Apollo 15 green glass He-Ne-Ar signatures – In search for indigenous lunar noble gases
Affiliations | Corresponding Author | Cite as | Funding information-
Share this article
Article views:3,864Cumulative count of HTML views and PDF downloads.
- Download Citation
-
Rights & Permissions
top
Abstract
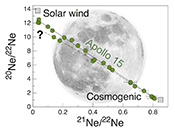
Figures and Tables
![]() Figure 1 Total 21Ne abundances of twelve single 15426 green glass beads. The uncertainty of 8 to 15 % is controlled by the precision in determining the sample mass. The 21Ne concentration range reported by Lakatos et al. (1973) for five grain size fractions and two large single spherules is indicated for comparison. |
![]() Figure 2 (a) Three-isotope plot of neon, (b) 20Ne/22Ne versus 36Ar/38Ar, (c) 36Ar/22Ne versus 21Ne/22Ne, and (d) 36Ar/22Ne versus the inverse of the 22Ne concentration measured in 15426 green glasses. Step-heating data for single glass beads from this study are shown together with results obtained by single-step heating of different grain size fractions and two large single spherules (Lakatos et al., 1973) and by laser ablation of individual spherules (Megrue, 1973). The neon and argon isotope compositions of the cosmogenic endmember (Cosm), modern solar wind (SW; Heber et al., 2009), and the meteoritic components A and Q (Ott, 2014) are shown for comparison. Error bars for the results from this study represent 1σ uncertainties and are, in some cases, smaller than symbol sizes. |
![]() Table 1 Abundances of cosmogenic 21Ne and 38Ar and corresponding cosmic ray exposure ages of twelve single 15426 green glass beads. |
![]() Figure 3 Cosmic ray exposure ages derived from the 21Necosm (T21) and 38Arcosm (T38) concentrations. Data for the four He-Ne-Ar-rich 15426 glass beads (open diamonds) and eight other spherules (filled diamonds) are shown together with the CRE ages determined previously (grey rectangle; Huneke et al., 1973; Lakatos et al., 1973; Megrue, 1973; Spangler et al., 1984). |
Figure 1 | Figure 2 | Table 1 | Figure 3 |
top
Introduction
The recent discovery of indigenous water and other highly volatile elements (C, F, S, Cl; Saal et al., 2008
Saal, A.E., Hauri, E.H., Lo Cascio, M., Van Orman, J.A., Rutherford, M.C., Cooper, R.F. (2008) Volatile content of lunar volcanic glasses and the presence of water in the Moon’s interior. Nature 454, 192–195.
; Sharp et al., 2010Sharp, Z.D., Shearer, C.K., McKeegan, K.D., Barnes, J.D., Wang, Y.Q. (2010). The chlorine isotope composition of the moon and implications for an anhydrous mantle. Science 329, 1050–1053.
; Wetzel et al., 2015Wetzel, D.T., Hauri, E.H., Saal, A.E., Rutherford, M.J. (2015) Carbon content and degassing history of the lunar volcanic glasses. Nature Geoscience 8, 755–758.
) in samples returned by the Apollo missions demonstrates that the formation and evolution of the Moon involved processes that allowed for the accretion and retention of the most volatile elements in the Solar System. Although the source and timing of volatile accretion is still debated (Hauri et al., 2015Hauri, E.H., Saal, A.E., Rutherford, M.J., Van Orman, J.A. (2015) Water in the Moon’s interior: Truth and consequences. Earth and Planetary Science Letters 409, 252–264.
; Barnes et al., 2016Barnes, J.J., Kring, D.A., Tartèse, R., Franchi, I.A., Anand, M., Russell, S.S. (2016) An asteroidal origin for water in the Moon. Nature Communications 7, 11684.
), these findings raise the question of whether the lunar mantle contains indigenous (i.e. primordial) noble gases that were supplied to the growing Moon by the delivery of volatile-rich chondritic matter or were inherited from the proto-Earth.The isotopic and/or elemental abundance signature of indigenous lunar noble gases could provide crucial constraints on the origin and evolution of the Moon. However, indigenous lunar noble gases have never been unambiguously found despite extensive searches over the past five decades (Wieler and Heber, 2003
Wieler, R., Heber, V.S. (2003) Noble gas isotopes on the Moon. Space Science Reviews 106, 197–210.
), although recent high precision analyses hint at the presence of indigenous xenon in lunar anorthosites (Bekaert et al., 2017Bekaert, D.V., Avice, G., Marty, B., Henderson, B., Gudipati, M.S. (2017) Stepwise heating of lunar anorthosites 60025, 60215, 65315 possibly reveals an indigenous noble gas component on the Moon. Geochimica et Cosmochimica Acta 218, 114–131.
). The key caveat is that noble gases of primordial origin can easily be masked by additional noble gas components. All rock and soil samples collected at the Moon’s surface contain 'trapped' surface-correlated noble gases implanted by SW irradiation. Volume-correlated noble gases comprise cosmogenic nuclides (3He, 21Ne, 38Ar) produced by spallation reactions, and radiogenic noble gases (4He, 40Ar) produced by the decay of long-lived radionuclides. Due to the modifications that occur during space exposure, discerning indigenous noble gases in currently available lunar samples is highly challenging.Lakatos et al. (1973)
Lakatos, S., Heymann, D., Yaniv, A. (1973) Green spherules from Apollo 15: Inferences about their origin from inert gas measurements. The Moon 7, 132–148.
proposed that green glass spherules from Apollo sample 15426 contain solar-like noble gases that may be of primordial origin. 15426 is a friable greenish clod that was collected by the Apollo 15 crew from the north rim of Spur Crater on the Apennine front. The green material is the common Apollo 15 very-low-Ti glass that was formed by volcanic fire-fountaining of primitive melts ~3.4 Gyr ago (Table S-1; Podosek and Huneke, 1973Podosek, F.A., Huneke, J.C. (1973) Argon in Apollo 15 green glass spherules (15426): 40Ar-39Ar age and trapped argon. Earth and Planetary Science Letters 19, 413–421.
; Delano, 1979Delano, J.W. (1979) Apollo 15 green glass: Chemistry and possible origin. Proceedings of the 10th Lunar and Planetary Science Conference, 275–300.
, 1986Delano, J.W. (1986) Pristine lunar glasses: criteria, data, and implications. Proceedings of the 16th Lunar and Planetary Science Conference, Part 2. Journal of Geophysical Research 91, D201–D213.
; Spangler et al., 1984Spangler, R.R., Warasila, R., Delano, J.W. (1984) 39Ar-40Ar ages for the Apollo 15 green and yellow glasses. Proceedings of the 14th Lunar and Planetary Science Conference, Part 2, Journal of Geophysical Research 89, B487–B497.
). Importantly, the presence of water, C, F, S, and Cl in the interior of the glass beads (Saal et al., 2008Saal, A.E., Hauri, E.H., Lo Cascio, M., Van Orman, J.A., Rutherford, M.C., Cooper, R.F. (2008) Volatile content of lunar volcanic glasses and the presence of water in the Moon’s interior. Nature 454, 192–195.
; Wetzel et al., 2015Wetzel, D.T., Hauri, E.H., Saal, A.E., Rutherford, M.J. (2015) Carbon content and degassing history of the lunar volcanic glasses. Nature Geoscience 8, 755–758.
), together with the condensation and enrichment of volatile elements on the glass surface (Delano, 1979Delano, J.W. (1979) Apollo 15 green glass: Chemistry and possible origin. Proceedings of the 10th Lunar and Planetary Science Conference, 275–300.
), requires the existence of a volatile-rich reservoir in the lunar mantle source.Step-wise gas extraction, combined with multi-element isotope analysis, provides the only means for resolving noble gases into constituent components, and for detecting indigenous gas in lunar samples. Therefore, in this study, we re-assess the noble gas signature of 15426 green glasses by step-wise CO2 laser extraction static mass spectrometry analysis (see Supplementary Information for details). Noble gas (He, Ne, Ar) concentrations and isotope ratios of twelve single vitreous glass beads, between 13 and 25 (±2) micrograms in mass, were measured using the Helix MC Plus noble gas mass spectrometer at CRPG in Nancy, France. Two heating steps were applied: a low temperature step (~600 °C) allowed extracting surface-sited, thermally labile gases, whereas the fusion step (~1500 °C) was aimed at releasing volume-correlated, more refractory noble gas components. Since neon isotopes were measured in multi-collection mode on three electron multipliers, the analytical precision on neon isotope ratios has improved by a factor of two compared to our previous study of orange lunar volcanic glasses (Füri et al., 2014
Füri, E., Deloule, E., Gurenko, A., Marty, B. (2014) New evidence for chondritic lunar water from combined D/H and noble gas analyses of single Apollo 17 volcanic glasses. Icarus 229, 109–120.
). Such high precision measurements are key for distinguishing different noble gas components in lunar samples.top
Noble Gas Signature of 15426 Green Glasses
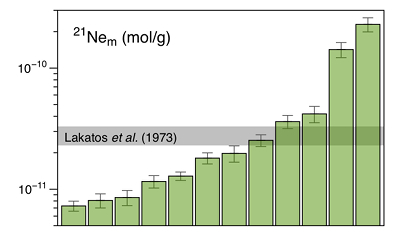
Figure 1 Total 21Ne abundances of twelve single 15426 green glass beads. The uncertainty of 8 to 15 % is controlled by the precision in determining the sample mass. The 21Ne concentration range reported by Lakatos et al. (1973)
Lakatos, S., Heymann, D., Yaniv, A. (1973) Green spherules from Apollo 15: Inferences about their origin from inert gas measurements. The Moon 7, 132–148.
for five grain size fractions and two large single spherules is indicated for comparison.The 4He content of several 15426 spherules analysed here is below the detection limit, implying that the glasses experienced severe diffusive helium losses. Any remaining helium is predominantly released at the first heating step, and, in most cases, shows an isotope ratio comparable to that of modern SW (Table S-2). These results indicate that the glass beads contain surface-sited noble gases implanted by SW irradiation, with only minor remnants of radiogenic 4He and cosmogenic 3He in the grain interior. The neon and argon abundances vary by two orders of magnitude (Figs. 1 and 2d), and the concentration range recorded by the small 15426 green glass beads analysed here is significantly greater than that reported by Lakatos et al. (1973)
Lakatos, S., Heymann, D., Yaniv, A. (1973) Green spherules from Apollo 15: Inferences about their origin from inert gas measurements. The Moon 7, 132–148.
for five grain size fractions and two large single spherules. 20Ne/22Ne and 21Ne/22Ne ratios vary between 12.50 and 1.18 and between 0.0350 and 0.815, respectively, and in a three-isotope plot of neon, most isotope signatures fall onto a mixing line between a high-20Ne/22Ne component (with 20Ne/22Ne = 12.42 ± 0.05 for 21Ne/22Ne = 0.03), interpreted to represent isotopically fractionated SW-derived neon, and cosmogenic neon (Fig. 2a). Similarly, 36Ar/38Ar ratios range from 5.51 to 0.91, also reflecting varying mixing proportions between SW-derived argon and a low-36Ar/38Ar cosmogenic component (Fig. 2b). The 'trapped' neon and argon component, implanted by SW irradiation, is predominantly released during the first heating step, whereas the cosmogenic isotopes are extracted during sample melting (Fig. 2). The solar gas component is isotopically depleted in the light neon isotopes compared to modern SW due to depth-dependent isotope fractionation upon implantation of SW and removal of surface-sited solar gas by ion sputtering (Wieler et al., 2007Wieler, R., Grimberg, A., Heber, V.S. (2007) Consequences of the non-existence of the “SEP” component for noble gas geo-and cosmochemistry. Chemical Geology 244, 382–390.
). Importantly, four glass beads stand out by having the highest noble gas contents and by showing a distinct gas release pattern; i.e. four spherules release large amounts of SW-derived helium, neon, and argon at low temperatures, and a volume-correlated component with high 20Ne/22Ne, 36Ar/38Ar, and 36Ar/22Ne ratios is extracted at the second heating step (Fig. 2). In the three-isotope plot of neon, this end member falls below the mixing line defined by the other glass beads, and points to a 20Ne/22Ne ratio of 11.41 ± 0.04 (for 21Ne/22Ne = 0.03).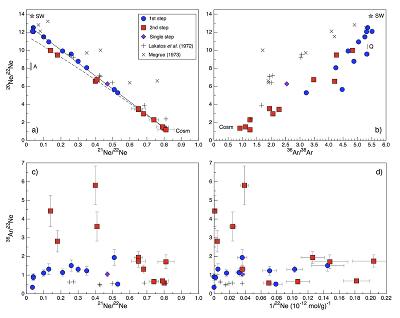
Figure 2 (a) Three-isotope plot of neon, (b) 20Ne/22Ne versus 36Ar/38Ar, (c) 36Ar/22Ne versus 21Ne/22Ne, and (d) 36Ar/22Ne versus the inverse of the 22Ne concentration measured in 15426 green glasses. Step-heating data for single glass beads from this study are shown together with results obtained by single-step heating of different grain size fractions and two large single spherules (Lakatos et al., 1973
Lakatos, S., Heymann, D., Yaniv, A. (1973) Green spherules from Apollo 15: Inferences about their origin from inert gas measurements. The Moon 7, 132–148.
) and by laser ablation of individual spherules (Megrue, 1973Megrue, G.H. (1973). Distribution of gases within Apollo 15 samples: implications for the incorporation of gases within solid bodies of the Solar System. Journal of Geophysical Research 78, 4875–4883.
). The neon and argon isotope compositions of the cosmogenic endmember (Cosm), modern solar wind (SW; Heber et al., 2009Heber, V.S., Wieler, R., Baur, H., Olinger, C., Friedmann, T.A., Burnett, D.S. (2009) Noble gas composition of the solar wind as collected by the Genesis mission. Geochimica et Cosmochimica Acta 73, 7414–7432.
), and the meteoritic components A and Q (Ott, 2014Ott, U. (2014) Planetary and pre-solar noble gases in meteorites. Chemie der Erde - Geochemistry 74, 519–544.
) are shown for comparison. Error bars for the results from this study represent 1σ uncertainties and are, in some cases, smaller than symbol sizes.The lack of correlation between the noble gas abundance and the grain size (i.e. the surface/volume ratio) lead Lakatos et al. (1973)
Lakatos, S., Heymann, D., Yaniv, A. (1973) Green spherules from Apollo 15: Inferences about their origin from inert gas measurements. The Moon 7, 132–148.
to propose that solar-like neon and argon in Apollo 15 green glasses may represent primordial lunar gas. However, the concentrations of 'trapped' solar neon and argon (Supplementary Information) found in 15426 glasses are several orders of magnitude greater than the Ne-Ar abundances in the 2ΠD43 popping rock, which is the least degassed terrestrial mid-ocean ridge basalt (Moreira et al., 1998Moreira, M., Kunz, J., Allègre, C. (1998) Rare gas systematics in Popping rock: Isotopic and elemental compositions in the upper mantle. Science 279, 1178-1181.
). Although an indigenous origin for noble gases in lunar volcanic glasses cannot be ruled out, it appears unlikely that i) lunar magmas escaped degassing, and ii) the lunar mantle contains a larger amount of neon and argon than the terrestrial upper mantle, given the Moon's general volatile element depletion compared to Earth (Hauri et al., 2015Hauri, E.H., Saal, A.E., Rutherford, M.J., Van Orman, J.A. (2015) Water in the Moon’s interior: Truth and consequences. Earth and Planetary Science Letters 409, 252–264.
). As an alternative to an indigenous origin, the melt that formed the 15426 green glasses may have assimilated SW-irradiated regolith upon eruption, resulting in dissolution of solar noble gases into the interior of the melt droplets before quenching (Lakatos et al., 1973Lakatos, S., Heymann, D., Yaniv, A. (1973) Green spherules from Apollo 15: Inferences about their origin from inert gas measurements. The Moon 7, 132–148.
). Since lunar regolith contains 1.5 to 2 wt. % carbonaceous chondrite-like material (e.g., Keays and Ganapathy, 1970Keays, R.R., Ganapathy, R. (1970) Trace elements and radioactivity in lunar rocks. Implications for meteorites infall, solar-wind flux, and formation conditions of Moon. Science 167, 490–493.
), regolith assimilation should be detectable through analyses of highly siderophile elements (HSEs). Walker et al. (2004)Walker, R.J., Horan, M.F., Shearer, C.K., Papike, J.J. (2004) Low abundances of highly siderophile elements in the lunar mantle: evidence for prolonged late accretion. Earth and Planetary Science Letters 224, 399–413.
argued that a significant proportion of chondritic HSEs is only present in the "etchate" of the surface of the green glasses, whereas concentrations are low in the residual glass. Another possibility is that the 15426 melt droplets trapped a gas phase that was released by heating and outgassing of vast quantities of solar wind-impregnated regolith (Lakatos et al., 1973Lakatos, S., Heymann, D., Yaniv, A. (1973) Green spherules from Apollo 15: Inferences about their origin from inert gas measurements. The Moon 7, 132–148.
). However, it is difficult to envision how high noble gas partial pressures, allowing for the dissolution of inert gases into the erupting melt, could have been maintained during fire-fountain style eruptions in near vacuum at the lunar surface. In addition, rapid quenching of the erupting melt droplets must have prevented ingassing of volatiles released from the regolith.In light of these caveats, the noble gas characteristics of the He-Ne-Ar-rich 15426 green glasses are best explained by inward diffusion of implanted SW-derived gas (with 20Ne/22Ne = 12.42 ± 0.05) – from the surface of the spherules into their interiors – during prolonged space exposure. When equilibrium conditions were established, the solar gases became volume- as opposed to surface-correlated (Lakatos et al., 1973
Lakatos, S., Heymann, D., Yaniv, A. (1973) Green spherules from Apollo 15: Inferences about their origin from inert gas measurements. The Moon 7, 132–148.
). Upon CO2 laser heating, the diffusive gas release fractionates the 20Ne/22Ne ratio according to:Eq. 1

where Rinitial is the initial (solar-like) 20Ne/22Ne ratio, f is the fraction of neon remaining in the glass, and α is the isotope fractionation factor, which can be approximated by

top
Irradiation History of 15426 Green Glasses
Since the 15426 glass beads contain a binary mixture of SW-derived and cosmogenic 20,21,22Ne and 36,38Ar, the amount of 21Necosm and 38Arcosm can be derived numerically for each sample, based on the isotopic signature of the two end members (see Supplementary Information for details on the component deconvolution). The concentrations of cosmogenic 21Ne and 38Ar correspond to cosmic ray exposure (CRE) ages (T21 and T38, respectively) that agree within uncertainties, and vary between 87 ± 9 and 347 ± 73 Myr in most cases (Fig. 3 and Table 1). These CRE ages are comparable to the values of 139 to 400 Myr obtained by previous noble gas analyses of 15426 green glasses (Huneke et al., 1973
Huneke, J.C., Podosek, F.A., Wasserburg, G.J. (1973) An argon bouillabaisse including ages from the Luna 20 site. Abstracts of the Lunar and Planetary Science Conference 4, 403–404.
; Lakatos et al., 1973Lakatos, S., Heymann, D., Yaniv, A. (1973) Green spherules from Apollo 15: Inferences about their origin from inert gas measurements. The Moon 7, 132–148.
; Megrue, 1973Megrue, G.H. (1973). Distribution of gases within Apollo 15 samples: implications for the incorporation of gases within solid bodies of the Solar System. Journal of Geophysical Research 78, 4875–4883.
; Spangler et al., 1984Spangler, R.R., Warasila, R., Delano, J.W. (1984) 39Ar-40Ar ages for the Apollo 15 green and yellow glasses. Proceedings of the 14th Lunar and Planetary Science Conference, Part 2, Journal of Geophysical Research 89, B487–B497.
). However, three out of the four gas-rich glass beads clearly stand out by having high concentrations of cosmogenic 21Ne and 38Ar, reflecting extremely high exposure ages (T38) between 542 ± 87 and 2139 ± 303 Myr (Fig. 3 and Table 1). This indicates that these spherules were exposed to cosmic, and possibly solar wind, irradiation at the (sub-) surface of the Moon for a much longer duration than the majority of the Apollo 15 green glasses which could have resulted in inward diffusion of SW-derived gases, facilitated by radiation damage. At the same time, diffusive loss of 21Necosm likely occurred, thus severely affecting the T21 exposure ages. These observations demonstrate that, due to intense stirring of the regolith by impact gardening, each individual lunar volcanic glass bead records its own unique irradiation history, which is accessible only through single grain analyses.Table 1 Abundances of cosmogenic 21Ne and 38Ar and corresponding cosmic ray exposure ages of twelve single 15426 green glass beads.
Sample ID | 21Necosm | % total | T21 | 38Arcosm | % total | T38 |
(pmol/g) | (Myr) | (pmol/g) | (Myr) | |||
15426-4 | 7.1 | 97 | 105 ± 21 | 3.7 | 59 | 87 ± 9 |
15426-11 | 7.8 | 96 | 116 ± 26 | 4.2 | 52 | 98 ± 14 |
15426-2 | 8.3 | 98 | 125 ± 29 | 4.2 | 64 | 97 ± 15 |
15426-5 | 10.7 | 92 | 160 ± 34 | 5.9 | 41 | 137 ± 17 |
15426-I | 12.4 | 97 | 185 ± 36 | 7.0 | 62 | 163 ± 15 |
15426-13* | 13.1 | 36 | 196 ± 43 | 13.0 | 9 | 303 ± 41 |
15426-II | 17.9 | 99 | 267 ± 56 | 9.8 | 87 | 228 ± 26 |
15426-10 | 19.4 | 98 | 289 ± 68 | 11.0 | 51 | 255 ± 41 |
15426-6 | 23.2 | 92 | 347 ± 73 | 13.5 | 44 | 315 ± 38 |
15426-12* | 35.3 | 84 | 527 ± 124 | 23.3 | 25 | 542 ± 87 |
15426-III* | 47.8 | 34 | 714 ± 164 | 64.6 | 10 | 1503 ± 227 |
15426-3* | 75.4 | 33 | 1125 ± 251 | 92.0 | 10 | 2139 ± 303 |
The four glass beads with the highest He-Ne-Ar abundances (Table S-2) are identified by asterisks.
Uncertainties for the 21Necosm and 38Arcosm concentrations are on the order of 10 to 15 %.
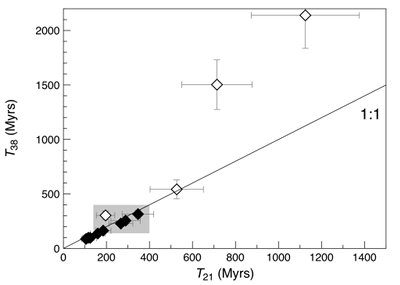
Figure 3 Cosmic ray exposure ages derived from the 21Necosm (T21) and 38Arcosm (T38) concentrations. Data for the four He-Ne-Ar-rich 15426 glass beads (open diamonds) and eight other spherules (filled diamonds) are shown together with the CRE ages determined previously (grey rectangle; Huneke et al., 1973
Huneke, J.C., Podosek, F.A., Wasserburg, G.J. (1973) An argon bouillabaisse including ages from the Luna 20 site. Abstracts of the Lunar and Planetary Science Conference 4, 403–404.
; Lakatos et al., 1973Lakatos, S., Heymann, D., Yaniv, A. (1973) Green spherules from Apollo 15: Inferences about their origin from inert gas measurements. The Moon 7, 132–148.
; Megrue, 1973Megrue, G.H. (1973). Distribution of gases within Apollo 15 samples: implications for the incorporation of gases within solid bodies of the Solar System. Journal of Geophysical Research 78, 4875–4883.
; Spangler et al., 1984Spangler, R.R., Warasila, R., Delano, J.W. (1984) 39Ar-40Ar ages for the Apollo 15 green and yellow glasses. Proceedings of the 14th Lunar and Planetary Science Conference, Part 2, Journal of Geophysical Research 89, B487–B497.
).top
In Search of Indigenous Lunar Noble Gases
The hydrogen (and nitrogen) isotopic signature of lunar samples is currently best explained by late accretion of volatile-rich chondritic material to the Moon, although the possibility that some volatiles were inherited from the proto-Earth cannot be ruled out (e.g., Füri et al., 2014
Füri, E., Deloule, E., Gurenko, A., Marty, B. (2014) New evidence for chondritic lunar water from combined D/H and noble gas analyses of single Apollo 17 volcanic glasses. Icarus 229, 109–120.
; Hauri et al., 2015Hauri, E.H., Saal, A.E., Rutherford, M.J., Van Orman, J.A. (2015) Water in the Moon’s interior: Truth and consequences. Earth and Planetary Science Letters 409, 252–264.
; Barnes et al., 2016Barnes, J.J., Kring, D.A., Tartèse, R., Franchi, I.A., Anand, M., Russell, S.S. (2016) An asteroidal origin for water in the Moon. Nature Communications 7, 11684.
). Could indigenous lunar noble gases provide further constraints on the origin of volatiles in the lunar interior, and, thus, on the formation and evolution of the Moon? Noble gas elemental ratios must have been fractionated throughout the volatile accretion process(es) by the Moon and/or upon partial melting and degassing during magmatic eruption; thus they are unlikely to preserve the original source characteristics. Noble gas isotope ratios represent excellent volatile source tracers; nonetheless identifying a chondritic or terrestrial provenance may be highly challenging because secondary contributions from the SW and/or cosmogenic nuclides hamper the identification of the indigenous component.The terrestrial mantle is characterised by 20Ne/22Ne and 36Ar/38Ar ratios of 12.65 and 5.3, respectively (Péron et al., 2017
Péron, S., Moreira, M., Putlitz, B., Kurz, M.D. (2017) Solar wind implantation supplied light volatiles during the first stage of Earth accretion. Geochemical Perspective Letters 3, 151–159.
); thus, transfer of noble gases from Earth to the Moon-forming material would have resulted in isotope compositions that are very similar to those of the 'trapped' component in Apollo 15426 green glasses. SW-irradiated chondritic dust – with a high surface/volume ratio – would be characterised by comparable noble gas isotope signatures; however small dust grains can be ruled out as the dominant contributors to the lunar volatile inventory on the basis of predominantly non-solar hydrogen and nitrogen isotope signatures of Apollo samples. For large chondritic bodies, the neon inventory is dominated by neon in presolar diamonds (Ne-A), whereas the argon budget is dominated by the Q component. These end members are characterised by 20Ne/22Ne and 36Ar/38Ar ratios of ~8.2 and 5.34, respectively (Fig. 2a,b; Ott, 2014Ott, U. (2014) Planetary and pre-solar noble gases in meteorites. Chemie der Erde - Geochemistry 74, 519–544.
). Therefore, step-heating neon extraction, combined with precise isotope analysis by state-of-the-art static vacuum mass spectrometry, would allow a distinction between a chondritic and a terrestrial provenance of lunar volatiles. Although high-precision (He-)Ne-Ar data can now be obtained for very small (≤25 µg) lunar volcanic glasses, new lunar samples are needed to detect a possible indigenous noble gas component, and to identify unambiguously its provenance. Pristine samples that have never been exposed to space at the lunar surface, i.e. rock samples retrieved by drilling from depths of several metres or fresh crater ejecta, are key for assessing whether the lunar mantle retains indigenous noble gases that were supplied to the growing Moon by the delivery of volatile-rich chondritic matter or were inherited from the proto-Earth.top
Acknowledgements
We thank two anonymous reviewers for their constructive comments. This work was supported by the European Research Council (grant no. 715028 to E.F.). This is CRPG-CNRS contribution 2601.
Editor: Cin-Ty Lee
top
References
Barnes, J.J., Kring, D.A., Tartèse, R., Franchi, I.A., Anand, M., Russell, S.S. (2016) An asteroidal origin for water in the Moon. Nature Communications 7, 11684.

Although the source and timing of volatile accretion is still debated (Hauri et al., 2015; Barnes et al., 2016), these findings raise the question of whether the lunar mantle contains indigenous (i.e. primordial) noble gases that were supplied to the growing Moon by the delivery of volatile-rich chondritic matter or were inherited from the proto-Earth.
View in article
The hydrogen (and nitrogen) isotopic signature of lunar samples is currently best explained by late accretion of volatile-rich chondritic material to the Moon, although the possibility that some volatiles were inherited from the proto-Earth cannot be ruled out (e.g., Füri et al., 2014; Hauri et al., 2015; Barnes et al., 2016).
View in article
Bekaert, D.V., Avice, G., Marty, B., Henderson, B., Gudipati, M.S. (2017) Stepwise heating of lunar anorthosites 60025, 60215, 65315 possibly reveals an indigenous noble gas component on the Moon. Geochimica et Cosmochimica Acta 218, 114–131.

However, indigenous lunar noble gases have never been unambiguously found despite extensive searches over the past five decades (Wieler and Heber, 2003), although recent high precision analyses hint at the presence of indigenous xenon in lunar anorthosites (Bekaert et al., 2017).
View in article
Delano, J.W. (1979) Apollo 15 green glass: Chemistry and possible origin. Proceedings of the 10th Lunar and Planetary Science Conference, 275–300.

The green material is the common Apollo 15 very-low-Ti glass that was formed by volcanic fire-fountaining of primitive melts ~3.4 Gyr ago (Table S-1; Podosek and Huneke, 1973; Delano, 1979, 1986; Spangler et al., 1984).
View in article
Importantly, the presence of water, C, F, S, and Cl in the interior of the glass beads (Saal et al., 2008; Wetzel et al., 2015), together with the condensation and enrichment of volatile elements on the glass surface (Delano, 1979), requires the existence of a volatile-rich reservoir in the lunar mantle source.
View in article
Delano, J.W. (1986) Pristine lunar glasses: criteria, data, and implications. Proceedings of the 16th Lunar and Planetary Science Conference, Part 2. Journal of Geophysical Research 91, D201–D213.

The green material is the common Apollo 15 very-low-Ti glass that was formed by volcanic fire-fountaining of primitive melts ~3.4 Gyr ago (Table S-1; Podosek and Huneke, 1973; Delano, 1979, 1986; Spangler et al., 1984).
View in article
Füri, E., Deloule, E., Gurenko, A., Marty, B. (2014) New evidence for chondritic lunar water from combined D/H and noble gas analyses of single Apollo 17 volcanic glasses. Icarus 229, 109–120.

Since neon isotopes were measured in multi-collection mode on three electron multipliers, the analytical precision on neon isotope ratios has improved by a factor of two compared to our previous study of orange lunar volcanic glasses (Füri et al., 2014).
View in article
The hydrogen (and nitrogen) isotopic signature of lunar samples is currently best explained by late accretion of volatile-rich chondritic material to the Moon, although the possibility that some volatiles were inherited from the proto-Earth cannot be ruled out (e.g., Füri et al., 2014; Hauri et al., 2015; Barnes et al., 2016).
View in article
Hauri, E.H., Saal, A.E., Rutherford, M.J., Van Orman, J.A. (2015) Water in the Moon’s interior: Truth and consequences. Earth and Planetary Science Letters 409, 252–264.

Although the source and timing of volatile accretion is still debated (Hauri et al., 2015; Barnes et al., 2016), these findings raise the question of whether the lunar mantle contains indigenous (i.e. primordial) noble gases that were supplied to the growing Moon by the delivery of volatile-rich chondritic matter or were inherited from the proto-Earth.
View in article
Although an indigenous origin for noble gases in lunar volcanic glasses cannot be ruled out, it appears unlikely that i) lunar magmas escaped degassing, and ii) the lunar mantle contains a larger amount of neon and argon than the terrestrial upper mantle, given the Moon's general volatile element depletion compared to Earth (Hauri et al., 2015).
View in article
The hydrogen (and nitrogen) isotopic signature of lunar samples is currently best explained by late accretion of volatile-rich chondritic material to the Moon, although the possibility that some volatiles were inherited from the proto-Earth cannot be ruled out (e.g., Füri et al., 2014; Hauri et al., 2015; Barnes et al., 2016).
View in article
Heber, V.S., Wieler, R., Baur, H., Olinger, C., Friedmann, T.A., Burnett, D.S. (2009) Noble gas composition of the solar wind as collected by the Genesis mission. Geochimica et Cosmochimica Acta 73, 7414–7432.

Figure 2 [...] The neon and argon isotope compositions of the cosmogenic endmember (Cosm), modern solar wind (SW; Heber et al., 2009), and the meteoritic components A and Q (Ott, 2014) are shown for comparison.
View in article
Huneke, J.C., Podosek, F.A., Wasserburg, G.J. (1973) An argon bouillabaisse including ages from the Luna 20 site. Abstracts of the Lunar and Planetary Science Conference 4, 403–404.

These CRE ages are comparable to the values of 139 to 400 Myr obtained by previous noble gas analyses of 15426 green glasses (Huneke et al., 1973; Lakatos et al., 1973; Megrue, 1973; Spangler et al., 1984).
View in article
Figure 3 [...] Data for the four He-Ne-Ar-rich 15426 glass beads (open diamonds) and eight other spherules (filled diamonds) are shown together with the CRE ages determined previously (grey rectangle; Huneke et al., 1973; Lakatos et al., 1973; Megrue, 1973; Spangler et al., 1984).
View in article
Keays, R.R., Ganapathy, R. (1970) Trace elements and radioactivity in lunar rocks. Implications for meteorites infall, solar-wind flux, and formation conditions of Moon. Science 167, 490–493.

Since lunar regolith contains 1.5 to 2 wt. % carbonaceous chondrite-like material (e.g., Keays and Ganapathy, 1970), regolith assimilation should be detectable through analyses of highly siderophile elements (HSEs).
View in article
Lakatos, S., Heymann, D., Yaniv, A. (1973) Green spherules from Apollo 15: Inferences about their origin from inert gas measurements. The Moon 7, 132–148.

Lakatos et al. (1973) proposed that green glass spherules from Apollo sample 15426 contain solar-like noble gases that may be of primordial origin. 15426 is a friable greenish clod that was collected by the Apollo 15 crew from the north rim of Spur Crater on the Apennine front.
View in article
Figure 1 [...] The 21Ne concentration range reported by Lakatos et al. (1973) for five grain size fractions and two large single spherules is indicated for comparison.
View in article
The neon and argon abundances vary by two orders of magnitude (Figs. 1 and 2d), and the concentration range recorded by the small 15426 green glass beads analysed here is significantly greater than that reported by Lakatos et al. (1973) for five grain size fractions and two large single spherules.
View in article
Figure 2 [...] Step-heating data for single glass beads from this study are shown together with results obtained by single-step heating of different grain size fractions and two large single spherules (Lakatos et al., 1973) and by laser ablation of individual spherules (Megrue, 1973).
View in article
The lack of correlation between the noble gas abundance and the grain size (i.e. the surface/volume ratio) lead Lakatos et al. (1973) to propose that solar-like neon and argon in Apollo 15 green glasses may represent primordial lunar gas.
View in article
As an alternative to an indigenous origin, the melt that formed the 15426 green glasses may have assimilated SW-irradiated regolith upon eruption, resulting in dissolution of solar noble gases into the interior of the melt droplets before quenching (Lakatos et al., 1973).
View in article
Another possibility is that the 15426 melt droplets trapped a gas phase that was released by heating and outgassing of vast quantities of solar wind-impregnated regolith (Lakatos et al., 1973).
View in article
When equilibrium conditions were established, the solar gases became volume- as opposed to surface-correlated (Lakatos et al., 1973).
View in article
These CRE ages are comparable to the values of 139 to 400 Myr obtained by previous noble gas analyses of 15426 green glasses (Huneke et al., 1973; Lakatos et al., 1973; Megrue, 1973; Spangler et al., 1984).
View in article
Figure 3 [...] Data for the four He-Ne-Ar-rich 15426 glass beads (open diamonds) and eight other spherules (filled diamonds) are shown together with the CRE ages determined previously (grey rectangle; Huneke et al., 1973; Lakatos et al., 1973; Megrue, 1973; Spangler et al., 1984).
View in article
Megrue, G.H. (1973). Distribution of gases within Apollo 15 samples: implications for the incorporation of gases within solid bodies of the Solar System. Journal of Geophysical Research 78, 4875–4883.

Figure 2 [...] Step-heating data for single glass beads from this study are shown together with results obtained by single-step heating of different grain size fractions and two large single spherules (Lakatos et al., 1973) and by laser ablation of individual spherules (Megrue, 1973).
View in article
These CRE ages are comparable to the values of 139 to 400 Myr obtained by previous noble gas analyses of 15426 green glasses (Huneke et al., 1973; Lakatos et al., 1973; Megrue, 1973; Spangler et al., 1984).
View in article
Figure 3 [...] Data for the four He-Ne-Ar-rich 15426 glass beads (open diamonds) and eight other spherules (filled diamonds) are shown together with the CRE ages determined previously (grey rectangle; Huneke et al., 1973; Lakatos et al., 1973; Megrue, 1973; Spangler et al., 1984).
View in article
Moreira, M., Kunz, J., Allègre, C. (1998) Rare gas systematics in Popping rock: Isotopic and elemental compositions in the upper mantle. Science 279, 1178-1181.

However, the concentrations of 'trapped' solar neon and argon (Supplementary Information) found in 15426 glasses are several orders of magnitude greater than the Ne-Ar abundances in the 2ΠD43 popping rock, which is the least degassed terrestrial mid-ocean ridge basalt (Moreira et al., 1998).
View in article
Ott, U. (2014) Planetary and pre-solar noble gases in meteorites. Chemie der Erde - Geochemistry 74, 519–544.

Figure 2 [...] The neon and argon isotope compositions of the cosmogenic endmember (Cosm), modern solar wind (SW; Heber et al., 2009), and the meteoritic components A and Q (Ott, 2014) are shown for comparison.
View in article
These end members are characterised by 20Ne/22Ne and 36Ar/38Ar ratios of ~8.2 and 5.34, respectively (Fig. 2a,b; Ott, 2014)
View in article
Péron, S., Moreira, M., Putlitz, B., Kurz, M.D. (2017) Solar wind implantation supplied light volatiles during the first stage of Earth accretion. Geochemical Perspective Letters 3, 151–159.

The terrestrial mantle is characterised by 20Ne/22Ne and 36Ar/38Ar ratios of 12.65 and 5.3, respectively (Péron et al., 2017); thus, transfer of noble gases from Earth to the Moon-forming material would have resulted in isotope compositions that are very similar to those of the 'trapped' component in Apollo 15426 green glasses.
View in article
Podosek, F.A., Huneke, J.C. (1973) Argon in Apollo 15 green glass spherules (15426): 40Ar-39Ar age and trapped argon. Earth and Planetary Science Letters 19, 413–421.

The green material is the common Apollo 15 very-low-Ti glass that was formed by volcanic fire-fountaining of primitive melts ~3.4 Gyr ago (Table S-1; Podosek and Huneke, 1973; Delano, 1979, 1986; Spangler et al., 1984).
View in article
Saal, A.E., Hauri, E.H., Lo Cascio, M., Van Orman, J.A., Rutherford, M.C., Cooper, R.F. (2008) Volatile content of lunar volcanic glasses and the presence of water in the Moon’s interior. Nature 454, 192–195.

The recent discovery of indigenous water and other highly volatile elements (C, F, S, Cl; Saal et al., 2008; Sharp et al., 2010; Wetzel et al., 2015) in samples returned by the Apollo missions demonstrates that the formation and evolution of the Moon involved processes that allowed for the accretion and retention of the most volatile elements in the Solar System.
View in article
Importantly, the presence of water, C, F, S, and Cl in the interior of the glass beads (Saal et al., 2008; Wetzel et al., 2015), together with the condensation and enrichment of volatile elements on the glass surface (Delano, 1979), requires the existence of a volatile-rich reservoir in the lunar mantle source.
View in article
Sharp, Z.D., Shearer, C.K., McKeegan, K.D., Barnes, J.D., Wang, Y.Q. (2010). The chlorine isotope composition of the moon and implications for an anhydrous mantle. Science 329, 1050–1053.

The recent discovery of indigenous water and other highly volatile elements (C, F, S, Cl; Saal et al., 2008; Sharp et al., 2010; Wetzel et al., 2015) in samples returned by the Apollo missions demonstrates that the formation and evolution of the Moon involved processes that allowed for the accretion and retention of the most volatile elements in the Solar System.
View in article
Spangler, R.R., Warasila, R., Delano, J.W. (1984) 39Ar-40Ar ages for the Apollo 15 green and yellow glasses. Proceedings of the 14th Lunar and Planetary Science Conference, Part 2, Journal of Geophysical Research 89, B487–B497.

The green material is the common Apollo 15 very-low-Ti glass that was formed by volcanic fire-fountaining of primitive melts ~3.4 Gyr ago (Table S-1; Podosek and Huneke, 1973; Delano, 1979, 1986; Spangler et al., 1984).
View in article
These CRE ages are comparable to the values of 139 to 400 Myr obtained by previous noble gas analyses of 15426 green glasses (Huneke et al., 1973; Lakatos et al., 1973; Megrue, 1973; Spangler et al., 1984).
View in article
Figure 3 [...] Data for the four He-Ne-Ar-rich 15426 glass beads (open diamonds) and eight other spherules (filled diamonds) are shown together with the CRE ages determined previously (grey rectangle; Huneke et al., 1973; Lakatos et al., 1973; Megrue, 1973; Spangler et al., 1984).
View in article
Walker, R.J., Horan, M.F., Shearer, C.K., Papike, J.J. (2004) Low abundances of highly siderophile elements in the lunar mantle: evidence for prolonged late accretion. Earth and Planetary Science Letters 224, 399–413.

Walker et al. (2004) argued that a significant proportion of chondritic HSEs is only present in the "etchate" of the surface of the green glasses, whereas concentrations are low in the residual glass.
View in article
Wetzel, D.T., Hauri, E.H., Saal, A.E., Rutherford, M.J. (2015) Carbon content and degassing history of the lunar volcanic glasses. Nature Geoscience 8, 755–758.

The recent discovery of indigenous water and other highly volatile elements (C, F, S, Cl; Saal et al., 2008; Sharp et al., 2010; Wetzel et al., 2015) in samples returned by the Apollo missions demonstrates that the formation and evolution of the Moon involved processes that allowed for the accretion and retention of the most volatile elements in the Solar System.
View in article
Importantly, the presence of water, C, F, S, and Cl in the interior of the glass beads (Saal et al., 2008; Wetzel et al., 2015), together with the condensation and enrichment of volatile elements on the glass surface (Delano, 1979), requires the existence of a volatile-rich reservoir in the lunar mantle source.
View in article
Wieler, R., Heber, V.S. (2003) Noble gas isotopes on the Moon. Space Science Reviews 106, 197–210.

However, indigenous lunar noble gases have never been unambiguously found despite extensive searches over the past five decades (Wieler and Heber, 2003), although recent high precision analyses hint at the presence of indigenous xenon in lunar anorthosites (Bekaert et al., 2017).
View in article
Wieler, R., Grimberg, A., Heber, V.S. (2007) Consequences of the non-existence of the “SEP” component for noble gas geo-and cosmochemistry. Chemical Geology 244, 382–390.

The solar gas component is isotopically depleted in the light neon isotopes compared to modern SW due to depth-dependent isotope fractionation upon implantation of SW and removal of surface-sited solar gas by ion sputtering (Wieler et al., 2007).
View in article
top
Supplementary Information
The Supplementary Information includes:
- Major Element Composition of 15426 Green Glasses
- Noble Gas (He, Ne, Ar) Analysis
- Noble Gas Component Deconvolution and Exposure Ages
- Figures S-1 and S-2
- Tables S-1 and S-2
- Supplementary Information References
Download the Supplementary Information (PDF).
Figures and Tables
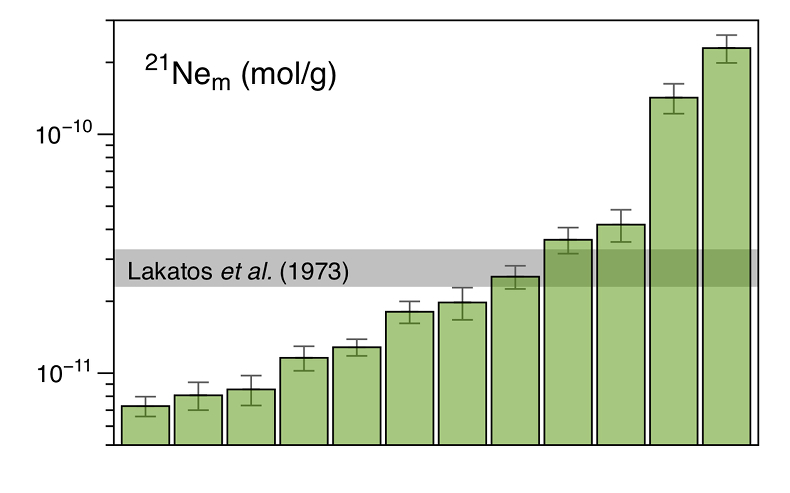
Figure 1 Total 21Ne abundances of twelve single 15426 green glass beads. The uncertainty of 8 to 15 % is controlled by the precision in determining the sample mass. The 21Ne concentration range reported by Lakatos et al. (1973)
Lakatos, S., Heymann, D., Yaniv, A. (1973) Green spherules from Apollo 15: Inferences about their origin from inert gas measurements. The Moon 7, 132–148.
for five grain size fractions and two large single spherules is indicated for comparison.
Figure 2 (a) Three-isotope plot of neon, (b) 20Ne/22Ne versus 36Ar/38Ar, (c) 36Ar/22Ne versus 21Ne/22Ne, and (d) 36Ar/22Ne versus the inverse of the 22Ne concentration measured in 15426 green glasses. Step-heating data for single glass beads from this study are shown together with results obtained by single-step heating of different grain size fractions and two large single spherules (Lakatos et al., 1973
Lakatos, S., Heymann, D., Yaniv, A. (1973) Green spherules from Apollo 15: Inferences about their origin from inert gas measurements. The Moon 7, 132–148.
) and by laser ablation of individual spherules (Megrue, 1973Megrue, G.H. (1973). Distribution of gases within Apollo 15 samples: implications for the incorporation of gases within solid bodies of the Solar System. Journal of Geophysical Research 78, 4875–4883.
). The neon and argon isotope compositions of the cosmogenic endmember (Cosm), modern solar wind (SW; Heber et al., 2009Heber, V.S., Wieler, R., Baur, H., Olinger, C., Friedmann, T.A., Burnett, D.S. (2009) Noble gas composition of the solar wind as collected by the Genesis mission. Geochimica et Cosmochimica Acta 73, 7414–7432.
), and the meteoritic components A and Q (Ott, 2014Ott, U. (2014) Planetary and pre-solar noble gases in meteorites. Chemie der Erde - Geochemistry 74, 519–544.
) are shown for comparison. Error bars for the results from this study represent 1σ uncertainties and are, in some cases, smaller than symbol sizes.Table 1 Abundances of cosmogenic 21Ne and 38Ar and corresponding cosmic ray exposure ages of twelve single 15426 green glass beads.
Sample ID | 21Necosm | % total | T21 | 38Arcosm | % total | T38 |
(pmol/g) | (Myr) | (pmol/g) | (Myr) | |||
15426-4 | 7.1 | 97 | 105 ± 21 | 3.7 | 59 | 87 ± 9 |
15426-11 | 7.8 | 96 | 116 ± 26 | 4.2 | 52 | 98 ± 14 |
15426-2 | 8.3 | 98 | 125 ± 29 | 4.2 | 64 | 97 ± 15 |
15426-5 | 10.7 | 92 | 160 ± 34 | 5.9 | 41 | 137 ± 17 |
15426-I | 12.4 | 97 | 185 ± 36 | 7.0 | 62 | 163 ± 15 |
15426-13* | 13.1 | 36 | 196 ± 43 | 13.0 | 9 | 303 ± 41 |
15426-II | 17.9 | 99 | 267 ± 56 | 9.8 | 87 | 228 ± 26 |
15426-10 | 19.4 | 98 | 289 ± 68 | 11.0 | 51 | 255 ± 41 |
15426-6 | 23.2 | 92 | 347 ± 73 | 13.5 | 44 | 315 ± 38 |
15426-12* | 35.3 | 84 | 527 ± 124 | 23.3 | 25 | 542 ± 87 |
15426-III* | 47.8 | 34 | 714 ± 164 | 64.6 | 10 | 1503 ± 227 |
15426-3* | 75.4 | 33 | 1125 ± 251 | 92.0 | 10 | 2139 ± 303 |
The four glass beads with the highest He-Ne-Ar abundances (Table S-2) are identified by asterisks.
Uncertainties for the 21Necosm and 38Arcosm concentrations are on the order of 10 to 15 %.
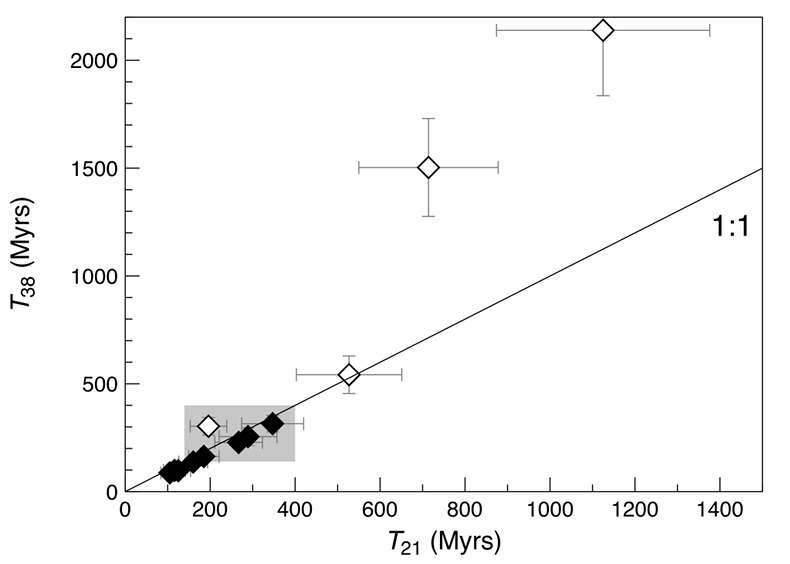
Figure 3 Cosmic ray exposure ages derived from the 21Necosm (T21) and 38Arcosm (T38) concentrations. Data for the four He-Ne-Ar-rich 15426 glass beads (open diamonds) and eight other spherules (filled diamonds) are shown together with the CRE ages determined previously (grey rectangle; Huneke et al., 1973
Huneke, J.C., Podosek, F.A., Wasserburg, G.J. (1973) An argon bouillabaisse including ages from the Luna 20 site. Abstracts of the Lunar and Planetary Science Conference 4, 403–404.
; Lakatos et al., 1973Lakatos, S., Heymann, D., Yaniv, A. (1973) Green spherules from Apollo 15: Inferences about their origin from inert gas measurements. The Moon 7, 132–148.
; Megrue, 1973Megrue, G.H. (1973). Distribution of gases within Apollo 15 samples: implications for the incorporation of gases within solid bodies of the Solar System. Journal of Geophysical Research 78, 4875–4883.
; Spangler et al., 1984Spangler, R.R., Warasila, R., Delano, J.W. (1984) 39Ar-40Ar ages for the Apollo 15 green and yellow glasses. Proceedings of the 14th Lunar and Planetary Science Conference, Part 2, Journal of Geophysical Research 89, B487–B497.
).