Formation of Archean continental crust constrained by boron isotopes
Affiliations | Corresponding Author | Cite as | Funding informationSmit, M.A., Scherstén, A., Næraa, T., Emo, R.B., Scherer, E.E., Sprung, P., Bleeker, W., Mezger, K., Maltese, A., Cai, Y., Rasbury, E.T., Whitehouse, M.J. (2019) Formation of Archean continental crust constrained by boron isotopes. Geochem. Persp. Let. 12, 23–26.
NSERC (Discovery Grant RGPIN-2015-04080 to Smit), CFI and BCKDF (Grant 229814 to Smit), NSF (MRI Grant EAR-0959524 to Rsbury), SNF (Grant 17452 to Mezger and Maltese), VR (Grant 2014-06375 to Whitehouse)
- Share this article
Article views:3,274Cumulative count of HTML views and PDF downloads.
- Download Citation
- Rights & Permissions
top
Abstract

Figures and Tables
![]() Figure 1 Trace element concentrations for the TTG samples and average TTG (Moyen and Martin, 2012). All data are normalised to primitive mantle (PM; data in Table S-7). | ![]() Figure 2 (a) B and (b) δ11B versus Zr/Hf with the correlation coefficients for the given regressions. | ![]() Figure 3 Boron isotope data for the analysed samples and terrestrial reservoirs (histograms). Error bars for whole rock analyses are smaller than the symbols. Acasta data represents bulk rock δ11B modelled from biotite data (Supplementary Information, Analytical Methods and Data). The values for reference reservoirs are in Table S-1. | ![]() Figure 4 Cathodoluminescence images of zircon from the Acasta Gneiss Complex samples showing analysed biotite inclusions. The δ11B values of biotite (in ‰) and the 207Pb/206Pb age of the surrounding zircon (minimum inclusion age) are shown. Analytical spots are to scale; asterisk marks >10 % discordance; scale bars are 50 μm. Data are in Table S-9. |
Figure 1 | Figure 2 | Figure 3 | Figure 4 |
top
Introduction
Plate tectonics is a global process in which partially hydrated and dense oceanic lithosphere is recycled in long lived subduction zones. Although prevalent throughout the Phanerozoic, it is unclear whether, or to what extent, this process operated during the Archean (4.0-2.5 Ga) when mantle heat flow was higher, and the lithosphere was perhaps less differentiated (Brown, 2014
Brown, M. (2014) The contribution of metamorphic petrology to understanding lithosphere evolution and geodynamics. Geoscience Frontiers 5, 553-569.
; Kamber, 2015Kamber, B.S. (2015) The evolving nature of terrestrial crust from the Hadean, through the Archaean, into the Proterozoic. Precambrian Research 258, 48-82.
). Critical to this debate is the interpretation of tonalite-trondhjemite-granodiorite (TTG) complexes, which make up 90 % of the preserved Archean juvenile continental crust (Martin et al., 2005Martin, H., Smithies, R.H., Rapp, R.P., Moyen, J.-F., Champion, D. (2005) An overview of adakite, tonalite trondhjemite granodiorite (TTG), and sanukitoid: relationships and some implications for crustal evolution. Lithos 79, 1-24.
; Moyen and Martin, 2012Moyen, J.-F., Martin, H. (2012) Forty years of TTG research. Lithos 148, 312-336.
). These low-K, high-GdN/YbN, I-type granitoids reflect melting of hydrous basaltic sources with residual hornblende and/or garnet (e.g., Arth and Hanson, 1975Arth, J.G., Hanson, G.N. (1975) Geochemistry and origin of the early Precambrian crust of northeastern Minnesota. Geochimica et Cosmochimica Acta 39, 325-362.
; Rapp et al., 1991Rapp, R.P., Watson, E.B., Miller, C. (1991) Partial melting of amphibolite/eclogite and the origin of Archean trondhjemites and tonalities. Precambrian Research 51, 1-25.
). Interpretations regarding the geodynamic setting of TTG formation are diverse and numerous (Moyen and Martin, 2012Moyen, J.-F., Martin, H. (2012) Forty years of TTG research. Lithos 148, 312-336.
). Several of these consider TTGs as slab melts from primitive flat slab subduction zones with a high geothermal gradient and no contribution from the mantle wedge (Smithies, 2000Smithies, R.H. (2000) The Archaean tonalite–trondhjemite–granodiorite (TTG) series is not an analogue of Cenozoic adakite. Earth and Planetary Science Letters 182, 115-125.
; Laurent et al., 2014Laurent, O., Martin, H., Moyen, J.-F., Doucelance, R. (2014) The diversity and evolution of late-Archean granitoids: Evidence for the onset of “modern-style” plate tectonics between 3.0 and 2.5 Ga. Lithos 205, 208-235.
). Others models suggest that TTGs represent melts from thickened mafic crust produced during episodes of density-driven crustal overturning, delamination, underplating or plume activity (Smithies, 2000Smithies, R.H. (2000) The Archaean tonalite–trondhjemite–granodiorite (TTG) series is not an analogue of Cenozoic adakite. Earth and Planetary Science Letters 182, 115-125.
; Bédard, 2006Bédard, J.H. (2006) A catalytic delamination-driven model for coupled genesis of Archaean crust and sub-continental lithospheric mantle. Geochimica et Cosmochimica Acta 70, 1188-1214.
; van Kranendonk et al., 2007van Kranendonk, M.J., Smithies, R.H., Hickman, A.H., Champion, D.C. (2007) Secular tectonic evolution of Archean continental crust: interplay between horizontal and vertical processes in the formation of the Pilbara Craton, Australia. Terra Nova 19, 1-38.
; Kamber, 2015Kamber, B.S. (2015) The evolving nature of terrestrial crust from the Hadean, through the Archaean, into the Proterozoic. Precambrian Research 258, 48-82.
; Johnson et al., 2017Johnson, T.E., Brown, M., Gardiner, N.J., Kirkland, C.L., Smithies, R.H. (2017) Earth’s first stable continents did not form by subduction. Nature 543, 239-242.
). Stagnant lid models (e.g., Debaille et al., 2013Debaille, V., O`Neill, C., Brandon, A.D., Naenecour, P., Yin, Q.-Z., Matielli, N., Treiman, A.H. (2013) Stagnant-lid tectonics in early Earth revealed by 142Nd variations in late Archean rocks. Earth and Planetary Science Letters 373, 83-92.
) provide hybrids to these ‘subduction’ and ‘intraplate’ models (Moyen and Martin, 2012Moyen, J.-F., Martin, H. (2012) Forty years of TTG research. Lithos 148, 312-336.
), involving plume-induced melting, as well as flux melting of the mantle during sporadic, local plate burial. Although the models differ at various levels of detail, they differ fundamentally regarding the nature of the mafic source: either it comprised deeply subducted crustal material from the Earth’s surface, or it represented the lower and never-before-surfaced sections of a thickened or underplated crust.Zircon Hf and O isotope data suggest that Archean juvenile continental crust formed by melting of enriched mafic crust extracted from a still primitive mantle, and that sediment recycling and crustal thickening occurred since the Mesoarchean (e.g., Kemp et al., 2010
Kemp, A.I.S., Wilde, S.A., Hawkesworth, C.J., Coath, C.D., Nemchin, A.A., Pidgeon, R.T., Vervoort, J.D., DuFrane, S.A. (2010) Hadean crustal evolution revisited: New constraints from Pb–Hf isotope systematics of the Jack Hills zircons. Earth and Planetary Science Letters 296, 45-56.
; Næraa et al., 2012Næraa, T., Scherstén, A., Rosing, M.T., Kemp, A.I.S., Hoffmann, J.E., Kokfelt, T.F., Whitehouse, M.J. (2012) Hafnium isotope evidence for a transition in the dynamics of continental growth 3.2 Gyr ago. Nature 485, 627-630.
; Reimink et al., 2016Reimink, J.R., Davies, J.H.F.L., Chacko, T., Stern, R.A., Heaman, L.M., Sarkar, C., Schaltegger, U., Creaser, R.A., Pearson, D.G. (2016) No evidence for Hadean continental crust within Earth’s oldest evolved rock unit. Nature Geoscience 9, 777-780.
; Roberts and Santosh, 2018Roberts, N.M.W., Santosh, M. (2018) Capturing the Mesoarchean emergence of continental Crust in the Coorg Block, Southern India. Geophysical Research Letters 45, 7444-7453.
). The same is indicated by Si-O isotope data for Archean detrital zircon (Trail et al., 2018Trail, D., Boehnke, P., Savage, P., Liu, M.-C., Miller, M.L., Bindeman, I. (2018) Origin and significance of Si and O isotope heterogeneities in Phanerozoic, Archean, and Hadean zircon. Proceedings of the National Academy of Sciences 115, 10287-10292.
). These interpretations fit the observation that high 18O/16O granitoids produced by melting of surface-altered rocks are restricted to post-Archean time when recycling of such material, possibly by subduction, became prevalent (Valley et al., 2005Valley, J.W., Lackey, J.S., Cavosie, A.J., Clechenko, C.C., Spicuzza, M.J., Basei, M.A.S., Bindeman, I.N., Ferreira, V.P., Sial, A.N., King, E.M., Peck, W.H., Sinha, A.K., Wei, C.S. (2005) 4.4 billion years of crustal maturation: oxygen isotope ratios of magmatic zircon. Contributions to Mineralogy and Petrology 150, 561-580.
). Some Archean TTGs show elevated 30Si/28Si and 18O/16O values, which were interpreted to indicate chert and, by inference, subducted sediments in the source (Deng et al., 2019Deng, Z., Chaussidon, M., Guitreau, M., Puchtel, I.S., Dauphas, N., Moynier, F. (2019) An oceanic subduction origin for Archaean granitoids revealed by silicon isotopes. Nature Geoscience 12, 774-778.
). The integral Si-O isotope record of Hadean zircon and Archean TTGs indicates source contributions from a variety of supra-crustal rocks, including chemical sediments, serpentinised and silicified basalts (Trail et al., 2018Trail, D., Boehnke, P., Savage, P., Liu, M.-C., Miller, M.L., Bindeman, I. (2018) Origin and significance of Si and O isotope heterogeneities in Phanerozoic, Archean, and Hadean zircon. Proceedings of the National Academy of Sciences 115, 10287-10292.
; André et al., 2019André, L., Abraham, K., Hofmann, A., Monin, L., Kleinhanns, I.C., Foley, S. (2019) Early continental crust generated by reworking of basalts variably silicified by seawater. Nature Geoscience 12, 769-773.
). Whether anatexis of such material requires deep burial by subduction is unclear. Moreover, Si and O may not both represent the source and its components. The elevated 18O/16O values that — together with elevated 30Si/28Si values — were taken to represent the chert component actually partly exceed those typically observed for Archean TTGs; these could reflect secondary 18O-enrichment via low temperature alteration (Valley et al., 2005Valley, J.W., Lackey, J.S., Cavosie, A.J., Clechenko, C.C., Spicuzza, M.J., Basei, M.A.S., Bindeman, I.N., Ferreira, V.P., Sial, A.N., King, E.M., Peck, W.H., Sinha, A.K., Wei, C.S. (2005) 4.4 billion years of crustal maturation: oxygen isotope ratios of magmatic zircon. Contributions to Mineralogy and Petrology 150, 561-580.
). The Sr anomalies (Sr/Sr*) of the analysed TTGs correlate strongly inversely with 18O/16O and may thus likewise be disturbed. Strikingly, the compositions of the samples with highest Sr/Sr* values match those modelled for low degree garnet-stable partial melting of a tholeiitic source that is not contaminated by chert or other components (Deng et al., 2019Deng, Z., Chaussidon, M., Guitreau, M., Puchtel, I.S., Dauphas, N., Moynier, F. (2019) An oceanic subduction origin for Archaean granitoids revealed by silicon isotopes. Nature Geoscience 12, 774-778.
). Although Si-O data provide a unique opportunity to discern supra-crustal contributions to TTG sources (Trail et al., 2018Trail, D., Boehnke, P., Savage, P., Liu, M.-C., Miller, M.L., Bindeman, I. (2018) Origin and significance of Si and O isotope heterogeneities in Phanerozoic, Archean, and Hadean zircon. Proceedings of the National Academy of Sciences 115, 10287-10292.
), their interpretation to discern the geodynamic setting of TTG formation is still controversial.Boron analysis provides an alternative approach to characterising the TTG source. The global B cycle and B isotope systematics are well characterised and appear uniform across geologic time (Supplementary Information, Introduction 1). Most importantly, seawater shows a very strong long term enrichment in the heavy isotope 11B, which is reflected in the B chemistry of subducted slabs and magmatic rocks from active margins (Ryan and Chauvel, 2014
Ryan, J.G., Chauvel, C. (2014) The subduction-zone filter and the impact of recycled materials on the evolution of the mantle. In: Holland, H.D., Turekian, K.K. (Eds.) Treatise on Geochemistry 3: The Mantle and Core. Elsevier, Amsterdam, 479-508.
; de Hoog and Savov, 2018de Hoog, J.C.M., Savov, I.P. (2018) Boron isotopes as a tracer of subduction zone processes. In: Marschall, H.R., Foster, G.L. (Eds.) Boron Isotopes – The Fifth Element, Advances in Isotope Geochemistry 6. Springer, Heidelberg, 217-247.
). The latter is because B is partitioned into melts or fluids that are expelled from the subducting slab and feed the arc source. Due to the enrichment of B and 11B in their source, most magmatic rocks from active margins — including adakites and modern I-type granitoids from continental arcs (Fig. 2) — typically exhibit higher δ11B values than the average continental crust (δ11B = -9.4 ± 0.4 ‰), or mid-oceanic ridge basalts (MORB) and the depleted MORB mantle (DMM; δ11B = -7.1 ± 0.9 ‰; Marschall et al., 2017Marschall, H.R., Wanless, V.D., Shimizu, N., Pogge von Strandmann, P.A.E., Elliott, T., Monteleone, B.D. (2017) The boron and lithium isotopic composition of mid-ocean ridge basalts and the mantle. Geochimica et Cosmochimica Acta 207, 102–138.
; Marschall, 2018Marschall, H.R. (2018) Boron isotopes in the ocean floor realm and the mantle. In: Marschall, H.R., Foster, G.L. (Eds.) Boron Isotopes – The Fifth Element, Advances in Isotope Geochemistry 6. Springer, Heidelberg, 189-215.
; δ11B = 1000 × [(11B/10B)sample/(11B/10B)SRM-951 - 1]). Only in rare situations of very steep or very hot subduction do magmatic rocks occasionally include δ11B values that are similar to, or even lower than, that of DMM. Intense dehydration in these locations cause slab B budgets to be so low that the B signature of the arc source becomes dominated by melting of the mantle wedge (e.g., de Hoog and Savov, 2018de Hoog, J.C.M., Savov, I.P. (2018) Boron isotopes as a tracer of subduction zone processes. In: Marschall, H.R., Foster, G.L. (Eds.) Boron Isotopes – The Fifth Element, Advances in Isotope Geochemistry 6. Springer, Heidelberg, 217-247.
).top
The B Signature of Archean TTGs
Boron concentrations and isotope compositions were determined for: 1) 3.78 Ga tonalite gneisses from the Isua Greenstone Belt, West Greenland; 2) ≥3.6 Ga tonalite and trondhjemite gneisses from the Acasta Gneiss; 3) 3.58 Ga tonalite gneisses from the Bastar Craton, India; 4) 2.87-2.79 Ga tonalite and trondhjemite gneisses from the Tartôq Greenstone Belt in Southwest Greenland. Whole rock materials were analysed, except for the Acasta TTG samples, which are poly-metamorphosed and exhibit altered matrices. To investigate the initial B composition of these rocks, in situ B analysis was done on pristine biotite inclusions inside igneous zircon (rationale in Supplementary Information, Introduction 2; geological descriptions in Supplementary Information, Introduction 3).
The samples show a range of trace element compositions (Table S-7) and comprise low and high GdN/YbN TTGs (Acasta, Tartôq), or high GdN/YbN TTGs only (Isua, Bastar; Fig. 1). Boron concentrations are 1.2-3.4 ppm — similar to those observed in other pristine Archean TTGs (Supplementary Information, Introduction 1). The B concentration correlates with Zr/Hf (R2 = 0.82; Fig. 2a), but not with B/Nb, B/La, B/Zr, GdN/YbN or Nb/Ta. The δ11B values of bulk TTG are -15.2 to -2.5 ‰ (Fig. 3, Table S-5). The δ11B values correlate negatively with B concentration (R2 = 0.73; Fig. 2a) and Zr/Hf (R2 = 0.75; Fig. 2b). The meta-tonalite sample 508281 shows a similar B concentration as pristine samples, yet yielded δ11B values that are at least 5 ‰ higher. The δ11B values of biotite inclusions in Acasta zircon are -5.4 ‰ or lower, indicating negative δ11B values for the protolith, even when assuming the largest biotite-melt fractionation factor (Supplementary Information, Analytical Methods and Data). The lowest values among this range were obtained from pristine zircon domains (Fig. 4a_c), whereas values above -10 ‰ were obtained for inclusions from zircon with disturbed U-Pb systematics and signs of alteration. The latter inclusions resemble biotite in the altered matrix in terms of B (Fig. 4d, Table S-5). The dispersion in biotite δ11B values for single samples may thus reflect late fluid-rock interaction. The dispersion in biotite δ11B values for single samples may thus reflect late fluid-rock interaction and the high values among the δ11B range may represent 11B enrichment from this process.

Figure 1 Trace element concentrations for the TTG samples and average TTG (Moyen and Martin, 2012
Moyen, J.-F., Martin, H. (2012) Forty years of TTG research. Lithos 148, 312-336.
). All data are normalised to primitive mantle (PM; data in Table S-7).
Figure 2 (a) B and (b) δ11B versus Zr/Hf with the correlation coefficients for the given regressions.

Figure 3 Boron isotope data for the analysed samples and terrestrial reservoirs (histograms). Error bars for whole rock analyses are smaller than the symbols. Acasta data represents bulk rock δ11B modelled from biotite data (Supplementary Information, Analytical Methods and Data). The values for reference reservoirs are in Table S-1.

Figure 4 Cathodoluminescence images of zircon from the Acasta Gneiss Complex samples showing analysed biotite inclusions. The δ11B values of biotite (in ‰) and the 207Pb/206Pb age of the surrounding zircon (minimum inclusion age) are shown. Analytical spots are to scale; asterisk marks >10 % discordance; scale bars are 50 μm. Data are in Table S-9.
top
Discussion
Changes in the Zr/Hf value of tonalitic melt typically result from the crystallisation of ferromagnesian minerals (Linnen and Keppler, 2002
Linnen, R.L., Keppler, H. (2002) Melt composition control of Zr/Hf fractionation in magmatic processes. Geochimica et Cosmochimica Acta 66, 3293-3301.
) — biotite + amphibole ± plagioclase in this case, with or without garnet (Supplementary Information, Discussion) — which likewise would enrich the melt in highly incompatible B. The effects of such magmatic differentiation are clearly reflected in the B-Zr/Hf correlation (Fig. 2a). At chondritic Zr/Hf, TTGs are predicted to have 1-2 ppm B and a δ11B value of ca. -7 ‰, which resembles melts from the DMM (1.2-1.3 ppm, -7.1 ± 0.9 ‰; Marschall et al., 2017Marschall, H.R., Wanless, V.D., Shimizu, N., Pogge von Strandmann, P.A.E., Elliott, T., Monteleone, B.D. (2017) The boron and lithium isotopic composition of mid-ocean ridge basalts and the mantle. Geochimica et Cosmochimica Acta 207, 102–138.
). The δ11B-Zr/Hf correlation may be linked to this through the role of H2O. Crystallisation of basaltic and andesitic magmas is associated with the exsolution of H2O and other volatiles (Sisson and Layne, 1993Sisson, T.W., Layne, G.D. (1993) H2O in basalt and basaltic andesite glass inclusions from four subduction-related volcanoes. Earth and Planetary Science Letters 117, 619-635.
). Aqueous fluids in equilibrium with silicate melt preferentially incorporate 11B, such that the more fractionated and hydrous magmas are, the lower δ11B values of the crystallising magmas become (Hervig et al., 2002Hervig, R.L., Moore, G.M., Williams, L.B., Peacock, S.M., Holloway, J.R., Roggensack, K., (2002) Isotopic and elemental partitioning of boron between hydrous fluid and silicate melt. American Mineralogist 87, 769-774.
). The B-Zr/Hf correlation in TTGs from different terranes and with different composition (Fig. 2) shows that their mafic sources — both garnet-present and garnet-absent—had a similar initial B-δ11B-Zr/Hf signature, and evolved without the addition of externally derived B.The low B concentrations and δ11B values, and their correlation with Zr/Hf, identify Archean TTGs as the differentiated magmatic products of a common mafic source that contained no detectable B from seawater or seawater-altered rocks. The TTGs differ from modern I-type granitoids from active margins; these are variably enriched in B and 11B, independent of the degree of crystal fractionation (de Hoog and Savov, 2018
de Hoog, J.C.M., Savov, I.P. (2018) Boron isotopes as a tracer of subduction zone processes. In: Marschall, H.R., Foster, G.L. (Eds.) Boron Isotopes – The Fifth Element, Advances in Isotope Geochemistry 6. Springer, Heidelberg, 217-247.
), and do not show fractionation down to the low δ11B values observed here (Fig. 2). As the relevant B reservoirs appear uniform through time (Supplementary Information, Introduction 1), such a signature would be expected for any Archean arc as well — possibly even more so, considering that a contribution from the mantle wedge appears lacking (Martin et al., 2005Martin, H., Smithies, R.H., Rapp, R.P., Moyen, J.-F., Champion, D. (2005) An overview of adakite, tonalite trondhjemite granodiorite (TTG), and sanukitoid: relationships and some implications for crustal evolution. Lithos 79, 1-24.
). A subduction origin for TTGs cannot be a priori excluded. However, this would require that slabs were dehydrated such that they lost all B from seawater-altered materials prior to melting. Such dehydration is not seen even in the warmest of modern arcs and appears inconsistent with the hydrous nature of the TTG source (Moyen and Martin, 2012Moyen, J.-F., Martin, H. (2012) Forty years of TTG research. Lithos 148, 312-336.
). More importantly, high geothermal gradients during the Archean would favour slab melting over slab dehydration (Martin, 1986Martin, H. (1986) Effect of steeper Archean geothermal gradient on geochemistry of subduction zone magmas. Geology 14, 753–756,
; Moyen and Martin, 2012Moyen, J.-F., Martin, H. (2012) Forty years of TTG research. Lithos 148, 312-336.
), indicating that B and H2O would have been expelled from slabs concomitantly, not sequentially. This considered, the data appear more consistent with the TTG formation via ‘intraplate’ processes (Moyen and Martin, 2012Moyen, J.-F., Martin, H. (2012) Forty years of TTG research. Lithos 148, 312-336.
) — e.g., crustal thickening, volcanic resurfacing, overturning, delamination, and stagnant lid or ‘squishy lid’ tectonics (Bédard, 2006Bédard, J.H. (2006) A catalytic delamination-driven model for coupled genesis of Archaean crust and sub-continental lithospheric mantle. Geochimica et Cosmochimica Acta 70, 1188-1214.
; van Kranendonk et al., 2007van Kranendonk, M.J., Smithies, R.H., Hickman, A.H., Champion, D.C. (2007) Secular tectonic evolution of Archean continental crust: interplay between horizontal and vertical processes in the formation of the Pilbara Craton, Australia. Terra Nova 19, 1-38.
; Hoffmann et al., 2011Hoffmann, J.E., Münker, C., Næraa, T., Rosing, M.T., Herwartz, D., Garbe-Schönberg, D., Svahnberg, S. (2011) Mechanisms of Archean crust formation inferred from high-precision HFSE systematics in TTGs. Geochimica et Cosmochimica Acta 75, 4157-4178.
; Debaille et al., 2013Debaille, V., O`Neill, C., Brandon, A.D., Naenecour, P., Yin, Q.-Z., Matielli, N., Treiman, A.H. (2013) Stagnant-lid tectonics in early Earth revealed by 142Nd variations in late Archean rocks. Earth and Planetary Science Letters 373, 83-92.
; Kamber, 2015Kamber, B.S. (2015) The evolving nature of terrestrial crust from the Hadean, through the Archaean, into the Proterozoic. Precambrian Research 258, 48-82.
; Johnson et al., 2017Johnson, T.E., Brown, M., Gardiner, N.J., Kirkland, C.L., Smithies, R.H. (2017) Earth’s first stable continents did not form by subduction. Nature 543, 239-242.
; Rozel et al., 2017Rozel, A.B., Golabek, G.J., Jain, C., Tackley, P.J., Gerya, T. (2017) Continental crust formation on early Earth controlled by intrusive magmatism. Nature 545, 332-335.
) — in which deep hydrated mafic crust with a DMM-like δ11B value melted under the influence of a high or unstable geotherm. The absence of an elevated δ11B signature from any supracrustal rocks, which Si-O data indicate were present in the source (André et al., 2019André, L., Abraham, K., Hofmann, A., Monin, L., Kleinhanns, I.C., Foley, S. (2019) Early continental crust generated by reworking of basalts variably silicified by seawater. Nature Geoscience 12, 769-773.
; Deng et al., 2019Deng, Z., Chaussidon, M., Guitreau, M., Puchtel, I.S., Dauphas, N., Moynier, F. (2019) An oceanic subduction origin for Archaean granitoids revealed by silicon isotopes. Nature Geoscience 12, 774-778.
), could signify B loss from these rocks during residence at depth on timescales that far exceed that of subduction. With subduction processes not clearly reflected in the B data, it would appear that these did not contribute substantially to Archean juvenile crustal growth before 2.8 Ga. If Archean juvenile crustal growth and the start of modern style plate tectonics are at all related, it would appear that the former was the cause, rather than the consequence, of the latter.top
Acknowledgements
We thank G.E. Harlow, C. Martin and T. Zack for providing reference materials, and K. Lindén (NRM), L. Kato and V. Lai (UBC) for technical support. The manuscript substantially benefited from constructive reviews by R. Halama and one anonymous reviewer, and by C.J. Hawkesworth, M. Brown, B. Kamber, and H.R. Marschall on a previous version. We thank M. Boyet for editorial handling. This is contribution #619 of the NordSIMS facility, a Swedish-Icelandic research infrastructure. The research was supported by NSERC (Discovery Grant RGPIN-2015-04080 to MAS), CFI and BC-KDF (Grant 229814 to MAS), NSF (MRI Grant EAR-0959524 to ETR), and VR (Grant 2014-06375). KM and AM acknowledge support through SNF (Grant 17452).
top
References
André, L., Abraham, K., Hofmann, A., Monin, L., Kleinhanns, I.C., Foley, S. (2019) Early continental crust generated by reworking of basalts variably silicified by seawater. Nature Geoscience 12, 769-773.

The integral Si-O isotope record of Hadean zircon and Archean TTGs indicates source contributions from a variety of supra-crustal rocks, including chemical sediments, serpentinised and silicified basalts (Trail et al., 2018; André et al., 2019).
View in article
The absence of an elevated δ11B signature from any supracrustal rocks, which Si-O data indicate were present in the source (André et al., 2019; Deng et al., 2019), could signify B loss from these rocks during residence at depth on timescales that far exceed that of subduction.
View in article
Arth, J.G., Hanson, G.N. (1975) Geochemistry and origin of the early Precambrian crust of northeastern Minnesota. Geochimica et Cosmochimica Acta 39, 325-362.

These low-K, high-GdN/YbN, I-type granitoids reflect melting of hydrous basaltic sources with residual hornblende and/or garnet (e.g., Arth and Hanson, 1975; Rapp et al., 1991).
View in article
Bédard, J.H. (2006) A catalytic delamination-driven model for coupled genesis of Archaean crust and sub-continental lithospheric mantle. Geochimica et Cosmochimica Acta 70, 1188-1214.

Others models suggest that TTGs represent melts from thickened mafic crust produced during episodes of density-driven crustal overturning, delamination, underplating or plume activity (Smithies, 2000; Bédard, 2006; van Kranendonk et al., 2007; Kamber, 2015; Johnson et al., 2017).
View in article
This considered, the data appear more consistent with the TTG formation via ‘intraplate’ processes (Moyen and Martin, 2012) — e.g., crustal thickening, volcanic resurfacing, overturning, delamination, and stagnant lid or ‘squishy lid’ tectonics (Bédard, 2006; van Kranendonk et al., 2007; Hoffmann et al., 2011; Debaille et al., 2013; Kamber, 2015; Johnson et al., 2017; Rozel et al., 2017) — in which deep hydrated mafic crust with a DMM-like δ11B value melted under the influence of a high or unstable geotherm.
View in article
Brown, M. (2014) The contribution of metamorphic petrology to understanding lithosphere evolution and geodynamics. Geoscience Frontiers 5, 553-569.

Although prevalent throughout the Phanerozoic, it is unclear whether, or to what extent, this process operated during the Archean (4.0-2.5 Ga) when mantle heat flow was higher, and the lithosphere was perhaps less differentiated (Brown, 2014; Kamber, 2015).
View in article
Debaille, V., O`Neill, C., Brandon, A.D., Naenecour, P., Yin, Q.-Z., Matielli, N., Treiman, A.H. (2013) Stagnant-lid tectonics in early Earth revealed by 142Nd variations in late Archean rocks. Earth and Planetary Science Letters 373, 83-92.

Stagnant lid models (e.g., Debaille et al., 2013) provide hybrids to these ‘subduction’ and ‘intraplate’ models (Moyen and Martin, 2012), involving plume-induced melting, as well as flux melting of the mantle during sporadic, local plate burial.
View in article
This considered, the data appear more consistent with the TTG formation via ‘intraplate’ processes (Moyen and Martin, 2012) — e.g., crustal thickening, volcanic resurfacing, overturning, delamination, and stagnant lid or ‘squishy lid’ tectonics (Bédard, 2006; van Kranendonk et al., 2007; Hoffmann et al., 2011; Debaille et al., 2013; Kamber, 2015; Johnson et al., 2017; Rozel et al., 2017) — in which deep hydrated mafic crust with a DMM-like δ11B value melted under the influence of a high or unstable geotherm.
View in article
de Hoog, J.C.M., Savov, I.P. (2018) Boron isotopes as a tracer of subduction zone processes. In: Marschall, H.R., Foster, G.L. (Eds.) Boron Isotopes – The Fifth Element, Advances in Isotope Geochemistry 6. Springer, Heidelberg, 217-247.

Most importantly, seawater shows a very strong long term enrichment in the heavy isotope 11B, which is reflected in the B chemistry of subducted slabs and magmatic rocks from active margins (Ryan and Chauvel, 2014; de Hoog and Savov, 2018).
View in article
Intense dehydration in these locations cause slab B budgets to be so low that the B signature of the arc source becomes dominated by melting of the mantle wedge (e.g., de Hoog and Savov, 2018).
View in article
The TTGs differ from modern I-type granitoids from active margins; these are variably enriched in B and 11B, independent of the degree of crystal fractionation (de Hoog and Savov, 2018), and do not show fractionation down to the low δ11B values observed here (Fig. 2).
View in article
Deng, Z., Chaussidon, M., Guitreau, M., Puchtel, I.S., Dauphas, N., Moynier, F. (2019) An oceanic subduction origin for Archaean granitoids revealed by silicon isotopes. Nature Geoscience 12, 774-778.

Some Archean TTGs show elevated 30Si/28Si and 18O/16O values, which were interpreted to indicate chert and, by inference, subducted sediments in the source (Deng et al., 2019).
View in article
Strikingly, the compositions of the samples with highest Sr/Sr* values match those modelled for low degree garnet-stable partial melting of a tholeiitic source that is not contaminated by chert or other components (Deng et al., 2019).
View in article
The absence of an elevated δ11B signature from any supracrustal rocks, which Si-O data indicate were present in the source (André et al., 2019; Deng et al., 2019), could signify B loss from these rocks during residence at depth on timescales that far exceed that of subduction.
View in article
Hervig, R.L., Moore, G.M., Williams, L.B., Peacock, S.M., Holloway, J.R., Roggensack, K., (2002) Isotopic and elemental partitioning of boron between hydrous fluid and silicate melt. American Mineralogist 87, 769-774.

Aqueous fluids in equilibrium with silicate melt preferentially incorporate 11B, such that the more fractionated and hydrous magmas are, the lower δ11B values of the crystallising magmas become (Hervig et al., 2002).
View in article
Hoffmann, J.E., Münker, C., Næraa, T., Rosing, M.T., Herwartz, D., Garbe-Schönberg, D., Svahnberg, S. (2011) Mechanisms of Archean crust formation inferred from high-precision HFSE systematics in TTGs. Geochimica et Cosmochimica Acta 75, 4157-4178.

This considered, the data appear more consistent with the TTG formation via ‘intraplate’ processes (Moyen and Martin, 2012) — e.g., crustal thickening, volcanic resurfacing, overturning, delamination, and stagnant lid or ‘squishy lid’ tectonics (Bédard, 2006; van Kranendonk et al., 2007; Hoffmann et al., 2011; Debaille et al., 2013; Kamber, 2015; Johnson et al., 2017; Rozel et al., 2017) — in which deep hydrated mafic crust with a DMM-like δ11B value melted under the influence of a high or unstable geotherm.
View in article
Johnson, T.E., Brown, M., Gardiner, N.J., Kirkland, C.L., Smithies, R.H. (2017) Earth’s first stable continents did not form by subduction. Nature 543, 239-242.

Others models suggest that TTGs represent melts from thickened mafic crust produced during episodes of density-driven crustal overturning, delamination, underplating or plume activity (Smithies, 2000; Bédard, 2006; van Kranendonk et al., 2007; Kamber, 2015; Johnson et al., 2017).
View in article
This considered, the data appear more consistent with the TTG formation via ‘intraplate’ processes (Moyen and Martin, 2012) — e.g., crustal thickening, volcanic resurfacing, overturning, delamination, and stagnant lid or ‘squishy lid’ tectonics (Bédard, 2006; van Kranendonk et al., 2007; Hoffmann et al., 2011; Debaille et al., 2013; Kamber, 2015; Johnson et al., 2017; Rozel et al., 2017) — in which deep hydrated mafic crust with a DMM-like δ11B value melted under the influence of a high or unstable geotherm.
View in article
Kamber, B.S. (2015) The evolving nature of terrestrial crust from the Hadean, through the Archaean, into the Proterozoic. Precambrian Research 258, 48-82.

Although prevalent throughout the Phanerozoic, it is unclear whether, or to what extent, this process operated during the Archean (4.0-2.5 Ga) when mantle heat flow was higher, and the lithosphere was perhaps less differentiated (Brown, 2014; Kamber, 2015).
View in article
Others models suggest that TTGs represent melts from thickened mafic crust produced during episodes of density-driven crustal overturning, delamination, underplating or plume activity (Smithies, 2000; Bédard, 2006; van Kranendonk et al., 2007; Kamber, 2015; Johnson et al., 2017).
View in article
This considered, the data appear more consistent with the TTG formation via ‘intraplate’ processes (Moyen and Martin, 2012) — e.g., crustal thickening, volcanic resurfacing, overturning, delamination, and stagnant lid or ‘squishy lid’ tectonics (Bédard, 2006; van Kranendonk et al., 2007; Hoffmann et al., 2011; Debaille et al., 2013; Kamber, 2015; Johnson et al., 2017; Rozel et al., 2017) — in which deep hydrated mafic crust with a DMM-like δ11B value melted under the influence of a high or unstable geotherm.
View in article
Kemp, A.I.S., Wilde, S.A., Hawkesworth, C.J., Coath, C.D., Nemchin, A.A., Pidgeon, R.T., Vervoort, J.D., DuFrane, S.A. (2010) Hadean crustal evolution revisited: New constraints from Pb–Hf isotope systematics of the Jack Hills zircons. Earth and Planetary Science Letters 296, 45-56.

Zircon Hf and O isotope data suggest that Archean juvenile continental crust formed by melting of enriched mafic crust extracted from a still primitive mantle, and that sediment recycling and crustal thickening occurred since the Mesoarchean (e.g., Kemp et al., 2010; Næraa et al., 2012; Reimink et al., 2016; Roberts and Santosh, 2018).
View in article
Laurent, O., Martin, H., Moyen, J.-F., Doucelance, R. (2014) The diversity and evolution of late-Archean granitoids: Evidence for the onset of “modern-style” plate tectonics between 3.0 and 2.5 Ga. Lithos 205, 208-235.

Several of these consider TTGs as slab melts from primitive flat slab subduction zones with a high geothermal gradient and no contribution from the mantle wedge (Smithies, 2000; Laurent et al., 2014).
View in article
Linnen, R.L., Keppler, H. (2002) Melt composition control of Zr/Hf fractionation in magmatic processes. Geochimica et Cosmochimica Acta 66, 3293-3301.

Changes in the Zr/Hf value of tonalitic melt typically result from the crystallisation of ferromagnesian minerals (Linnen and Keppler, 2002) — biotite + amphibole ± plagioclase in this case, with or without garnet (Supplementary Information, Discussion) — which likewise would enrich the melt in highly incompatible B.
View in article
Marschall, H.R., Wanless, V.D., Shimizu, N., Pogge von Strandmann, P.A.E., Elliott, T., Monteleone, B.D. (2017) The boron and lithium isotopic composition of mid-ocean ridge basalts and the mantle. Geochimica et Cosmochimica Acta 207, 102–138.

Due to the enrichment of B and 11B in their source, most magmatic rocks from active margins — including adakites and modern I-type granitoids from continental arcs (Fig. 2) — typically exhibit higher δ11B values than the average continental crust (δ11B = -9.4 ± 0.4 ‰), or mid-oceanic ridge basalts (MORB) and the depleted MORB mantle (DMM; δ11B = -7.1 ± 0.9 ‰; Marschall et al., 2017; Marschall, 2018; δ11B = 1000 × [(11B/10B)sample/(11B/10B)SRM-951 - 1]).
View in article
At chondritic Zr/Hf, TTGs are predicted to have 1-2 ppm B and a δ11B value of ca. -7 ‰, which resembles melts from the DMM (1.2-1.3 ppm, -7.1 ± 0.9 ‰; Marschall et al., 2017).
View in article
Marschall, H.R. (2018) Boron isotopes in the ocean floor realm and the mantle. In: Marschall, H.R., Foster, G.L. (Eds.) Boron Isotopes – The Fifth Element, Advances in Isotope Geochemistry 6. Springer, Heidelberg, 189-215.

Due to the enrichment of B and 11B in their source, most magmatic rocks from active margins — including adakites and modern I-type granitoids from continental arcs (Fig. 2) — typically exhibit higher δ11B values than the average continental crust (δ11B = -9.4 ± 0.4 ‰), or mid-oceanic ridge basalts (MORB) and the depleted MORB mantle (DMM; δ11B = -7.1 ± 0.9 ‰; Marschall et al., 2017; Marschall, 2018; δ11B = 1000 × [(11B/10B)sample/(11B/10B)SRM-951 - 1]).
View in article
Martin, H. (1986) Effect of steeper Archean geothermal gradient on geochemistry of subduction zone magmas. Geology 14, 753–756,

More importantly, high geothermal gradients during the Archean would favour slab melting over slab dehydration (Martin, 1986; Moyen and Martin, 2012), indicating that B and H2O would have been expelled from slabs concomitantly, not sequentially.
View in article
Martin, H., Smithies, R.H., Rapp, R.P., Moyen, J.-F., Champion, D. (2005) An overview of adakite, tonalite trondhjemite granodiorite (TTG), and sanukitoid: relationships and some implications for crustal evolution. Lithos 79, 1-24.

Critical to this debate is the interpretation of tonalite-trondhjemite-granodiorite (TTG) complexes, which make up 90 % of the preserved Archean juvenile continental crust (Martin et al., 2005; Moyen and Martin, 2012).
View in article
As the relevant B reservoirs appear uniform through time (Supplementary Information, Introduction 1), such a signature would be expected for any Archean arc as well — possibly even more so, considering that a contribution from the mantle wedge appears lacking (Martin et al., 2005).
View in article
Moyen, J.-F., Martin, H. (2012) Forty years of TTG research. Lithos 148, 312-336.

Critical to this debate is the interpretation of tonalite-trondhjemite-granodiorite (TTG) complexes, which make up 90 % of the preserved Archean juvenile continental crust (Martin et al., 2005; Moyen and Martin, 2012).
View in article
Interpretations regarding the geodynamic setting of TTG formation are diverse and numerous (Moyen and Martin, 2012).
View in article
Stagnant lid models (e.g., Debaille et al., 2013) provide hybrids to these ‘subduction’ and ‘intraplate’ models (Moyen and Martin, 2012), involving plume-induced melting, as well as flux melting of the mantle during sporadic, local plate burial.
View in article
Figure 1 Trace element concentrations for the TTG samples and average TTG (Moyen and Martin, 2012).
View in article
Such dehydration is not seen even in the warmest of modern arcs and appears inconsistent with the hydrous nature of the TTG source (Moyen and Martin, 2012).
View in article
More importantly, high geothermal gradients during the Archean would favour slab melting over slab dehydration (Martin, 1986; Moyen and Martin, 2012), indicating that B and H2O would have been expelled from slabs concomitantly, not sequentially.
View in article
This considered, the data appear more consistent with the TTG formation via ‘intraplate’ processes (Moyen and Martin, 2012) — e.g., crustal thickening, volcanic resurfacing, overturning, delamination, and stagnant lid or ‘squishy lid’ tectonics (Bédard, 2006; van Kranendonk et al., 2007; Hoffmann et al., 2011; Debaille et al., 2013; Kamber, 2015; Johnson et al., 2017; Rozel et al., 2017) — in which deep hydrated mafic crust with a DMM-like δ11B value melted under the influence of a high or unstable geotherm.
View in article
Næraa, T., Scherstén, A., Rosing, M.T., Kemp, A.I.S., Hoffmann, J.E., Kokfelt, T.F., Whitehouse, M.J. (2012) Hafnium isotope evidence for a transition in the dynamics of continental growth 3.2 Gyr ago. Nature 485, 627-630.

Zircon Hf and O isotope data suggest that Archean juvenile continental crust formed by melting of enriched mafic crust extracted from a still primitive mantle, and that sediment recycling and crustal thickening occurred since the Mesoarchean (e.g., Kemp et al., 2010; Næraa et al., 2012; Reimink et al., 2016; Roberts and Santosh, 2018).
View in article
Rapp, R.P., Watson, E.B., Miller, C. (1991) Partial melting of amphibolite/eclogite and the origin of Archean trondhjemites and tonalities. Precambrian Research 51, 1-25.

These low-K, high-GdN/YbN, I-type granitoids reflect melting of hydrous basaltic sources with residual hornblende and/or garnet (e.g., Arth and Hanson, 1975; Rapp et al., 1991).
View in article
Reimink, J.R., Davies, J.H.F.L., Chacko, T., Stern, R.A., Heaman, L.M., Sarkar, C., Schaltegger, U., Creaser, R.A., Pearson, D.G. (2016) No evidence for Hadean continental crust within Earth’s oldest evolved rock unit. Nature Geoscience 9, 777-780.

Zircon Hf and O isotope data suggest that Archean juvenile continental crust formed by melting of enriched mafic crust extracted from a still primitive mantle, and that sediment recycling and crustal thickening occurred since the Mesoarchean (e.g., Kemp et al., 2010; Næraa et al., 2012; Reimink et al., 2016; Roberts and Santosh, 2018).
View in article
Roberts, N.M.W., Santosh, M. (2018) Capturing the Mesoarchean emergence of continental Crust in the Coorg Block, Southern India. Geophysical Research Letters 45, 7444-7453.

Zircon Hf and O isotope data suggest that Archean juvenile continental crust formed by melting of enriched mafic crust extracted from a still primitive mantle, and that sediment recycling and crustal thickening occurred since the Mesoarchean (e.g., Kemp et al., 2010; Næraa et al., 2012; Reimink et al., 2016; Roberts and Santosh, 2018).
View in article
Rozel, A.B., Golabek, G.J., Jain, C., Tackley, P.J., Gerya, T. (2017) Continental crust formation on early Earth controlled by intrusive magmatism. Nature 545, 332-335.

This considered, the data appear more consistent with the TTG formation via ‘intraplate’ processes (Moyen and Martin, 2012) — e.g., crustal thickening, volcanic resurfacing, overturning, delamination, and stagnant lid or ‘squishy lid’ tectonics (Bédard, 2006; van Kranendonk et al., 2007; Hoffmann et al., 2011; Debaille et al., 2013; Kamber, 2015; Johnson et al., 2017; Rozel et al., 2017) — in which deep hydrated mafic crust with a DMM-like δ11B value melted under the influence of a high or unstable geotherm.
View in article
Ryan, J.G., Chauvel, C. (2014) The subduction-zone filter and the impact of recycled materials on the evolution of the mantle. In: Holland, H.D., Turekian, K.K. (Eds.) Treatise on Geochemistry 3: The Mantle and Core. Elsevier, Amsterdam, 479-508.

Most importantly, seawater shows a very strong long term enrichment in the heavy isotope 11B, which is reflected in the B chemistry of subducted slabs and magmatic rocks from active margins (Ryan and Chauvel, 2014; de Hoog and Savov, 2018).
View in article
Sisson, T.W., Layne, G.D. (1993) H2O in basalt and basaltic andesite glass inclusions from four subduction-related volcanoes. Earth and Planetary Science Letters 117, 619-635.

Crystallisation of basaltic and andesitic magmas is associated with the exsolution of H2O and other volatiles (Sisson and Layne, 1993).
View in article
Smithies, R.H. (2000) The Archaean tonalite–trondhjemite–granodiorite (TTG) series is not an analogue of Cenozoic adakite. Earth and Planetary Science Letters 182, 115-125.

Several of these consider TTGs as slab melts from primitive flat slab subduction zones with a high geothermal gradient and no contribution from the mantle wedge (Smithies, 2000; Laurent et al., 2014).
View in article
Others models suggest that TTGs represent melts from thickened mafic crust produced during episodes of density-driven crustal overturning, delamination, underplating or plume activity (Smithies, 2000; Bédard, 2006; van Kranendonk et al., 2007; Kamber, 2015; Johnson et al., 2017).
View in article
Trail, D., Boehnke, P., Savage, P., Liu, M.-C., Miller, M.L., Bindeman, I. (2018) Origin and significance of Si and O isotope heterogeneities in Phanerozoic, Archean, and Hadean zircon. Proceedings of the National Academy of Sciences 115, 10287-10292.

The same is indicated by Si-O isotope data for Archean detrital zircon (Trail et al., 2018).
View in article
The integral Si-O isotope record of Hadean zircon and Archean TTGs indicates source contributions from a variety of supra-crustal rocks, including chemical sediments, serpentinised and silicified basalts (Trail et al., 2018; André et al., 2019).
View in article
Although Si-O data provide a unique opportunity to discern supra-crustal contributions to TTG sources (Trail et al., 2018), their interpretation to discern the geodynamic setting of TTG formation is still controversial.
View in article
Valley, J.W., Lackey, J.S., Cavosie, A.J., Clechenko, C.C., Spicuzza, M.J., Basei, M.A.S., Bindeman, I.N., Ferreira, V.P., Sial, A.N., King, E.M., Peck, W.H., Sinha, A.K., Wei, C.S. (2005) 4.4 billion years of crustal maturation: oxygen isotope ratios of magmatic zircon. Contributions to Mineralogy and Petrology 150, 561-580.

These interpretations fit the observation that high 18O/16O granitoids produced by melting of surface-altered rocks are restricted to post-Archean time when recycling of such material, possibly by subduction, became prevalent (Valley et al., 2005).
View in article
The elevated 18O/16O values that — together with elevated 30Si/28Si values — were taken to represent the chert component actually partly exceed those typically observed for Archean TTGs; these could reflect secondary 18O-enrichment via low temperature alteration (Valley et al., 2005).
View in article
van Kranendonk, M.J., Smithies, R.H., Hickman, A.H., Champion, D.C. (2007) Secular tectonic evolution of Archean continental crust: interplay between horizontal and vertical processes in the formation of the Pilbara Craton, Australia. Terra Nova 19, 1-38.

Others models suggest that TTGs represent melts from thickened mafic crust produced during episodes of density-driven crustal overturning, delamination, underplating or plume activity (Smithies, 2000; Bédard, 2006; van Kranendonk et al., 2007; Kamber, 2015; Johnson et al., 2017).
View in article
This considered, the data appear more consistent with the TTG formation via ‘intraplate’ processes (Moyen and Martin, 2012) — e.g., crustal thickening, volcanic resurfacing, overturning, delamination, and stagnant lid or ‘squishy lid’ tectonics (Bédard, 2006; van Kranendonk et al., 2007; Hoffmann et al., 2011; Debaille et al., 2013; Kamber, 2015; Johnson et al., 2017; Rozel et al., 2017) — in which deep hydrated mafic crust with a DMM-like δ11B value melted under the influence of a high or unstable geotherm.
View in article
top
Supplementary Information
The Supplementary Information includes:
- Supplementary Introduction 1 to 3
- Supplementary Analytical Methods and Data
- Supplementary Discussion
- Figures S-1 to S-7
- Tables S-1 to S-9
- Supplementary Information References
Download the Supplementary Information (PDF).
Download Table S-1 (Excel).
Figures and Tables

Figure 1 Trace element concentrations for the TTG samples and average TTG (Moyen and Martin, 2012
Moyen, J.-F., Martin, H. (2012) Forty years of TTG research. Lithos 148, 312-336.
). All data are normalised to primitive mantle (PM; data in Table S-7).
Figure 2 (a) B and (b) δ11B versus Zr/Hf with the correlation coefficients for the given regressions.
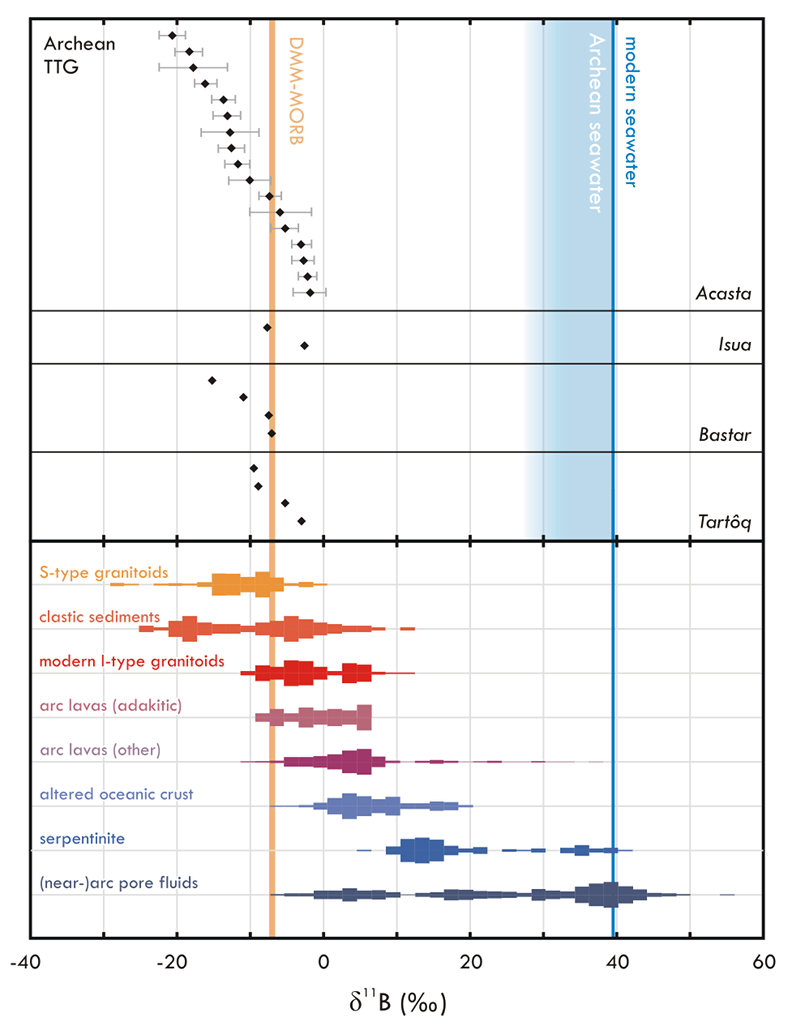
Figure 3 Boron isotope data for the analysed samples and terrestrial reservoirs (histograms). Error bars for whole rock analyses are smaller than the symbols. Acasta data represents bulk rock δ11B modelled from biotite data (Supplementary Information, Analytical Methods and Data). The values for reference reservoirs are in Table S-1.
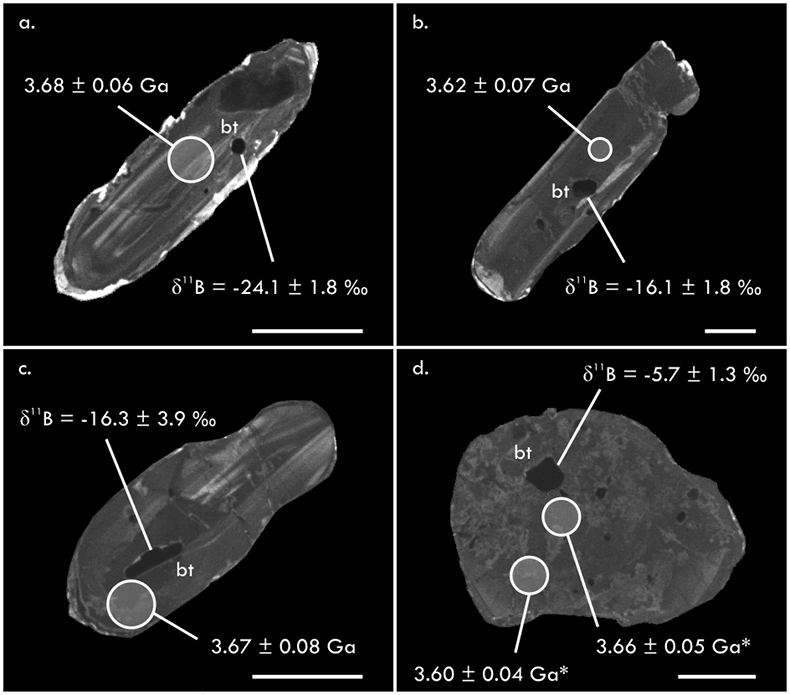
Figure 4 Cathodoluminescence images of zircon from the Acasta Gneiss Complex samples showing analysed biotite inclusions. The δ11B values of biotite (in ‰) and the 207Pb/206Pb age of the surrounding zircon (minimum inclusion age) are shown. Analytical spots are to scale; asterisk marks >10 % discordance; scale bars are 50 μm. Data are in Table S-9.