70 | 99.75 | 99.53 | 99.97 | Total | 100.09 | 99.95 | 99.68 | 100.29 | 100.08 | ||
Si | 3.010 | 2.993 | 3.007 | 0.999 | 1.992 | Si | 3.034 | 3.042 | 3.054 | 1.005 | 1.996 | ||
Ti | 0.003 | 0.003 | 0.002 | 0.000 | 0.001 | Ti | 0.000 | 0.002 | 0.000 | 0.000 | 0.000 | ||
Al | 1.778 | 1.826 | 1.808 | 0.002 | 0.034 | Al | 1.681 | 1.714 | 1.695 | 0.000 | 0.025 | ||
Cr | 0.167 | 0.137 | 0.139 | 0.000 | 0.008 | Cr | 0.237 | 0.204 | 0.229 | 0.000 | 0.007 | ||
Fe3+ | 0.019 | 0.024 | 0.021 | 0.000 | 0.000 | Fe3+ | 0.017 | 0.006 | 0.015 | 0.000 | 0.000 | ||
Fe2+ | 0.472 | 0.471 | 0.442 | 0.152 | 0.136 | Fe2+ | 0.510 | 0.395 | 0.392 | 0.155 | 0.130 | ||
Mn | 0.033 | 0.025 | 0.021 | 0.003 | 0.003 | Mn | 0.030 | 0.023 | 0.017 | 0.003 | 0.003 | ||
Mg | 2.183 | 2.157 | 2.213 | 1.836 | 1.799 | Mg | 2.100 | 2.283 | 2.200 | 1.822 | 1.817 | ||
Ca | 0.335 | 0.371 | 0.352 | 0.000 | 0.010 | Ca | 0.385 | 0.326 | 0.371 | 0.001 | 0.007 | ||
Na | 0.006 | 0.006 | 0.001 | 0.001 | 0.002 | Na | 0.002 | 0.000 | 0.006 | 0.000 | 0.002 | ||
Ni | 0.002 | 0.001 | 0.000 | 0.008 | 0.002 | Ni | 0.002 | 0.000 | 0.000 | 0.008 | 0.002 | ||
K | 0.000 | 0.002 | 0.000 | 0.000 | 0.000 | K | 0.001 | 0.001 | 0.000 | 0.000 | 0.000 | ||
Total | 8.008 | 8.015 | 8.007 | 3.001 | 3.987 | Total | 7.999 | 7.995 | 7.979 | 2.995 | 3.989 | ||
P (GPa) | 6.3 | 6.3 | 6.3 | P (GPa) | 7.6 | 7.6 | 7.6 | ||||||
T (˚C) | 1000 | 1000 | 1000 | T (˚C) | 1572 | 1572 | 1572 | ||||||
Fe3+/Fetot | 0.039 | 0.049 | 0.046 | Fe3+/Fetot | 0.033 | 0.014 | 0.038 | ||||||
ƒO2 | -3.47 | -3.16 | -3.21 | ƒO2 | -4.88 | -6.36 | -4.75 |
Table S-2 Results of Fe K-edge XANES spectroscopy analyses. The intensities at 7138.4 eV and 7161.7 eV measured in garnet standards are reported with their ratio and the respective Fe3+/Fetot determined by Mössbauer spectroscopy. Intensities in garnet from samples UGL01 and BDN03 are reported together with their Fe3+/Fetot retrieved using the calibration line in Figure S-4.
Standards | ||||
ID | 7138.4 eV | 7161.7 eV | Intensity ratio | Mössbauer |
Dvk_1 | 0.868 | 1.057 | 1.219 | 0.105 |
Dvk_2 | 0.789 | 1.071 | 1.358 | 0.022 |
Dvk_3 | 0.873 | 1.061 | 1.215 | 0.105 |
Dvk_4 | 0.871 | 1.093 | 1.256 | 0.076 |
Dvk_6 | 0.858 | 1.073 | 1.252 | 0.066 |
Dvk_5 | 0.871 | 1.072 | 1.232 | 0.099 |
Dvk_7 | 0.874 | 1.054 | 1.207 | 0.101 |
Dvk_8 | 0.891 | 1.077 | 1.208 | 0.098 |
Dvk_10 | 0.858 | 1.074 | 1.251 | 0.060 |
LET6-2 | 0.877 | 1.118 | 1.275 | 0.043 |
LET-21 | 0.836 | 1.093 | 1.308 | 0.045 |
LET-7 | 0.838 | 1.102 | 1.314 | 0.048 |
LET-1 | 0.864 | 1.095 | 1.267 | 0.043 |
KIM44 | 0.843 | 1.063 | 1.261 | 0.069 |
KIM35 | 0.862 | 1.105 | 1.281 | 0.060 |
KIM30 | 0.841 | 1.087 | 1.292 | 0.052 |
LET9 | 0.877 | 1.063 | 1.212 | 0.095 |
KIM17 | 0.850 | 1.071 | 1.261 | 0.064 |
Kim39 | 0.869 | 1.135 | 1.306 | 0.051 |
Kim13 | 0.876 | 1.115 | 1.273 | 0.068 |
Kim1 | 0.869 | 1.094 | 1.259 | 0.054 |
Samples | ||||
ID | 7138.4 eV | 7161.7 eV | Intensity ratio | Fe3+/Fetot |
UGL01_1 | 0.83 | 1.09 | 1.314 | 0.033 |
UGL01_2 | 0.85 | 1.11 | 1.306 | 0.038 |
UGL01_3 | 0.82 | 1.10 | 1.341 | 0.014 |
BDN03_1 | 0.82 | 1.07 | 1.305 | 0.039 |
BDN03_2 | 0.86 | 1.11 | 1.291 | 0.049 |
BDN03_3 | 0.84 | 1.09 | 1.295 | 0.046 |
Supplementary Information References
Canil, D., O'Neill, H.S.C., Pearson, D.G., Rudnick, R.L., McDonough, W.F., Carswell, D.A. (1994) Ferric iron in peridotites and mantle oxidation states. Earth and Planetary Science Letters 123, 205–220.
Jennings, E.S., Holland, T.J.B. (2015) A Simple Thermodynamic Model for Melting of Peridotite in the System NCFMASOCr. Journal of Petrology 56, 869–892.
Malaspina, N., Poli, S., Fumagalli, P. (2009) The Oxidation State of Metasomatized Mantle Wedge: Insights from C-O-H-bearing Garnet Peridotite. Journal of Petrology 50, 1533–1552.
Malaspina, N., Scambelluri, M., Poli, S., Van Roermund, H.L.M., Langenhorst, F. (2010) The oxidation state of mantle wedge majoritic garnet websterites metasomatised by C-bearing subduction fluids. Earth and Planetary Science Letters 298, 417–426.
Miller, W.G.R., Holland, T.J.B., Gibson, S.A. (2016) Garnet and Spinel Oxybarometers: New Internally Consistent Multi-equilibria Models with Applications to the Oxidation State of the Lithospheric Mantle. Journal of Petrology 57, 1199–1222.
Paterson, D., de Jonge, M.D., Howard, D.L., Lewis, W., McKinlay, J., Starritt, A., Kusel, M., Ryan, C.G., Kirkham, R., Moorhead, G., Siddons, D.P., McNulty, I., Eyberger, C., Lai, B. (2011) The X-ray Fluorescence Microscopy Beamline at the Australian Synchrotron. AIP Conference Proceedings 1365, 219–222.
Powell, R., Holland, T.J.B. (1988) An internally consistent dataset with uncertainties and correlations: 3. Applications to geobarometry, worked examples and a computer program. Journal of Metamorphic Geology 6, 173–204.
Ryan, C.G., Siddons, D.P., Kirkham, R., Li, Z.Y., de Jonge, M.D., Paterson, D.J., Kuczewski, A., Howard, D.L., Dunn, P.A., Falkenberg, G., Boesenberg, U., De Geronimo, G., Fisher, L.A., Halfpenny, A., Lintern, M.J., Lombi, E., Dyl, K.A., Jensen, M., Moorhead, G.F., Cleverley, J.S., Hough, R.M., Godel, B., Barnes, S.J., James, S.A., Spiers, K.M., Alfeld, M., Wellenreuther, G., Vukmanovic, Z., Borg, S. (2014) Maia X-ray fluorescence imaging: Capturing detail in complex natural samples. Journal of Physics: Conference Series 499, 1–12.
Salters, V.J.M., Stracke, A. (2004) Composition of the depleted mantle. Geochemistry, Geophysics, Geosystems 5, 1–27.
Scambelluri, M., Pettke, T., van Roermund, H.L.M. (2008) Majoritic garnets monitor deep subduction fluid flow and mantle dynamics. Geology 36, 59–62.
Weng, T.C., Waldo, G.S., Penner-Hahn, J.E. (2005) A method for normalization of X-ray absorption spectra. Journal of Synchrotron Radiation 12, 506–510.
Wijbrans, C.H., Rohrbach, A., Klemme, S. (2016) An experimental investigation of the stability of majoritic garnet in the Earth’s mantle and an improved majorite geobarometer. Contributions to Mineralogy and Petrology 171.
Figures and Tables

Figure 1 Example of studied metasomatised harzburgite from the Bardane peridotite. (a) Scanned thin section of sample BDN03 showing metasomatic garnet (Grt), orthopyroxene (Opx), phlogopite (Phl) and clinopyroxene (Cpx). (b) Photomicrograph showing exsolved needles of Cpx and Opx in the garnet crystal, testifying to a majoritic precursor.

Figure 2 Multi-phase solid inclusions in BDN03 garnet. Back scattered electron (BSE) images showing: (a) MSI containing phlogopite and barite; (b) MSI containing magnesite, phlogopite, apatite and monazite; (c) pentlandite inclusions in garnet; (d) garnet-enclosed graphite.

Figure 3 Comparison between metasomatic garnet from Bardane (BDN03) and garnet from Ugelvik (UGL01). (a) Compositional map of sample UGL01 showing variations in the concentration of K (red; not detected), Ba (green; not detected) and Mn (blue; 0 to 0.46 wt. % ± 133 ppm), indicating a lack of metasomatic minerals. Green spots represent the sites chosen for the acquisition of XANES spectra. (b) Results of XANES analysis comparing the Fe3+/Fetot ratio in primary garnet in UGL01 with metasomatic garnet in BDN03. Error bar is shown in the lower right-hand corner. (c) Compositional map of sample BDN03 showing variations in the concentration of K (red; 0 to 14.1 wt. % ± 0.7 wt. %), Ba (green; 0 to 11.6 wt. % ± 0.3 wt. %) and Mn (blue; 0 to 1.0 wt. % ± 0.1 wt. %). Yellow dots are locations where XANES spectra were acquired. Phlogopite is highlighted by elevated K (red), and barite-rich inclusions by elevated Ba (green). The yellow–orange tinted areas highlight the coexistence of barite and phlogopite.

Figure 4 Oxygen fugacity of orogenic peridotites. Variation in ƒO2 with depth is reported for a model depleted mantle (green line) and the stability of carbonate vs. graphite/diamond is shown by the EMOD/G (blue line). Dashed line is a regression line at pressures where it was not possible to directly calculate ƒO2. ƒO2 for UGL01 is represented by green dots and the green bold line represents the variation range. BDN03 ƒO2 is reported in yellow while in orange is the ƒO2 of metasomatised orogenic peridotites from Malaspina et al. (2009)
Malaspina, N., Poli, S., Fumagalli, P. (2009) The Oxidation State of Metasomatized Mantle Wedge: Insights from C-O-H-bearing Garnet Peridotite. Journal of Petrology 50, 1533–1552.
and Malaspina et al. (2010)Malaspina, N., Scambelluri, M., Poli, S., Van Roermund, H.L.M., Langenhorst, F. (2010) The oxidation state of mantle wedge majoritic garnet websterites metasomatised by C-bearing subduction fluids. Earth and Planetary Science Letters 298, 417–426.
. Literature data have been recalculated using the Miller et al. (2016)Miller, W.G.R., Holland, T.J.B., Gibson, S.A. (2016) Garnet and Spinel Oxybarometers: New Internally Consistent Multi-equilibria Models with Applications to the Oxidation State of the Lithospheric Mantle. Journal of Petrology 57, 1199–1222.
oxybarometer. An error bar for the ƒO2 is reported in the lower right-hand side of the diagram.Back to article
Supplementary Figures and Tables
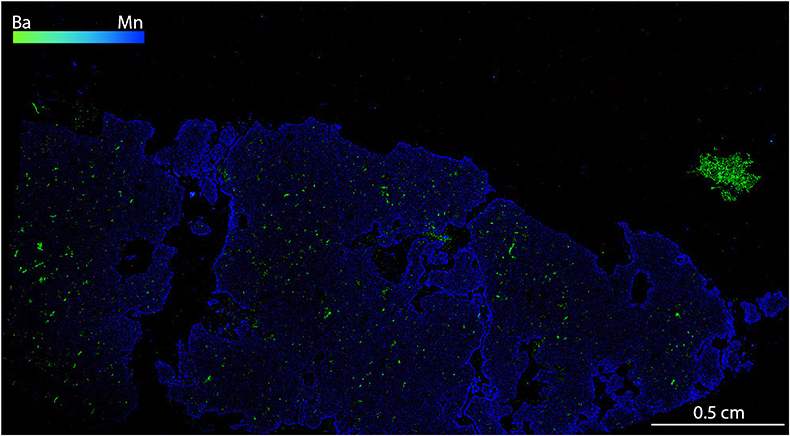
Figure S-1 Compositional maps showing the distribution of barium (green) and manganese (blue) in garnet BDN03. The manganese distribution highlights the garnet crystal whilst barium indicate the presence of barite hosted in the garnet and the high content of Ba in phlogopite, this latter is visible in the upper-right side of the figure. Ba concentration 0–24 wt. % ± 0.4 wt. %, Mn concentration 0–0.87 wt. % ± 178 ppm.

Figure S-2 Compositional maps showing the distribution of barium (green) and manganese (blue) in garnet UGL01. Mn concentration 0–0.59 wt. % ± 240 ppm. Ba has not been detected.
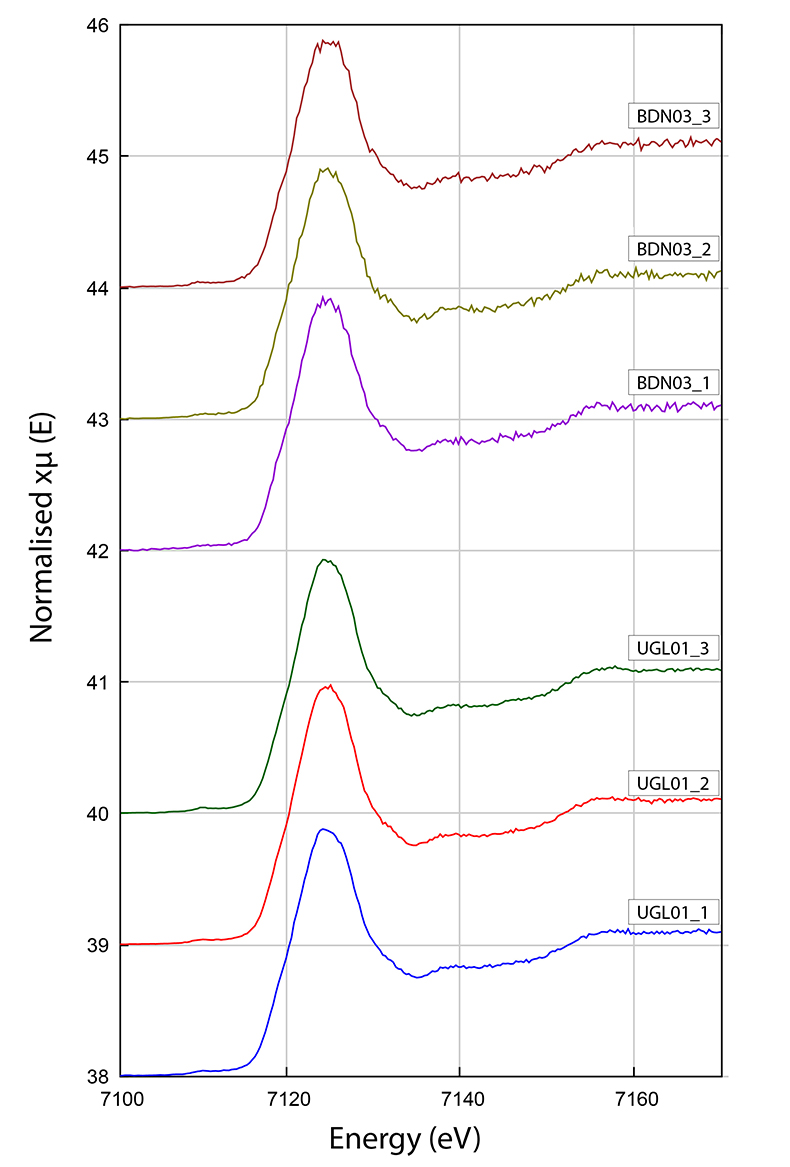
Figure S-3 Fe K-edge XANES spectra for BDN03 and UGL01.

Figure S-4 Calibration line (line of best fit) correlating the intensity ratio of standards at 7138.4 and 7161.7 eV in the XANES spectra with Fe3+/Fetot measured by Mössbauer spectroscopy.
Table S-1 Major and minor element compositions of garnet (Grt), olivine (Ol) and orthopyroxene (Opx) from samples BDN03 and UGL01. The Fe3+/ Fetot of garnet is also reported, together with the pressure and temperature used for the calculation of ƒO2.
BDN03 | UGL01 | ||||||||||||
BDN03_1 | BDN03_2 | BDN03_3 | UGL01_3 | UGL01_1 | UGL01_2 | ||||||||
wt. % | Grt rim | Grt core_1 | Grt core_2 | Ol | Opx | wt. % | Grt rim | Grt core_1 | Grt core_2 | Ol | Opx | ||
SiO2 | 42.06 | 41.78 | 42.16 | 40.96 | 58.25 | SiO2 | 42.18 | 42.83 | 42.78 | 41.52 | 58.52 | ||
TiO2 | 0.05 | 0.05 | 0.04 | 0.00 | 0.03 | TiO2 | 0.01 | 0.03 | 0.00 | 0.01 | 0.01 | ||
Al2O3 | 21.08 | 21.63 | 21.50 | 0.05 | 0.85 | Al2O3 | 19.82 | 20.47 | 20.15 | 0.01 | 0.61 | ||
Cr2O3 | 2.96 | 2.42 | 2.47 | 0.01 | 0.31 | Cr2O3 | 4.16 | 3.63 | 4.06 | 0.01 | 0.25 | ||
Fe2O3 | 0.36 | 0.45 | 0.40 | n.d. | n.d. | Fe2O3 | 0.32 | 0.11 | 0.29 | n.d. | n.d. | ||
FeO | 7.89 | 7.86 | 7.40 | 7.46 | 4.75 | FeO | 8.48 | 6.66 | 6.56 | 7.68 | 4.56 | ||
MnO | 0.54 | 0.41 | 0.36 | 0.14 | 0.11 | MnO | 0.49 | 0.38 | 0.27 | 0.13 | 0.09 | ||
MgO | 20.46 | 20.20 | 20.81 | 50.49 | 35.29 | MgO | 19.57 | 21.56 | 20.67 | 50.49 | 35.73 | ||
CaO | 4.37 | 4.83 | 4.61 | 0.01 | 0.26 | CaO | 5.00 | 4.28 | 4.85 | 0.02 | 0.20 | ||
Na2O | 0.04 | 0.04 | 0.01 | 0.02 | 0.03 | Na2O | 0.02 | 0.00 | 0.04 | 0.01 | 0.03 | ||
NiO | 0.03 | 0.02 | 0.00 | 0.39 | 0.08 | NiO | 0.03 | 0.00 | 0.00 | 0.42 | 0.08 | ||
K2O | 0.00 | 0.02 | 0.00 | 0.01 | 0.01 | K2O | 0.01 | 0.01 | 0.01 | 0.00 | 0.01 | ||
Total | 99.83 | 99.70 | 99.75 | 99.53 | 99.97 | Total | 100.09 | 99.95 | 99.68 | 100.29 | 100.08 | ||
Si | 3.010 | 2.993 | 3.007 | 0.999 | 1.992 | Si | 3.034 | 3.042 | 3.054 | 1.005 | 1.996 | ||
Ti | 0.003 | 0.003 | 0.002 | 0.000 | 0.001 | Ti | 0.000 | 0.002 | 0.000 | 0.000 | 0.000 | ||
Al | 1.778 | 1.826 | 1.808 | 0.002 | 0.034 | Al | 1.681 | 1.714 | 1.695 | 0.000 | 0.025 | ||
Cr | 0.167 | 0.137 | 0.139 | 0.000 | 0.008 | Cr | 0.237 | 0.204 | 0.229 | 0.000 | 0.007 | ||
Fe3+ | 0.019 | 0.024 | 0.021 | 0.000 | 0.000 | Fe3+ | 0.017 | 0.006 | 0.015 | 0.000 | 0.000 | ||
Fe2+ | 0.472 | 0.471 | 0.442 | 0.152 | 0.136 | Fe2+ | 0.510 | 0.395 | 0.392 | 0.155 | 0.130 | ||
Mn | 0.033 | 0.025 | 0.021 | 0.003 | 0.003 | Mn | 0.030 | 0.023 | 0.017 | 0.003 | 0.003 | ||
Mg | 2.183 | 2.157 | 2.213 | 1.836 | 1.799 | Mg | 2.100 | 2.283 | 2.200 | 1.822 | 1.817 | ||
Ca | 0.335 | 0.371 | 0.352 | 0.000 | 0.010 | Ca | 0.385 | 0.326 | 0.371 | 0.001 | 0.007 | ||
Na | 0.006 | 0.006 | 0.001 | 0.001 | 0.002 | Na | 0.002 | 0.000 | 0.006 | 0.000 | 0.002 | ||
Ni | 0.002 | 0.001 | 0.000 | 0.008 | 0.002 | Ni | 0.002 | 0.000 | 0.000 | 0.008 | 0.002 | ||
K | 0.000 | 0.002 | 0.000 | 0.000 | 0.000 | K | 0.001 | 0.001 | 0.000 | 0.000 | 0.000 | ||
Total | 8.008 | 8.015 | 8.007 | 3.001 | 3.987 | Total | 7.999 | 7.995 | 7.979 | 2.995 | 3.989 | ||
P (GPa) | 6.3 | 6.3 | 6.3 | P (GPa) | 7.6 | 7.6 | 7.6 | ||||||
T (˚C) | 1000 | 1000 | 1000 | T (˚C) | 1572 | 1572 | 1572 | ||||||
Fe3+/Fetot | 0.039 | 0.049 | 0.046 | Fe3+/Fetot | 0.033 | 0.014 | 0.038 | ||||||
ƒO2 | -3.47 | -3.16 | -3.21 | | | | ƒO2 | -4.88 | -6.36 | -4.75 |
Table S-2 Results of Fe K-edge XANES spectroscopy analyses. The intensities at 7138.4 eV and 7161.7 eV measured in garnet standards are reported with their ratio and the respective Fe3+/Fetot determined by Mössbauer spectroscopy. Intensities in garnet from samples UGL01 and BDN03 are reported together with their Fe3+/Fetot retrieved using the calibration line in Figure S-4.
Standards | ||||
ID | 7138.4 eV | 7161.7 eV | Intensity ratio | Mössbauer |
Dvk_1 | 0.868 | 1.057 | 1.219 | 0.105 |
Dvk_2 | 0.789 | 1.071 | 1.358 | 0.022 |
Dvk_3 | 0.873 | 1.061 | 1.215 | 0.105 |
Dvk_4 | 0.871 | 1.093 | 1.256 | 0.076 |
Dvk_6 | 0.858 | 1.073 | 1.252 | 0.066 |
Dvk_5 | 0.871 | 1.072 | 1.232 | 0.099 |
Dvk_7 | 0.874 | 1.054 | 1.207 | 0.101 |
Dvk_8 | 0.891 | 1.077 | 1.208 | 0.098 |
Dvk_10 | 0.858 | 1.074 | 1.251 | 0.060 |
LET6-2 | 0.877 | 1.118 | 1.275 | 0.043 |
LET-21 | 0.836 | 1.093 | 1.308 | 0.045 |
LET-7 | 0.838 | 1.102 | 1.314 | 0.048 |
LET-1 | 0.864 | 1.095 | 1.267 | 0.043 |
KIM44 | 0.843 | 1.063 | 1.261 | 0.069 |
KIM35 | 0.862 | 1.105 | 1.281 | 0.060 |
KIM30 | 0.841 | 1.087 | 1.292 | 0.052 |
LET9 | 0.877 | 1.063 | 1.212 | 0.095 |
KIM17 | 0.850 | 1.071 | 1.261 | 0.064 |
Kim39 | 0.869 | 1.135 | 1.306 | 0.051 |
Kim13 | 0.876 | 1.115 | 1.273 | 0.068 |
Kim1 | 0.869 | 1.094 | 1.259 | 0.054 |
Samples | ||||
ID | 7138.4 eV | 7161.7 eV | Intensity ratio | Fe3+/Fetot |
UGL01_1 | 0.83 | 1.09 | 1.314 | 0.033 |
UGL01_2 | 0.85 | 1.11 | 1.306 | 0.038 |
UGL01_3 | 0.82 | 1.10 | 1.341 | 0.014 |
BDN03_1 | 0.82 | 1.07 | 1.305 | 0.039 |
BDN03_2 | 0.86 | 1.11 | 1.291 | 0.049 |
BDN03_3 | 0.84 | 1.09 | 1.295 | 0.046 |