A lunar hygrometer based on plagioclase-melt partitioning of water
Affiliations | Corresponding Author | Cite as | Funding information- Share this article
Article views:2,951Cumulative count of HTML views and PDF downloads.
- Download Citation
- Rights & Permissions
top
Abstract

Figures and Tables
![]() Figure 1 Backscattered electron (BSE) images of representative experimental run products (Pl2_LBS7H, 0.4 GPa – 1200 °С; and 2018_Pl3_LBS7H, 0.3 GPa – 1160 °С). Px = pyroxene; Plag = plagioclase. | ![]() Figure 2 Representative unpolarised infrared spectra of plagioclase, normalised to 1 cm thickness. Spectra are shifted vertically to facilitate comparison. | ![]() Table 1 Summary of experimental conditions and water contents of run products in our experiments and literature data. | ![]() Figure 3 Partition coefficients of water between plagioclase and melt from this study and literature data (Hamada et al., 2013; Caseres et al., 2017), plotted versus (a) oxygen fugacity, and (b) water concentration in silicate melt. Melt inclusion data (Hamada et al., 2013) in the dotted box are not used in this study because the formation temperature of these inclusions and the degree of water loss from inclusions after formation are uncertain. |
Figure 1 | Figure 2 | Table 1 | Figure 3 |
top
Introduction
The canonical view of a dry lunar interior has been challenged by detections of hydrogen (H or OH, reported here as equivalent amounts of H2O in μg/g) in picritic glass beads (Saal et al., 2008
Saal, A.E., Hauri, E.H., Cascio, M.L., Van Orman, J.A., Rutherford, M.C., Cooper, R.F. (2008) Volatile content of lunar volcanic glasses and the presence of water in the Moon’s interior. Nature 454, 192–195.
), apatites (e.g., McCubbin et al., 2010McCubbin, F.M., Steele, A., Hauri, E.H., Nekvasil, H., Yamashita, S., Hemley, R.J. (2010) Nominally hydrous magmatism on the Moon. Proceedings of the National Academy of Sciences of the United States of America 107, 11223–11228.
; Lin and van Westrenen, 2019Lin, Y.H., van Westrenen, W. (2019) Isotopic evidence for volatile replenishment of the Moon during Late Accretion. National Science Review, doi: 10.1093/nsr/nwz033.
), olivine-hosted melt inclusions (e.g., Hauri et al., 2011Hauri, E.H., Weinreich, T., Saal, A.E., Rutherford, M.C., van Orman, J.A. (2011) High pre-eruptive water contents preserved in lunar melt inclusions. Science 213, 10–13.
) and plagioclases (Hui et al., 2013Hui, H., Peslier, A.H., Zhang, Y., Neal, C.R. (2013) Water in lunar anorthosites and evidence for a wet early moon. Nature Geoscience 6, 177–180.
). Sample-based inferences about water in the Moon have been complemented by experimental and modelling studies of lunar magma ocean (LMO) crystallisation (Elkins-Tanton and Grove, 2011Elkins-Tanton, L.T., Grove, T.L. (2011) Water (hydrogen) in the lunar mantle: Results from petrology and magma ocean modeling. Earth and Planetary Science Letters 307, 173–179.
; Lin et al., 2017aLin, Y.H., Tronche, E.J., Steenstra, E.S., van Westrenen, W. (2017a) Evidence for an early wet Moon from experimental crystallization of the lunar magma ocean. Nature Geoscience 10, 14–18.
,bLin, Y.H., Tronche, E.J., Steenstra, E.S., van Westrenen, W. (2017b) Experimental constraints on the solidification of a nominally dry lunar magma ocean. Earth and Planetary Science Letters 471, 104–116.
; Charlier et al., 2018Charlier, B., Grove, T.L., Namur, O., Holtz, F. (2018) Crystallization of the lunar magma ocean and the primordial mantle-crust differentiation of the Moon. Geochimica et Cosmochimica Acta 234, 50–69.
; Rapp and Draper, 2018Rapp, J.F., Draper, D.S. (2018) Fractional crystallization of the lunar magma ocean: Updating the dominant paradigm. Meteoritics and Planetary Science 53, 1432–1455.
). Quantification of the evolution of the lunar interior volatile budget would provide further insight into the thermal and magmatic evolution of the Moon. However, converting hydrogen abundance data measured in lunar samples or estimated from laboratory experiments to models of the temporal and spatial evolution of water in the Moon, is far from straightforward (McCubbin et al., 2015aMcCubbin, F.M., Vander Kaaden, K.E., Tartèse, R., Boyce, J.W., Mikhail, S., Whitson, E.S., Bell, A.S., Anand, M., Franchi, I.A., Wang, J., Hauri, E.H. (2015a) Experimental investigation of F, Cl, and OH partitioning between apatite and Fe-rich basaltic melt at 1.0–1.2 GPa and 950–1000 ºC. American Mineralogist 100, 1790–1802.
).This study focuses on improving constraints on the water content in the Moon specifically during the LMO stage. Plagioclase is thought to have crystallised and floated to the surface during the late stages of LMO crystallisation, forming the lunar primary feldspathic crust (Warren, 1985
Warren, P.H. (1985) The magma ocean concept and lunar evolution. Annual Review of Earth and Planetary Sciences 13, 201–240.
). This indicates that plagioclase in lunar ferroan anorthosite could be our best candidate for estimating the water content of the LMO (Hui et al., 2013Hui, H., Peslier, A.H., Zhang, Y., Neal, C.R. (2013) Water in lunar anorthosites and evidence for a wet early moon. Nature Geoscience 6, 177–180.
, 2017Hui H., Guan, Y., Chen, Y., Peslier, A.H., Zhang, Y., Liu, Y., Flemming, R.L., Rossman, G.R., Eiler, J.M., Neal, C.R., Osinski, G.R. (2017) A heterogeneous lunar interior for hydrogen isotopes as revealed by the lunar highlands samples. Earth and Planetary Science Letters 473, 14–23.
). In addition, plagioclase could have formed continuously from ~70 % all the way up to >99 % of LMO crystallisation (Lin et al., 2017aLin, Y.H., Tronche, E.J., Steenstra, E.S., van Westrenen, W. (2017a) Evidence for an early wet Moon from experimental crystallization of the lunar magma ocean. Nature Geoscience 10, 14–18.
; Charlier et al., 2018Charlier, B., Grove, T.L., Namur, O., Holtz, F. (2018) Crystallization of the lunar magma ocean and the primordial mantle-crust differentiation of the Moon. Geochimica et Cosmochimica Acta 234, 50–69.
; Rapp and Draper, 2018Rapp, J.F., Draper, D.S. (2018) Fractional crystallization of the lunar magma ocean: Updating the dominant paradigm. Meteoritics and Planetary Science 53, 1432–1455.
). Therefore, the water content of plagioclase formed at different stages during LMO crystallisation could in principle be used to track and quantify the LMO water content through time.While nominally anhydrous, terrestrial plagioclase can incorporate trace amounts of H as structural OH and/or molecular H2O. In magmatic feldspars, concentrations from less than a few to more than 1000 μg/g H2O have been reported (Johnson and Rossman, 2003
Johnson, E.A., Rossman, G.R. (2003) The concentration and speciation of hydrogen in feldspars using FTIR and 1H MAS NMR spectroscopy. American Mineralogist 88, 901–911.
, 2004Johnson, E.A., Rossman, G.R. (2004) A survey of hydrous species and concentrations in igneous feldspars. American Mineralogist 89, 586–600.
; Johnson, 2006Johnson, E.A. (2006) Water in nominally anhydrous crustal minerals: speciation, concentration, and geologic significance. Reviews in Mineralogy and Geochemistry 62, 117–154.
; Mosenfelder et al., 2015Mosenfelder, J.L., Rossman, G.R., Johnson, E.A. (2015) Hydrous species in feldspars: A reassessment based on FTIR and SIMS. American Mineralogist 100, 1209–1221.
). Only very few studies have measured water contents of lunar feldspars from a primary crystallisation product of the LMO so far (Hui et al., 2013Hui, H., Peslier, A.H., Zhang, Y., Neal, C.R. (2013) Water in lunar anorthosites and evidence for a wet early moon. Nature Geoscience 6, 177–180.
; 2017Hui H., Guan, Y., Chen, Y., Peslier, A.H., Zhang, Y., Liu, Y., Flemming, R.L., Rossman, G.R., Eiler, J.M., Neal, C.R., Osinski, G.R. (2017) A heterogeneous lunar interior for hydrogen isotopes as revealed by the lunar highlands samples. Earth and Planetary Science Letters 473, 14–23.
).To link the water content in plagioclase to that in the melt from which the mineral crystallised, plagioclase-melt partition coefficients D of water are required, with Dplag-melt water = Cplagwater/Cmeltwater. Literature values for Dplag-melt water range between 0.002 ± 0.0004 and 0.006 ± 0.0009 (recalculated using the plagioclase absorption coefficient determined by Mosenfelder et al. (2015)
Mosenfelder, J.L., Rossman, G.R., Johnson, E.A. (2015) Hydrous species in feldspars: A reassessment based on FTIR and SIMS. American Mineralogist 100, 1209–1221.
based on measurements carried out in both natural and experimental systems, which have focused solely on magmatism on Earth (e.g., Hamada et al., 2013Hamada, M., Ushioda, M., Fujii, T., Takahashi, E. (2013) Hydrogen concentration in plagioclase as a hygrometer of arc basaltic melts: Approaches from melt inclusion analyses and hydrous melting experiments. Earth and Planetary Science Letters 365, 253–262.
). To date, no plagioclase-melt partition coefficient of water under lunar conditions has been published. This is problematic, for example in terms of oxygen fugacity, as it has previously been suggested that ƒO2 can affect hydrogen solubility in plagioclase (Yang, 2012Yang, X. (2012) An experimental study of H solubility in feldspars: Effect of composition, oxygen fugacity, temperature and pressure and implications for crustal processes. Geochimica et Cosmochimica Acta 97, 46–57.
). The available Dplag-melt water data, which are applied to terrestrial systems, were obtained at relatively oxidising conditions. The ƒO2 in the Moon is thought to be significantly lower, at ~IW to ~IW-2 (IW: iron-wustite) (Sato et al., 1973Sato, M., Hickling, N.L., McLane, J.E. (1973) Oxygen fugacity values of Apollo 12, 14, and 15 lunar samples and reduced state of lunar magmas. Proceedings of the Lunar Science Conference 1, 1061–1079.
; Rutherford and Papale, 2009Rutherford, M.J., Papale, P. (2009) Origin of basalt fire-fountain eruptions on Earth versus the Moon. Geology 37, 219–222.
) based on sample analyses. In addition, although Yang (2012)Yang, X. (2012) An experimental study of H solubility in feldspars: Effect of composition, oxygen fugacity, temperature and pressure and implications for crustal processes. Geochimica et Cosmochimica Acta 97, 46–57.
suggests plagioclase composition, temperature and pressure have insignificant effects on Dplag-meltwater , this suggestion was based on experiments conducted in a limited temperature-pressure range.In this study, Dplag-melt water was determined at pressure-temperature-composition conditions occurring in the lunar magma ocean using high pressure and high temperature experiments and Fourier-Transform Infrared Spectroscopy (FTIR). The pressures (0.4–0.6 GPa) and temperatures (1130–1220 °C) were chosen to be consistent with plagioclase formation during crystallisation of a water-bearing lunar magma ocean (Lin et al., 2017a
Lin, Y.H., Tronche, E.J., Steenstra, E.S., van Westrenen, W. (2017a) Evidence for an early wet Moon from experimental crystallization of the lunar magma ocean. Nature Geoscience 10, 14–18.
). The main purposes of this paper are: (1) to quantify the effects of composition and ƒO2 on Dplag-meltwater , and (2) to offer further constraints on the water content of the LMO at the time of plagioclase crystallisation.top
Water Partition Coefficients
Details of high pressure, high temperature, plagioclase-melt partitioning experiments are given in the Supplementary Information. Table 1 provides a summary of experimental P-T-fO2 conditions. Starting compositions, EMPA analyses of the major element concentrations in plagioclase and melt phases in the experimental run products, and log(fO2) calculations are shown in Tables S-1 and S-2 of the Supplementary Information. All experimental charges contain plagioclase, pyroxene, and quenched glass (Fig. 1). One experimental charge contains Fe metal in addition, indicating an oxygen fugacity at or below that of the iron-wüstite buffer. Representative unpolarised FTIR spectra of plagioclase are shown in Figure 2. All plagioclases show absorption bands (~3000–3600 cm-1) in the mid-infrared region typical of O–H bonds (Johnson and Rossman, 2004
Johnson, E.A., Rossman, G.R. (2004) A survey of hydrous species and concentrations in igneous feldspars. American Mineralogist 89, 586–600.
). No H2 bands have been observed in our plagioclase spectra. Further descriptions are shown in the Supplementary Information.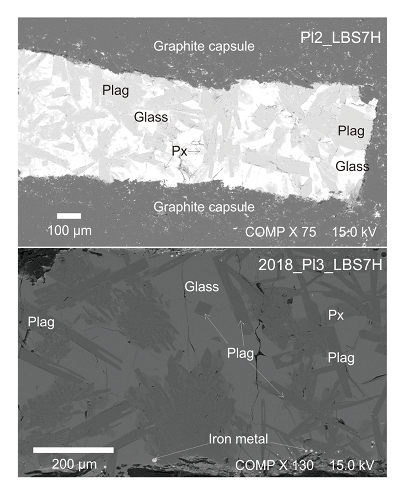
Figure 1 Backscattered electron (BSE) images of representative experimental run products (Pl2_LBS7H, 0.4 GPa – 1200 °С; and 2018_Pl3_LBS7H, 0.3 GPa – 1160 °С). Px = pyroxene; Plag = plagioclase.
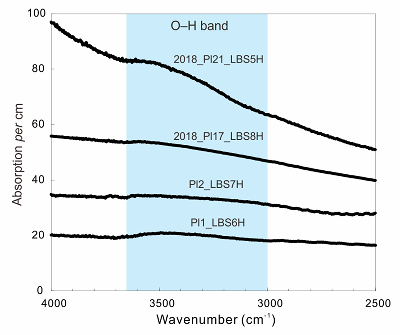
Figure 2 Representative unpolarised infrared spectra of plagioclase, normalised to 1 cm thickness. Spectra are shifted vertically to facilitate comparison.
Table 1 gives FTIR-derived H2O concentrations in plagioclase and glass from our work, Hamada et al. (2013)
Hamada, M., Ushioda, M., Fujii, T., Takahashi, E. (2013) Hydrogen concentration in plagioclase as a hygrometer of arc basaltic melts: Approaches from melt inclusion analyses and hydrous melting experiments. Earth and Planetary Science Letters 365, 253–262.
and Caseres et al. (2017)Caseres, J.R., Mosenfelder, J.L., Hirschmann, M.M. (2017) Partitioning of hydrogen and fluorine between feldspar and melt under the conditions of lunar crust formation. Lunar and Planetary Science Conference 48, 2303.
. The H2O equivalent concentrations in our samples range from 42 ± 6 to 99 ± 36 μg/g in plagioclase and 0.13 ± 0.01 to 1.74 ± 0.01 wt. % in silicate glass. The corresponding partition coefficients range between 0.0020 ± 0.0004 and 0.0460 ± 0.0096.The H2O concentrations in our plagioclase crystals are significantly below water solubility at our experimental conditions (Yang, 2012
Yang, X. (2012) An experimental study of H solubility in feldspars: Effect of composition, oxygen fugacity, temperature and pressure and implications for crustal processes. Geochimica et Cosmochimica Acta 97, 46–57.
). Sample 2018_Pl17_LBS8H, with glass containing the highest water concentration (1.74 wt. % H2O), has the lowest water concentration in plagioclase (42 μg/g H2O) and hence the lowest partition coefficient (Dplag-melt water = 0.0020 ± 0.0004). This lowest value is at the lower end of the range of previously published partition coefficients (Dplag-melt water = 0.002–0.006) by Hamada et al. (2013)Hamada, M., Ushioda, M., Fujii, T., Takahashi, E. (2013) Hydrogen concentration in plagioclase as a hygrometer of arc basaltic melts: Approaches from melt inclusion analyses and hydrous melting experiments. Earth and Planetary Science Letters 365, 253–262.
. Our highest partition coefficient is ~7–20 times higher than values from the Hamada et al. (2013)Hamada, M., Ushioda, M., Fujii, T., Takahashi, E. (2013) Hydrogen concentration in plagioclase as a hygrometer of arc basaltic melts: Approaches from melt inclusion analyses and hydrous melting experiments. Earth and Planetary Science Letters 365, 253–262.
data set. The water partition coefficients reported by Caseres et al. (2017Caseres, J.R., Mosenfelder, J.L., Hirschmann, M.M. (2017) Partitioning of hydrogen and fluorine between feldspar and melt under the conditions of lunar crust formation. Lunar and Planetary Science Conference 48, 2303.
; Dplag-melt water = 0.018–0.023 at the IW buffer) overlap with our results.Table 1 Summary of experimental conditions and water contents of run products in our experiments and literature data.
Sample | Conditions | Plagioclase | Glass | Water partition coefficient | Oxygen fugacity | ||||||||
P (GPa) | T °С | Duration (h) | OH (μg/g H2O) | n | 1 s.d. | OH (wt.% H2O) | n | 1 s.d. | Dplag-melt | 1 s.d. | Oxygen buffer | Log (ƒO2) | |
This study | |||||||||||||
Pl1_LBS6H | 0.4 | 1160 | 14 | 58.2 | 11 | 17.9 | 1.03 | 9 | 0.16 | 0.006 | 0.0017 | Graphite–COH (C–COH) | -10.4 |
Pl2_LBS7H | 1200 | 16 | 63.2 | 9 | 32.8 | 0.35 | 10 | 0.02 | 0.018 | 0.0092 | -10.0 | ||
Pl3_LBS8H_1 | 1160 | 22 | 96.0 | 4 | 28.8 | 0.24 | 12 | 0.09 | 0.040 | 0.0120 | -10.4 | ||
Pl4_LBS8H_2 | 1180 | 14 | 85.5 | 5 | 33.8 | 0.63 | 8 | 0.04 | 0.014 | 0.0054 | -10.2 | ||
Pl5_LBS8H_3 | 1180 | 18 | 99.1 | 6 | 36.4 | 0.32 | 10 | 0.01 | 0.030 | 0.0112 | -10.2 | ||
2018_Pl2_LBS8H | 1170 | 24 | 73.4 | 7 | 17.0 | 0.22 | 8 | 0.02 | 0.034 | 0.0083 | -10.3 | ||
2018_Pl17_LBS8H | 1180 | 24 | 42.2 | 8 | 6.64 | 1.74 | 9 | 0.01 | 0.002 | 0.0004 | -10.2 | ||
2018_Pl21_LBS5H | 1190 | 24 | 54.2 | 6 | 14.2 | 0.13 | 8 | 0.01 | 0.043 | 0.0116 | -10.1 | ||
2018_Pl3_LBS7H | 0.3 | 1160 | 24 | 61.1 | 7 | 12.6 | 0.13 | 11 | 0.004 | 0.046 | 0.0096 | Iron-Wustite (IW) | -12.4 |
Caseres et al. (2017) Caseres, J.R., Mosenfelder, J.L., Hirschmann, M.M. (2017) Partitioning of hydrogen and fluorine between feldspar and melt under the conditions of lunar crust formation. Lunar and Planetary Science Conference 48, 2303. | |||||||||||||
1# | 0.8 | 1150 | 82 | 11 | 0.36 | 0.003 | 0.023 | 0.003 | Iron-Wustite (IW) | -12.3 | |||
2# | 118 | 6 | 0.65 | 0.008 | 0.018 | 0.001 | -12.3 | ||||||
Hamada et al. (2013) Hamada, M., Ushioda, M., Fujii, T., Takahashi, E. (2013) Hydrogen concentration in plagioclase as a hygrometer of arc basaltic melts: Approaches from melt inclusion analyses and hydrous melting experiments. Earth and Planetary Science Letters 365, 253–262. | |||||||||||||
MTL04 | 0.35 | 1130 | 24 | 89.8 | 13.5 | 3.70 | 0.56 | 0.002 | 0.0004 | > Ni–NiO (NNO) | |||
MTL05 | 1170 | 24 | 80.8 | 12.1 | 2.50 | 0.38 | 0.003 | 0.0005 | |||||
MTL17 | 1220 | 24 | 35.9 | 5.4 | 0.90 | 0.14 | 0.004 | 0.0006 | |||||
MTL22 | 1130 | 24 | 36.0 | 5.4 | 2.30 | 0.35 | 0.002 | 0.0002 | -4.7 | ||||
MTL26 | 1160 | 24 | 30.1 | 4.5 | 0.70 | 0.11 | 0.004 | 0.0006 | |||||
MTL29 | 1170 | 24 | 45.4 | 6.8 | 0.90 | 0.14 | 0.005 | 0.0008 | -5.2 | ||||
MTL33 | 1230 | 24 | 44.4 | 6.7 | 1.00 | 0.15 | 0.004 | 0.0007 | -5.8 | ||||
MTL37 | 1070 | 24 | 111 | 16.6 | 4.70 | 0.71 | 0.002 | 0.0004 | -5.2 | ||||
MTL39 | 1100 | 24 | 82.9 | 12.4 | 3.50 | 0.53 | 0.002 | 0.0004 | |||||
MTL40 | 1100 | 24 | 121 | 18.2 | 4.60 | 0.69 | 0.003 | 0.0004 | -4.7 | ||||
MTL41 | 1050 | 24 | 119 | 17.8 | 6.00 | 0.90 | 0.002 | 0.0003 | |||||
Melt Inclusion | |||||||||||||
Pl19-MI | 15.3 | 2.3 | 0.32 | 0.05 | 0.005 | 0.0007 | Fe2SiO4–Fe3O4–SiO2 (FMQ) | ||||||
Pl21-MI | 10.6 | 1.6 | 0.24 | 0.04 | 0.004 | 0.0007 | |||||||
Pl22-MI | 16.4 | 2.5 | 0.26 | 0.04 | 0.006 | 0.0009 |
Note: n, number of analysed plagioclases; s.d., 1 sigma standard deviation; Log ƒO2(buffer) corrected in the Supplementary Information;
Melt inclusion data not used in this study because we do not know exact T, P, and whether there was any water loss from the inclusions after formation;
The latest infrared absorption coefficient (Mosenfelder et al., 2015Mosenfelder, J.L., Rossman, G.R., Johnson, E.A. (2015) Hydrous species in feldspars: A reassessment based on FTIR and SIMS. American Mineralogist 100, 1209–1221.) was used for calibrating water contents of all plagioclases.
top
The Effects of Oxygen Fugacity and Water Content in Melt
It has been shown that a number of parameters can affect water partitioning between nominally anhydrous minerals and silicate melts, including the presence and abundance of chemical impurities and vacancies, the possibility of substitutions with charge-balancing species, temperature, pressure, and oxygen fugacity (e.g., Yang, 2012
Yang, X. (2012) An experimental study of H solubility in feldspars: Effect of composition, oxygen fugacity, temperature and pressure and implications for crustal processes. Geochimica et Cosmochimica Acta 97, 46–57.
and references therein). Previous work on water solubility in feldspar has shown that there is no obvious compositional dependence on the incorporation of H in the feldspar group except for a possible link with potassium content or sodium-hydrogen diffusion during heating (Yang, 2012Yang, X. (2012) An experimental study of H solubility in feldspars: Effect of composition, oxygen fugacity, temperature and pressure and implications for crustal processes. Geochimica et Cosmochimica Acta 97, 46–57.
; Johnson and Rossman, 2013Johnson, E.A., Rossman, G.R. (2013) The diffusion behavior of hydrogen in plagioclase feldspar at 800-1000°C: Implications for re-equilibration of OH in volcanic phenocrysts. American Mineralogist 98, 1779–1787.
). Potassium, however, has very low concentrations in ferroan anorthositic plagioclase, <0.03 wt. % (Dixon and Papike, 1975Dixon, J.R., Papike, J.J. (1975) Petrology of anorthosites from the Descartes region of the Moon: Apollo 16. Lunar and Planetary Science Conference 6, 263–291.
) and is absent in our experiments. Pressure and temperature effects cannot be assessed on the basis of our experiments, and those of Hamada et al. (2013)Hamada, M., Ushioda, M., Fujii, T., Takahashi, E. (2013) Hydrogen concentration in plagioclase as a hygrometer of arc basaltic melts: Approaches from melt inclusion analyses and hydrous melting experiments. Earth and Planetary Science Letters 365, 253–262.
and Caseres et al. (2017)Caseres, J.R., Mosenfelder, J.L., Hirschmann, M.M. (2017) Partitioning of hydrogen and fluorine between feldspar and melt under the conditions of lunar crust formation. Lunar and Planetary Science Conference 48, 2303.
, due to the overall limited pressure (0.3–0.8 GPa) and temperature (1000–1230 ºC) range.The H solubility in Fe-poor plagioclase near the IW buffer was demonstrated to be more than twice that determined at more oxidising conditions (Yang, 2012
Yang, X. (2012) An experimental study of H solubility in feldspars: Effect of composition, oxygen fugacity, temperature and pressure and implications for crustal processes. Geochimica et Cosmochimica Acta 97, 46–57.
), leading to the hypothesis that oxygen fugacity could cause the difference between data obtained at lunar conditions, including our data and the data of Caseres et al. (2017)Caseres, J.R., Mosenfelder, J.L., Hirschmann, M.M. (2017) Partitioning of hydrogen and fluorine between feldspar and melt under the conditions of lunar crust formation. Lunar and Planetary Science Conference 48, 2303.
, and those obtained at terrestrial conditions, i.e. the data of Hamada et al. (2013)Hamada, M., Ushioda, M., Fujii, T., Takahashi, E. (2013) Hydrogen concentration in plagioclase as a hygrometer of arc basaltic melts: Approaches from melt inclusion analyses and hydrous melting experiments. Earth and Planetary Science Letters 365, 253–262.
. However, Figure 3a shows that there is no correlation between Dplag-meltwater and fO2 in the overall data set. The terrestrial data set at relatively oxidising conditions yields lower Dplag-meltwater than the lunar data sets obtained at lower fO2, similar to the trend between oxygen fugacity and hydrogen solubility in plagioclase (Yang, 2012Yang, X. (2012) An experimental study of H solubility in feldspars: Effect of composition, oxygen fugacity, temperature and pressure and implications for crustal processes. Geochimica et Cosmochimica Acta 97, 46–57.
). One possibility is that the number of vacancies available for hydrogen incorporation is increased at low fO2, for example due to the enhanced incorporation of divalent iron in Al sites (Mosenfelder et al., 2019Mosenfelder, J.L., Andrys, J.L., Caseres, J.R., Hirschmann, M.M. (2019) Water in the Moon: The perspective from nominally anhydrous minerals. Lunar and Planetary Science Conference 50, 2132.
). However, although high values are found at low fO2, some low-fO2 experiments show low Dplag-meltwater (Fig. 3a). Oxygen fugacity is therefore not the main factor affecting Dplag-melt water . Instead, the experiments suggest that the water content of the silicate melt plays a key role in determining the partition coefficient of water between plagioclase and melt (Fig. 3b). There is an inverse relationship between the measured Dplag-melt water and the water concentration in silicate melt. In the absence of a theoretical framework to guide the functional form used to describe these inverse relations, we provide the following best-fit equations:Eq. 1a
Dplag-melt water = -6·10-2·x + 0.05 (x ≤ 0.7; R2 = 0.85),
Eq. 1b
Dplag-meltwater = -5·10-4·x + 0.005 (x > 0.7; R2 = 0.60),
where x is wt. % H2O in the silicate melt. Equations 1a and 1b provide quantitative estimates of Dplag-melt water given ranges of the magma oxygen fugacity and water content in silicate melt.
The exact mechanism controlling the observed variations of Dplag-melt water with ƒO2 and water content cannot be derived from our experiments, and it is not feasible to construct a thermodynamics-based predictive model of Dplag-melt water with the currently available data sets. Clearly water partitioning shows strong non-Henrian behaviour in our experiments, pointing to non-ideal behaviour of the relevant hydrogen-bearing species in mineral and/or melt. Previous work has suggested that changes in the OH site in plagioclases occur as a function of plagioclase OH content (Hamada et al. (2013)
Hamada, M., Ushioda, M., Fujii, T., Takahashi, E. (2013) Hydrogen concentration in plagioclase as a hygrometer of arc basaltic melts: Approaches from melt inclusion analyses and hydrous melting experiments. Earth and Planetary Science Letters 365, 253–262.
), but we cannot identify variations in the shape of the FTIR spectra that would be consistent with such a change in our experiments. It therefore seems more likely that the non-Henrian behaviour is caused by water activity coefficient changes in the melt. The dominant hydrogen-bearing species in hydrous melts at the hydrogen levels in our experiments could be OH (Stolper, 1982Stolper, E. (1982) Water in silicate glasses: An infrared spectroscopic study. Contributions to Mineralogy and Petrology 81, 1–17.
; Newcombe et al., 2017Newcombe, M.E., Brett, A., Beckett, J.R., Baker, M.B., Newman, S., Guan, Y., Eiler, J.M., Stolper, E.M. (2017) Solubility of water in lunar basalt at low pH2O. Geochimica et Cosmochimica Acta 200, 330–352.
), but non-linear increases in the H2O/OH ratio with increasing silicate melt hydrogen content have previously been observed (Stolper, 1982Stolper, E. (1982) Water in silicate glasses: An infrared spectroscopic study. Contributions to Mineralogy and Petrology 81, 1–17.
). These speciation changes affect the OH activity in the silicate melts, consistent with the observed trend in Dplag-meltwater values, though future work is needed to quantify this correlation.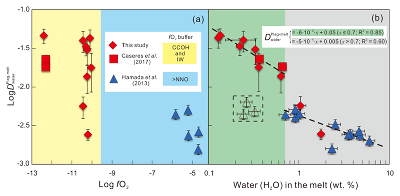
Figure 3 Partition coefficients of water between plagioclase and melt from this study and literature data (Hamada et al., 2013
Hamada, M., Ushioda, M., Fujii, T., Takahashi, E. (2013) Hydrogen concentration in plagioclase as a hygrometer of arc basaltic melts: Approaches from melt inclusion analyses and hydrous melting experiments. Earth and Planetary Science Letters 365, 253–262.
; Caseres et al., 2017Caseres, J.R., Mosenfelder, J.L., Hirschmann, M.M. (2017) Partitioning of hydrogen and fluorine between feldspar and melt under the conditions of lunar crust formation. Lunar and Planetary Science Conference 48, 2303.
), plotted versus (a) oxygen fugacity, and (b) water concentration in silicate melt. Melt inclusion data (Hamada et al., 2013Hamada, M., Ushioda, M., Fujii, T., Takahashi, E. (2013) Hydrogen concentration in plagioclase as a hygrometer of arc basaltic melts: Approaches from melt inclusion analyses and hydrous melting experiments. Earth and Planetary Science Letters 365, 253–262.
) in the dotted box are not used in this study because the formation temperature of these inclusions and the degree of water loss from inclusions after formation are uncertain.top
Water Content of the Lunar Magma Ocean
Equation 1a can be used to calculate the water content of the LMO at lunar oxygen fugacity conditions. This calculation requires estimates of (a) the abundance of water in lunar plagioclase, and (b) the degree of crystallisation of the LMO at the time of plagioclase formation.
The latest study published to date on the water content of lunar plagioclase from a primary crystallisation product of the LMO reported ~5 μg/g water (H2O) in ferroan anorthosite samples including Apollo sample 60015 (Hui et al., 2017
Hui H., Guan, Y., Chen, Y., Peslier, A.H., Zhang, Y., Liu, Y., Flemming, R.L., Rossman, G.R., Eiler, J.M., Neal, C.R., Osinski, G.R. (2017) A heterogeneous lunar interior for hydrogen isotopes as revealed by the lunar highlands samples. Earth and Planetary Science Letters 473, 14–23.
). We constrained the degree of crystallisation of the LMO when this plagioclase was formed by comparing the Mg# (molar (MgO/MgO + FeO) x 100) of plagioclase from sample 60015 (Mg# of 17–47) (Dixon and Papike, 1975Dixon, J.R., Papike, J.J. (1975) Petrology of anorthosites from the Descartes region of the Moon: Apollo 16. Lunar and Planetary Science Conference 6, 263–291.
) to the Mg# of plagioclase formed at different stages from our recent experimental study of LMO crystallisation (Lin et al., 2017aLin, Y.H., Tronche, E.J., Steenstra, E.S., van Westrenen, W. (2017a) Evidence for an early wet Moon from experimental crystallization of the lunar magma ocean. Nature Geoscience 10, 14–18.
,bLin, Y.H., Tronche, E.J., Steenstra, E.S., van Westrenen, W. (2017b) Experimental constraints on the solidification of a nominally dry lunar magma ocean. Earth and Planetary Science Letters 471, 104–116.
). The first plagioclase formed during LMO crystallisation has a Mg# of ~60. This Mg# decreases with progressive crystallisation. Plagioclase with Mg# as low as 17–47 forms after ~95 % crystallisation of the LMO.Based on the above constraints, the amount of H2O equivalent in the residual LMO after ~95 % solidification of the initial magma ocean is calculated to be ~100 μg/g by solving the equation Dplag-melt water = 5/Cmeltwater = -6·10-2·(Cmeltwater/10000) + 0.05 (here, C meltwater is in μg/g). If the LMO hydrogen budget remained constant throughout LMO solidification, this implies a very low initial LMO water content of just 5 μg/g H2O equivalent, consistent with inferences from petrology and magma ocean modelling (Elkins-Tanton and Grove, 2011
Elkins-Tanton, L.T., Grove, T.L. (2011) Water (hydrogen) in the lunar mantle: Results from petrology and magma ocean modeling. Earth and Planetary Science Letters 307, 173–179.
), lunar sample measurements (McCubbin et al., 2015bMcCubbin, F.M., Vander Kaaden, K.E., Tartèse, R., Klima, R.L., Liu, Y., Mortimer, J., Barnes, J., Shearer, C.K., Treiman, A.H., Lawrence, D.J., Elardo, S.M., Hurley, D.M., Boyce, J.W., Anand, M. (2015b) Magmatic volatiles (H, C, N, F, S, Cl) in the lunar mantle, crust, and regolith: Abundances, distributions, processes, and reservoirs. American Mineralogist 100, 1668–1707.
), and the experimental LMO solidification studies of Rapp and Draper (2018)Rapp, J.F., Draper, D.S. (2018) Fractional crystallization of the lunar magma ocean: Updating the dominant paradigm. Meteoritics and Planetary Science 53, 1432–1455.
and Charlier et al. (2018)Charlier, B., Grove, T.L., Namur, O., Holtz, F. (2018) Crystallization of the lunar magma ocean and the primordial mantle-crust differentiation of the Moon. Geochimica et Cosmochimica Acta 234, 50–69.
. In contrast, if the initial LMO contained >500–1800 μg/g water as suggested by the experimental LMO solidification study of Lin et al. (2017a)Lin, Y.H., Tronche, E.J., Steenstra, E.S., van Westrenen, W. (2017a) Evidence for an early wet Moon from experimental crystallization of the lunar magma ocean. Nature Geoscience 10, 14–18.
the minimum amount of water in the residual LMO at the time of lunar plagioclase formation would have exceeded 1 wt. %, far exceeding the ~100 μg/g estimated using the plagioclase hygrometer in this study. In this case, the early Moon experienced extensive degassing, with >99 % of the initial LMO water budget lost during LMO crystallisation. Such a high degree of degassing is consistent with observations based on the isotopic compositions of hydrogen of lunar plagioclase (Hui et al., 2017Hui H., Guan, Y., Chen, Y., Peslier, A.H., Zhang, Y., Liu, Y., Flemming, R.L., Rossman, G.R., Eiler, J.M., Neal, C.R., Osinski, G.R. (2017) A heterogeneous lunar interior for hydrogen isotopes as revealed by the lunar highlands samples. Earth and Planetary Science Letters 473, 14–23.
) and of chlorine in lunar apatites (Sharp et al., 2010Sharp, Z.D., Shearer, C.K., McKeegan, K.D., Barnes, J.D., Wang, Y.Q. (2010) The chlorine isotope composition of the Moon and implications for an anhydrous mantle. Science 329, 1050–1053.
) if degassing of Cl occurred primarily through metal chlorides (e.g., Schaefer and Fegley, 2004Schaefer, L., Fegley, B. (2004) A thermodynamic model of high temperature lava vaporization on Io. Icarus 169, 216–241.
; Sarafian et al., 2017Sarafian, A.R., John, T., Roszjar, J., Whitehouse, M.J. (2017) Chlorine and hydrogen degassing in Vesta’s magma ocean. Earth and Planetary Science Letters 459, 311–319.
), and would point to a highly dynamic volatile cycle during early lunar evolution.top
Acknowledgements
We dedicate this manuscript to the memory of Erik Hauri. We thank Fei Peng and Wei Chen for technical assistance during electron microprobe analyses and experiments. Dr. Francis McCubbin and Dr. Adam Sarafian are thanked for their constructive reviews, and Dr. Horst R. Marschall is thanked for the editorial handling. This work was supported financially by a Netherlands Organization for Scientific Research (N.W.O.) Vici grant and N.W.O. User Support Programme Space Research grant to WvW, National Natural Science Foundation of China grants (41573055 and 41590623) to HH, Royal Netherlands Academy of Arts and Sciences China Exchange Programme grant (530-6CDP20) to WvW, HH, and YHL, National Natural Science Foundation of China grant (U1530402) to HKM, and partially supported by the Key Research Program of the Chinese Academy of Sciences, Grant NO. XDPB11, Strategic Priority Research Program (B) of the Chinese Academy of Sciences (XDB18020301) to YL, and Key Research Program of Frontier Sciences (QYZDJ-SSW-DQC012) of the CAS to XX.
Editor: Horst R. Marschall
top
Author Contributions
YHL, HH, and WvW. designed this project. YHL performed the experiments. YJX, YHL, and HH performed the FTIR analyses. YHL wrote the paper with input from all co-authors.
top
References
Caseres, J.R., Mosenfelder, J.L., Hirschmann, M.M. (2017) Partitioning of hydrogen and fluorine between feldspar and melt under the conditions of lunar crust formation. Lunar and Planetary Science Conference 48, 2303.

Table 1 gives FTIR-derived H2O concentrations in plagioclase and glass from our work, Hamada et al. (2013) and Caseres et al. (2017).
View in article
The water partition coefficients reported by Caseres et al. (2017; Dplag-meltwater = 0.018–0.023 at the IW buffer) overlap with our results.
View in article
Table 1
View in article
Pressure and temperature effects cannot be assessed on the basis of our experiments, and those of Hamada et al. (2013) and Caseres et al. (2017), due to the overall limited pressure (0.3–0.8 GPa) and temperature (1000–1230 ºC) range.
View in article
The H solubility in Fe-poor plagioclase near the IW buffer was demonstrated to be more than twice that determined at more oxidising conditions (Yang, 2012), leading to the hypothesis that oxygen fugacity could cause the difference between data obtained at lunar conditions, including our data and the data of Caseres et al. (2017), and those obtained at terrestrial conditions, i.e. the data of Hamada et al. (2013).
View in article
Figure 3 Partition coefficients of water between plagioclase and melt from this study and literature data (Hamada et al., 2013; Caseres et al., 2017), plotted versus (a) oxygen fugacity, and (b) water concentration in silicate melt.
View in article
Charlier, B., Grove, T.L., Namur, O., Holtz, F. (2018) Crystallization of the lunar magma ocean and the primordial mantle-crust differentiation of the Moon. Geochimica et Cosmochimica Acta 234, 50–69.

Sample-based inferences about water in the Moon have been complemented by experimental and modelling studies of lunar magma ocean (LMO) crystallisation (Elkins-Tanton and Grove, 2011; Lin et al., 2017a,b; Charlier et al., 2018; Rapp and Draper, 2018).
View in article
In addition, plagioclase could have formed continuously from ~70 % all the way up to >99 % of LMO crystallisation (Lin et al., 2017a; Charlier et al., 2018; Rapp and Draper, 2018).
View in article
If the LMO hydrogen budget remained constant throughout LMO solidification, this implies a very low initial LMO water content of just 5 μg/g H2O equivalent, consistent with inferences from petrology and magma ocean modelling (Elkins-Tanton and Grove, 2011), lunar sample measurements (McCubbin et al., 2015b), and the experimental LMO solidification studies of Rapp and Draper (2018) and Charlier et al. (2018).
View in article
Dixon, J.R., Papike, J.J. (1975) Petrology of anorthosites from the Descartes region of the Moon: Apollo 16. Lunar and Planetary Science Conference 6, 263–291.

Potassium, however, has very low concentrations in ferroan anorthositic plagioclase, <0.03 wt. % (Dixon and Papike, 1975) and is absent in our experiments.
View in article
We constrained the degree of crystallisation of the LMO when this plagioclase was formed by comparing the Mg# (molar (MgO/MgO + FeO) x 100) of plagioclase from sample 60015 (Mg# of 17–47) (Dixon and Papike, 1975) to the Mg# of plagioclase formed at different stages from our recent experimental study of LMO crystallisation (Lin et al., 2017a,b).
View in article
Elkins-Tanton, L.T., Grove, T.L. (2011) Water (hydrogen) in the lunar mantle: Results from petrology and magma ocean modeling. Earth and Planetary Science Letters 307, 173–179.

Sample-based inferences about water in the Moon have been complemented by experimental and modelling studies of lunar magma ocean (LMO) crystallisation (Elkins-Tanton and Grove, 2011; Lin et al., 2017a,b; Charlier et al., 2018; Rapp and Draper, 2018).
View in article
If the LMO hydrogen budget remained constant throughout LMO solidification, this implies a very low initial LMO water content of just 5 μg/g H2O equivalent, consistent with inferences from petrology and magma ocean modelling (Elkins-Tanton and Grove, 2011), lunar sample measurements (McCubbin et al., 2015b), and the experimental LMO solidification studies of Rapp and Draper (2018) and Charlier et al. (2018).
View in article
Hamada, M., Ushioda, M., Fujii, T., Takahashi, E. (2013) Hydrogen concentration in plagioclase as a hygrometer of arc basaltic melts: Approaches from melt inclusion analyses and hydrous melting experiments. Earth and Planetary Science Letters 365, 253–262.

Literature values for Dplag-meltwater range between 0.002 ± 0.0004 and 0.006 ± 0.0009 (recalculated using the plagioclase absorption coefficient determined by Mosenfelder et al. (2015) based on measurements carried out in both natural and experimental systems, which have focused solely on magmatism on Earth (e.g., Hamada et al., 2013).
View in article
Table 1 gives FTIR-derived H2O concentrations in plagioclase and glass from our work, Hamada et al. (2013) and Caseres et al. (2017).
View in article
This lowest value is at the lower end of the range of previously published partition coefficients (Dplag-meltwater = 0.002–0.006) by Hamada et al. (2013).
View in article
Our highest partition coefficient is ~7–20 times higher than values from the Hamada et al. (2013) data set.
View in article
Table 1
View in article
Pressure and temperature effects cannot be assessed on the basis of our experiments, and those of Hamada et al. (2013) and Caseres et al. (2017), due to the overall limited pressure (0.3–0.8 GPa) and temperature (1000–1230 ºC) range.
View in article
The H solubility in Fe-poor plagioclase near the IW buffer was demonstrated to be more than twice that determined at more oxidising conditions (Yang, 2012), leading to the hypothesis that oxygen fugacity could cause the difference between data obtained at lunar conditions, including our data and the data of Caseres et al. (2017), and those obtained at terrestrial conditions, i.e. the data of Hamada et al. (2013).
View in article
Previous work has suggested that changes in the OH site in plagioclases occur as a function of plagioclase OH content (Hamada et al., 2013), but we cannot identify variations in the shape of the FTIR spectra that would be consistent with such a change in our experiments.
View in article
Figure 3 Partition coefficients of water between plagioclase and melt from this study and literature data (Hamada et al., 2013; Caseres et al., 2017), plotted versus (a) oxygen fugacity, and (b) water concentration in silicate melt.
View in article
Figure 3 [...] Melt inclusion data (Hamada et al., 2013) in the dotted box are not used in this study because the formation temperature of these inclusions and the degree of water loss from inclusions after formation are uncertain.
View in article
Hauri, E.H., Weinreich, T., Saal, A.E., Rutherford, M.C., van Orman, J.A. (2011) High pre-eruptive water contents preserved in lunar melt inclusions. Science 213, 10–13.

The canonical view of a dry lunar interior has been challenged by detections of hydrogen (H or OH, reported here as equivalent amounts of H2O in μg/g) in picritic glass beads (Saal et al., 2008), apatites (e.g., McCubbin et al., 2010; Lin and van Westrenen, 2019), olivine-hosted melt inclusions (e.g., Hauri et al., 2011) and plagioclases (Hui et al., 2013).
View in article
Hui, H., Peslier, A.H., Zhang, Y., Neal, C.R. (2013) Water in lunar anorthosites and evidence for a wet early moon. Nature Geoscience 6, 177–180.

The canonical view of a dry lunar interior has been challenged by detections of hydrogen (H or OH, reported here as equivalent amounts of H2O in μg/g) in picritic glass beads (Saal et al., 2008), apatites (e.g., McCubbin et al., 2010; Lin and van Westrenen, 2019), olivine-hosted melt inclusions (e.g., Hauri et al., 2011) and plagioclases (Hui et al., 2013).
View in article
This indicates that plagioclase in lunar ferroan anorthosite could be our best candidate for estimating the water content of the LMO (Hui et al., 2013, 2017).
View in article
Only very few studies have measured water contents of lunar feldspars from a primary crystallisation product of the LMO so far (Hui et al., 2013; 2017).
View in article
Hui H., Guan, Y., Chen, Y., Peslier, A.H., Zhang, Y., Liu, Y., Flemming, R.L., Rossman, G.R., Eiler, J.M., Neal, C.R., Osinski, G.R. (2017) A heterogeneous lunar interior for hydrogen isotopes as revealed by the lunar highlands samples. Earth and Planetary Science Letters 473, 14–23.

This indicates that plagioclase in lunar ferroan anorthosite could be our best candidate for estimating the water content of the LMO (Hui et al., 2013, 2017).
View in article
Only very few studies have measured water contents of lunar feldspars from a primary crystallisation product of the LMO so far (Hui et al., 2013; 2017).
View in article
The latest study published to date on the water content of lunar plagioclase from a primary crystallisation product of the LMO reported ~5 μg/g water (H2O) in ferroan anorthosite samples including Apollo sample 60015 (Hui et al., 2017).
View in article
Such a high degree of degassing is consistent with observations based on the isotopic compositions of hydrogen of lunar plagioclase (Hui et al., 2017) and of chlorine in lunar apatites (Sharp et al., 2010) if degassing of Cl occurred primarily through metal chlorides (e.g., Schaefer and Fegley, 2004; Sarafian et al., 2017), and would point to a highly dynamic volatile cycle during early lunar evolution.
View in article
Johnson, E.A. (2006) Water in nominally anhydrous crustal minerals: speciation, concentration, and geologic significance. Reviews in Mineralogy and Geochemistry 62, 117–154.

In magmatic feldspars, concentrations from less than a few to more than 1000 μg/g H2O have been reported (Johnson and Rossman, 2003, 2004; Johnson, 2006; Mosenfelder et al., 2015).
View in article
Johnson, E.A., Rossman, G.R. (2003) The concentration and speciation of hydrogen in feldspars using FTIR and 1H MAS NMR spectroscopy. American Mineralogist 88, 901–911.

In magmatic feldspars, concentrations from less than a few to more than 1000 μg/g H2O have been reported (Johnson and Rossman, 2003, 2004; Johnson, 2006; Mosenfelder et al., 2015).
View in article
Johnson, E.A., Rossman, G.R. (2004) A survey of hydrous species and concentrations in igneous feldspars. American Mineralogist 89, 586–600.

In magmatic feldspars, concentrations from less than a few to more than 1000 μg/g H2O have been reported (Johnson and Rossman, 2003, 2004; Johnson, 2006; Mosenfelder et al., 2015).
View in article
All plagioclases show absorption bands (~3000–3600 cm-1) in the mid-infrared region typical of O–H bonds (Johnson and Rossman, 2004).
View in article
Johnson, E.A., Rossman, G.R. (2013) The diffusion behavior of hydrogen in plagioclase feldspar at 800-1000°C: Implications for re-equilibration of OH in volcanic phenocrysts. American Mineralogist 98, 1779–1787.

Previous work on water solubility in feldspar has shown that there is no obvious compositional dependence on the incorporation of H in the feldspar group except for a possible link with potassium content or sodium-hydrogen diffusion during heating (Yang, 2012; Johnson and Rossman, 2013).
View in article
Lin, Y.H., Tronche, E.J., Steenstra, E.S., van Westrenen, W. (2017a) Evidence for an early wet Moon from experimental crystallization of the lunar magma ocean. Nature Geoscience 10, 14–18.

Sample-based inferences about water in the Moon have been complemented by experimental and modelling studies of lunar magma ocean (LMO) crystallisation (Elkins-Tanton and Grove, 2011; Lin et al., 2017a,b; Charlier et al., 2018; Rapp and Draper, 2018).
View in article
In addition, plagioclase could have formed continuously from ~70 % all the way up to >99 % of LMO crystallisation (Lin et al., 2017a; Charlier et al., 2018; Rapp and Draper, 2018).
View in article
The pressures (0.4–0.6 GPa) and temperatures (1130–1220 °C) were chosen to be consistent with plagioclase formation during crystallisation of a water-bearing lunar magma ocean (Lin et al., 2017a).
View in article
We constrained the degree of crystallisation of the LMO when this plagioclase was formed by comparing the Mg# (molar (MgO/MgO + FeO) x 100) of plagioclase from sample 60015 (Mg# of 17–47) (Dixon and Papike, 1975) to the Mg# of plagioclase formed at different stages from our recent experimental study of LMO crystallisation (Lin et al., 2017a,b).
View in article
In contrast, if the initial LMO contained >500–1800 μg/g water as suggested by the experimental LMO solidification study of Lin et al. (2017a) the minimum amount of water in the residual LMO at the time of lunar plagioclase formation would have exceeded 1 wt. %, far exceeding the ~100 μg/g estimated using the plagioclase hygrometer in this study.
View in article
Lin, Y.H., Tronche, E.J., Steenstra, E.S., van Westrenen, W. (2017b) Experimental constraints on the solidification of a nominally dry lunar magma ocean. Earth and Planetary Science Letters 471, 104–116.

Sample-based inferences about water in the Moon have been complemented by experimental and modelling studies of lunar magma ocean (LMO) crystallisation (Elkins-Tanton and Grove, 2011; Lin et al., 2017a,b; Charlier et al., 2018; Rapp and Draper, 2018).
View in article
We constrained the degree of crystallisation of the LMO when this plagioclase was formed by comparing the Mg# (molar (MgO/MgO + FeO) x 100) of plagioclase from sample 60015 (Mg# of 17–47) (Dixon and Papike, 1975) to the Mg# of plagioclase formed at different stages from our recent experimental study of LMO crystallisation (Lin et al., 2017a,b).
View in article
Lin, Y.H., van Westrenen, W. (2019) Isotopic evidence for volatile replenishment of the Moon during Late Accretion. National Science Review, doi: 10.1093/nsr/nwz033.

The canonical view of a dry lunar interior has been challenged by detections of hydrogen (H or OH, reported here as equivalent amounts of H2O in μg/g) in picritic glass beads (Saal et al., 2008), apatites (e.g., McCubbin et al., 2010; Lin and van Westrenen, 2019), olivine-hosted melt inclusions (e.g., Hauri et al., 2011) and plagioclases (Hui et al., 2013).
View in article
McCubbin, F.M., Steele, A., Hauri, E.H., Nekvasil, H., Yamashita, S., Hemley, R.J. (2010) Nominally hydrous magmatism on the Moon. Proceedings of the National Academy of Sciences of the United States of America 107, 11223–11228.

The canonical view of a dry lunar interior has been challenged by detections of hydrogen (H or OH, reported here as equivalent amounts of H2O in μg/g) in picritic glass beads (Saal et al., 2008), apatites (e.g., McCubbin et al., 2010; Lin and van Westrenen, 2019), olivine-hosted melt inclusions (e.g., Hauri et al., 2011) and plagioclases (Hui et al., 2013).
View in article
McCubbin, F.M., Vander Kaaden, K.E., Tartèse, R., Boyce, J.W., Mikhail, S., Whitson, E.S., Bell, A.S., Anand, M., Franchi, I.A., Wang, J., Hauri, E.H. (2015a) Experimental investigation of F, Cl, and OH partitioning between apatite and Fe-rich basaltic melt at 1.0–1.2 GPa and 950–1000 ºC. American Mineralogist 100, 1790–1802.

However, converting hydrogen abundance data measured in lunar samples or estimated from laboratory experiments to models of the temporal and spatial evolution of water in the Moon, is far from straightforward (McCubbin et al., 2015a).
View in article
McCubbin, F.M., Vander Kaaden, K.E., Tartèse, R., Klima, R.L., Liu, Y., Mortimer, J., Barnes, J., Shearer, C.K., Treiman, A.H., Lawrence, D.J., Elardo, S.M., Hurley, D.M., Boyce, J.W., Anand, M. (2015b) Magmatic volatiles (H, C, N, F, S, Cl) in the lunar mantle, crust, and regolith: Abundances, distributions, processes, and reservoirs. American Mineralogist 100, 1668–1707.

If the LMO hydrogen budget remained constant throughout LMO solidification, this implies a very low initial LMO water content of just 5 μg/g H2O equivalent, consistent with inferences from petrology and magma ocean modelling (Elkins-Tanton and Grove, 2011), lunar sample measurements (McCubbin et al., 2015b), and the experimental LMO solidification studies of Rapp and Draper (2018) and Charlier et al. (2018).
View in article
Mosenfelder, J.L., Rossman, G.R., Johnson, E.A. (2015) Hydrous species in feldspars: A reassessment based on FTIR and SIMS. American Mineralogist 100, 1209–1221.

In magmatic feldspars, concentrations from less than a few to more than 1000 μg/g H2O have been reported (Johnson and Rossman, 2003, 2004; Johnson, 2006; Mosenfelder et al., 2015).
View in article
Literature values for Dplag-meltwater range between 0.002 ± 0.0004 and 0.006 ± 0.0009 (recalculated using the plagioclase absorption coefficient determined by Mosenfelder et al. (2015) based on measurements carried out in both natural and experimental systems, which have focused solely on magmatism on Earth (e.g., Hamada et al., 2013).
View in article
Table 1
View in article
Mosenfelder, J.L., Andrys, J.L., Caseres, J.R., Hirschmann, M.M. (2019) Water in the Moon: The perspective from nominally anhydrous minerals. Lunar and Planetary Science Conference 50, 2132.

One possibility is that the number of vacancies available for hydrogen incorporation is increased at low fO2, for example due to the enhanced incorporation of divalent iron in Al sites (Mosenfelder et al., 2019).
View in article
Newcombe, M.E., Brett, A., Beckett, J.R., Baker, M.B., Newman, S., Guan, Y., Eiler, J.M., Stolper, E.M. (2017) Solubility of water in lunar basalt at low pH2O. Geochimica et Cosmochimica Acta 200, 330–352.

The dominant hydrogen-bearing species in hydrous melts at the hydrogen levels in our experiments could be OH (Stolper, 1982; Newcombe et al., 2017), but non-linear increases in the H2O/OH ratio with increasing silicate melt hydrogen content have previously been observed (Stolper, 1982).
View in article
Rapp, J.F., Draper, D.S. (2018) Fractional crystallization of the lunar magma ocean: Updating the dominant paradigm. Meteoritics and Planetary Science 53, 1432–1455.

Sample-based inferences about water in the Moon have been complemented by experimental and modelling studies of lunar magma ocean (LMO) crystallisation (Elkins-Tanton and Grove, 2011; Lin et al., 2017a,b; Charlier et al., 2018; Rapp and Draper, 2018).
View in article
In addition, plagioclase could have formed continuously from ~70 % all the way up to >99 % of LMO crystallisation (Lin et al., 2017a; Charlier et al., 2018; Rapp and Draper, 2018).
View in article
If the LMO hydrogen budget remained constant throughout LMO solidification, this implies a very low initial LMO water content of just 5 μg/g H2O equivalent, consistent with inferences from petrology and magma ocean modelling (Elkins-Tanton and Grove, 2011), lunar sample measurements (McCubbin et al., 2015b), and the experimental LMO solidification studies of Rapp and Draper (2018) and Charlier et al. (2018).
View in article
Rutherford, M.J., Papale, P. (2009) Origin of basalt fire-fountain eruptions on Earth versus the Moon. Geology 37, 219–222.

The ƒO2 in the Moon is thought to be significantly lower, at ~IW to ~IW-2 (IW: iron-wustite) (Sato et al., 1973; Rutherford and Papale, 2009) based on sample analyses.
View in article
Saal, A.E., Hauri, E.H., Cascio, M.L., Van Orman, J.A., Rutherford, M.C., Cooper, R.F. (2008) Volatile content of lunar volcanic glasses and the presence of water in the Moon’s interior. Nature 454, 192–195.

The canonical view of a dry lunar interior has been challenged by detections of hydrogen (H or OH, reported here as equivalent amounts of H2O in μg/g) in picritic glass beads (Saal et al., 2008), apatites (e.g., McCubbin et al., 2010; Lin and van Westrenen, 2019), olivine-hosted melt inclusions (e.g., Hauri et al., 2011) and plagioclases (Hui et al., 2013).
View in article
Sarafian, A.R., John, T., Roszjar, J., Whitehouse, M.J. (2017) Chlorine and hydrogen degassing in Vesta’s magma ocean. Earth and Planetary Science Letters 459, 311–319.

Such a high degree of degassing is consistent with observations based on the isotopic compositions of hydrogen of lunar plagioclase (Hui et al., 2017) and of chlorine in lunar apatites (Sharp et al., 2010) if degassing of Cl occurred primarily through metal chlorides (e.g., Schaefer and Fegley, 2004; Sarafian et al., 2017), and would point to a highly dynamic volatile cycle during early lunar evolution.
View in article
Sato, M., Hickling, N.L., McLane, J.E. (1973) Oxygen fugacity values of Apollo 12, 14, and 15 lunar samples and reduced state of lunar magmas. Proceedings of the Lunar Science Conference 1, 1061–1079.

The ƒO2 in the Moon is thought to be significantly lower, at ~IW to ~IW-2 (IW: iron-wustite) (Sato et al., 1973; Rutherford and Papale, 2009) based on sample analyses.
View in article
Schaefer, L., Fegley, B. (2004) A thermodynamic model of high temperature lava vaporization on Io. Icarus 169, 216–241.

Such a high degree of degassing is consistent with observations based on the isotopic compositions of hydrogen of lunar plagioclase (Hui et al., 2017) and of chlorine in lunar apatites (Sharp et al., 2010) if degassing of Cl occurred primarily through metal chlorides (e.g., Schaefer and Fegley, 2004; Sarafian et al., 2017), and would point to a highly dynamic volatile cycle during early lunar evolution.
View in article
Sharp, Z.D., Shearer, C.K., McKeegan, K.D., Barnes, J.D., Wang, Y.Q. (2010) The chlorine isotope composition of the Moon and implications for an anhydrous mantle. Science 329, 1050–1053.

Such a high degree of degassing is consistent with observations based on the isotopic compositions of hydrogen of lunar plagioclase (Hui et al., 2017) and of chlorine in lunar apatites (Sharp et al., 2010) if degassing of Cl occurred primarily through metal chlorides (e.g., Schaefer and Fegley, 2004; Sarafian et al., 2017), and would point to a highly dynamic volatile cycle during early lunar evolution.
View in article
Stolper, E. (1982) Water in silicate glasses: An infrared spectroscopic study. Contributions to Mineralogy and Petrology 81, 1–17.

The dominant hydrogen-bearing species in hydrous melts at the hydrogen levels in our experiments could be OH (Stolper, 1982; Newcombe et al., 2017), but non-linear increases in the H2O/OH ratio with increasing silicate melt hydrogen content have previously been observed (Stolper, 1982).
View in article
Warren, P.H. (1985) The magma ocean concept and lunar evolution. Annual Review of Earth and Planetary Sciences 13, 201–240.

Plagioclase is thought to have crystallised and floated to the surface during the late stages of LMO crystallisation, forming the lunar primary feldspathic crust (Warren, 1985).
View in article
Yang, X. (2012) An experimental study of H solubility in feldspars: Effect of composition, oxygen fugacity, temperature and pressure and implications for crustal processes. Geochimica et Cosmochimica Acta 97, 46–57.

This is problematic, for example in terms of oxygen fugacity, as it has previously been suggested that ƒO2 can affect hydrogen solubility in plagioclase (Yang, 2012).
View in article
In addition, although Yang (2012) suggests plagioclase composition, temperature and pressure have insignificant effects on
Dplag-meltwater , this suggestion was based on experiments conducted in a limited temperature-pressure range.
View in article
The H2O concentrations in our plagioclase crystals are significantly below water solubility at our experimental conditions (Yang, 2012).
View in article
It has been shown that a number of parameters can affect water partitioning between nominally anhydrous minerals and silicate melts, including the presence and abundance of chemical impurities and vacancies, the possibility of substitutions with charge-balancing species, temperature, pressure, and oxygen fugacity (e.g., Yang, 2012 and references therein).
View in article
Previous work on water solubility in feldspar has shown that there is no obvious compositional dependence on the incorporation of H in the feldspar group except for a possible link with potassium content or sodium-hydrogen diffusion during heating (Yang, 2012; Johnson and Rossman, 2013).
View in article
The H solubility in Fe-poor plagioclase near the IW buffer was demonstrated to be more than twice that determined at more oxidising conditions (Yang, 2012), leading to the hypothesis that oxygen fugacity could cause the difference between data obtained at lunar conditions, including our data and the data of Caseres et al. (2017), and those obtained at terrestrial conditions, i.e. the data of Hamada et al. (2013).
View in article
top
Supplementary Information
The Supplementary Information includes:
- 1. Experimental and Analytical Methods
- 2. Accuracy of Partition Coefficients
- 3. Oxygen Fugacity Calculation
- Tables S-1 and S-2
- Figures S-1 and S-2
- Supplementary Information References
Download the Supplementary Information (PDF)
Figures and Tables

Figure 1 Backscattered electron (BSE) images of representative experimental run products (Pl2_LBS7H, 0.4 GPa – 1200 °С; and 2018_Pl3_LBS7H, 0.3 GPa – 1160 °С). Px = pyroxene; Plag = plagioclase.

Figure 2 Representative unpolarised infrared spectra of plagioclase, normalised to 1 cm thickness. Spectra are shifted vertically to facilitate comparison.
Table 1 Summary of experimental conditions and water contents of run products in our experiments and literature data.
Sample | Conditions | Plagioclase | Glass | Water partition coefficient | Oxygen fugacity | ||||||||
P (GPa) | T °С | Duration (h) | OH (μg/g H2O) | n | 1 s.d. | OH (wt.% H2O) | n | 1 s.d. | Dplag-melt | 1 s.d. | Oxygen buffer | Log (ƒO2) | |
This study | |||||||||||||
Pl1_LBS6H | 0.4 | 1160 | 14 | 58.2 | 11 | 17.9 | 1.03 | 9 | 0.16 | 0.006 | 0.0017 | Graphite–COH (C–COH) | -10.4 |
Pl2_LBS7H | 1200 | 16 | 63.2 | 9 | 32.8 | 0.35 | 10 | 0.02 | 0.018 | 0.0092 | -10.0 | ||
Pl3_LBS8H_1 | 1160 | 22 | 96.0 | 4 | 28.8 | 0.24 | 12 | 0.09 | 0.040 | 0.0120 | -10.4 | ||
Pl4_LBS8H_2 | 1180 | 14 | 85.5 | 5 | 33.8 | 0.63 | 8 | 0.04 | 0.014 | 0.0054 | -10.2 | ||
Pl5_LBS8H_3 | 1180 | 18 | 99.1 | 6 | 36.4 | 0.32 | 10 | 0.01 | 0.030 | 0.0112 | -10.2 | ||
2018_Pl2_LBS8H | 1170 | 24 | 73.4 | 7 | 17.0 | 0.22 | 8 | 0.02 | 0.034 | 0.0083 | -10.3 | ||
2018_Pl17_LBS8H | 1180 | 24 | 42.2 | 8 | 6.64 | 1.74 | 9 | 0.01 | 0.002 | 0.0004 | -10.2 | ||
2018_Pl21_LBS5H | 1190 | 24 | 54.2 | 6 | 14.2 | 0.13 | 8 | 0.01 | 0.043 | 0.0116 | -10.1 | ||
2018_Pl3_LBS7H | 0.3 | 1160 | 24 | 61.1 | 7 | 12.6 | 0.13 | 11 | 0.004 | 0.046 | 0.0096 | Iron-Wustite (IW) | -12.4 |
Caseres et al. (2017) Caseres, J.R., Mosenfelder, J.L., Hirschmann, M.M. (2017) Partitioning of hydrogen and fluorine between feldspar and melt under the conditions of lunar crust formation. Lunar and Planetary Science Conference 48, 2303. | |||||||||||||
1# | 0.8 | 1150 | 82 | 11 | 0.36 | 0.003 | 0.023 | 0.003 | Iron-Wustite (IW) | -12.3 | |||
2# | 118 | 6 | 0.65 | 0.008 | 0.018 | 0.001 | -12.3 | ||||||
Hamada et al. (2013) Hamada, M., Ushioda, M., Fujii, T., Takahashi, E. (2013) Hydrogen concentration in plagioclase as a hygrometer of arc basaltic melts: Approaches from melt inclusion analyses and hydrous melting experiments. Earth and Planetary Science Letters 365, 253–262. | |||||||||||||
MTL04 | 0.35 | 1130 | 24 | 89.8 | 13.5 | 3.70 | 0.56 | 0.002 | 0.0004 | > Ni–NiO (NNO) | |||
MTL05 | 1170 | 24 | 80.8 | 12.1 | 2.50 | 0.38 | 0.003 | 0.0005 | |||||
MTL17 | 1220 | 24 | 35.9 | 5.4 | 0.90 | 0.14 | 0.004 | 0.0006 | |||||
MTL22 | 1130 | 24 | 36.0 | 5.4 | 2.30 | 0.35 | 0.002 | 0.0002 | -4.7 | ||||
MTL26 | 1160 | 24 | 30.1 | 4.5 | 0.70 | 0.11 | 0.004 | 0.0006 | |||||
MTL29 | 1170 | 24 | 45.4 | 6.8 | 0.90 | 0.14 | 0.005 | 0.0008 | -5.2 | ||||
MTL33 | 1230 | 24 | 44.4 | 6.7 | 1.00 | 0.15 | 0.004 | 0.0007 | -5.8 | ||||
MTL37 | 1070 | 24 | 111 | 16.6 | 4.70 | 0.71 | 0.002 | 0.0004 | -5.2 | ||||
MTL39 | 1100 | 24 | 82.9 | 12.4 | 3.50 | 0.53 | 0.002 | 0.0004 | |||||
MTL40 | 1100 | 24 | 121 | 18.2 | 4.60 | 0.69 | 0.003 | 0.0004 | -4.7 | ||||
MTL41 | 1050 | 24 | 119 | 17.8 | 6.00 | 0.90 | 0.002 | 0.0003 | |||||
Melt Inclusion | |||||||||||||
Pl19-MI | 15.3 | 2.3 | 0.32 | 0.05 | 0.005 | 0.0007 | Fe2SiO4–Fe3O4–SiO2 (FMQ) | ||||||
Pl21-MI | 10.6 | 1.6 | 0.24 | 0.04 | 0.004 | 0.0007 | |||||||
Pl22-MI | 16.4 | 2.5 | 0.26 | 0.04 | 0.006 | 0.0009 |
Note: n, number of analysed plagioclases; s.d., 1 sigma standard deviation; Log ƒO2(buffer) corrected in the Supplementary Information;
Melt inclusion data not used in this study because we do not know exact T, P, and whether there was any water loss from the inclusions after formation;
The latest infrared absorption coefficient (Mosenfelder et al., 2015Mosenfelder, J.L., Rossman, G.R., Johnson, E.A. (2015) Hydrous species in feldspars: A reassessment based on FTIR and SIMS. American Mineralogist 100, 1209–1221.) was used for calibrating water contents of all plagioclases.

Figure 3 Partition coefficients of water between plagioclase and melt from this study and literature data (Hamada et al., 2013
Hamada, M., Ushioda, M., Fujii, T., Takahashi, E. (2013) Hydrogen concentration in plagioclase as a hygrometer of arc basaltic melts: Approaches from melt inclusion analyses and hydrous melting experiments. Earth and Planetary Science Letters 365, 253–262.
; Caseres et al., 2017Caseres, J.R., Mosenfelder, J.L., Hirschmann, M.M. (2017) Partitioning of hydrogen and fluorine between feldspar and melt under the conditions of lunar crust formation. Lunar and Planetary Science Conference 48, 2303.
), plotted versus (a) oxygen fugacity, and (b) water concentration in silicate melt. Melt inclusion data (Hamada et al., 2013Hamada, M., Ushioda, M., Fujii, T., Takahashi, E. (2013) Hydrogen concentration in plagioclase as a hygrometer of arc basaltic melts: Approaches from melt inclusion analyses and hydrous melting experiments. Earth and Planetary Science Letters 365, 253–262.
) in the dotted box are not used in this study because the formation temperature of these inclusions and the degree of water loss from inclusions after formation are uncertain.