Fate of organic compounds during transformation of ferrihydrite in iron formations
Affiliations | Corresponding Author | Cite as | Funding information- Share this article
-
Article views:465Cumulative count of HTML views and PDF downloads.
- Download Citation
- Rights & Permissions
top
Abstract
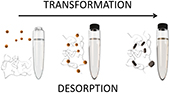
Figures and Tables
![]() Figure 1 (a) Schematics of the DFS (Dynamic Force Spectroscopy): self assembled monolayers with carboxyl, hydroxyl and phosphate headgroups covalently bonded to AFM tip. (b) A scheme of a force curve. (c) In transformation experiments, ferrihydrite and glycerol were mixed at room temperature and left to equilibrate overnight. One sample was then taken for TGA (equilibrated sample) and the rest was placed in the oven at 90 °C until the transformation was complete (aged sample). | ![]() Figure 2 Multibond fit (Friddle et al., 2012) to the dynamic force spectra between hematite surface and alkyl thiol self assembled monolayers with OH, | ![]() Figure 3 Comparison of thermogravimetry curves of ferrihydrite-glycerol (equilibrium-black curve) and resulting hematite-glycerol (aged-red curve). The smaller mass loss in the region 125–700 °C (dashed vertical lines) for the aged sample compared to the equilibrium sample suggests bigger loss of tightly bound water and glycerol during the transformation. | ![]() Table 1 Weight loss during the TGA analysis in <125 °C and 125–700 °C regions. |
Figure 1 | Figure 2 | Figure 3 | Table 1 |
top
Introduction
Traditionally, iron formations (IFs) have been considered as abiotically generated chemical sediments but an increasing body of evidence suggests the active role of microbes in their precipitation (Koehler et al., 2010
Koehler, I., Konhauser, K., Kappler, A. (2010) Role of Microorganisms in Banded Iron Formations. In: Barton, L.L., Mandl, M., Loy, A (Eds.) Geomicrobiology: Molecular and Environmental Perspective. Springer Netherlands, Dordrecht, 309–324.
). The role of anoxygenic phototrophic bacteria in the formation of IFs has been the object of speculation since the work of Garrels et al. (1973)Garrels, R.M., Perry, E.A., Mackenzie, F.T. (1973) Genesis of Precambrian Iron-Formations and the Development of Atmospheric Oxygen. Economic Geology 68, 1173–1179.
. An improved understanding of microbially induced Fe(II) oxidation highlights that oxidising bacteria, such as Rhodobacter ferrooxidans, could likely drive the formation of IFs (Kappler et al., 2005Kappler, A., Pasquero, C., Konhauser, K.O., Newman, D.K. (2005) Deposition of banded iron formations by anoxygenic phototrophic Fe(II)-oxidizing bacteria. Geology 33, 865–868.
). Further, mass balance calculations have shown that Fe(II)-oxidising phototrophic bacteria have the capacity to oxidise all Fe(II) from the Precambrian ocean, causing formation of iron (oxyhydr)oxide (FeOx) (Hegler et al., 2008Hegler, F., Posth, N.R., Jiang, J., Kappler, A. (2008) Physiology of phototrophic iron(II)-oxidizing bacteria: implications for modern and ancient environments. FEMS Microbiology Ecology 66, 250–260.
). However, the low concentration of organic compounds in IFs and their diagenetic or metamorphic derivatives, challenges the hypothesis of their biogenic origin (Klein, 2005Klein, C. (2005) Some Precambrian banded iron-formations (BIFs) from around the world: Their age, geologic setting, mineralogy, metamorphism, geochemistry, and origins. American Mineralogist 90, 1473–1499.
).Extracellular polymeric substances (EPS) promote FeOx nucleation (Sand et al., 2020
Sand, K.K., Jelavić, S., Dobberschütz, S., Ashby, P.D., Marshall, M.J., Dideriksen, K., Stipp, S.L.S., Kerisit, S.N., Friddle, R.W., DeYoreo, J.J. (2020) Mechanistic insight into biopolymer induced iron oxide mineralization through quantification of molecular bonding. Nanoscale Advances 2, 3323–3333.
) where microbially formed FeOx are often found in close association with the EPS (Chan et al., 2004Chan, C.S., Stasio, G.D., Welch, S.A., Girasole, M., Frazer, B.H., Nesterova, M.V., Fakra, S., Banfield, J.F. (2004) Microbial Polysaccharides Template Assembly of Nanocrystal Fibers. Science 303, 1656–1658.
). When EPS is encrusted with FeOx, the polymers are shed and new EPS are formed. This prevents the encrustation of the microbe itself (Phoenix et al., 2000Phoenix, V.R., Adams, D.G., Konhauser, K.O. (2000) Cyanobacterial viability during hydrothermal biomineralisation. Chemical Geology 169, 329–338.
; Chan et al., 2004Chan, C.S., Stasio, G.D., Welch, S.A., Girasole, M., Frazer, B.H., Nesterova, M.V., Fakra, S., Banfield, J.F. (2004) Microbial Polysaccharides Template Assembly of Nanocrystal Fibers. Science 303, 1656–1658.
). During IF formation, FeOx-EPS composites would have settled through the water column and been deposited on the sea floor. Some of the organic compounds were degraded by diagenetic and metamorphic processes (Köhler et al., 2013Köhler, I., Konhauser, K.O., Papineau, D., Bekker, A., Kappler, A. (2013) Biological carbon precursor to diagenetic siderite with spherical structures in iron formations. Nature Communications 4, 1–7.
; Posth et al., 2013Posth, N.R., Köhler, I., Swanner, E.D., Schröder, C., Wellmann, E., Binder, B., Konhauser, K.O., Neumann, U., Berthold, C., Nowak, M., Kappler, A. (2013) Simulating Precambrian banded iron formation diagenesis. Chemical Geology, Special Issue dedicated to H.D. Holland: Evolution of the atmosphere and ocean through time 362, 66–73.
; Halama et al., 2016Halama, M., Swanner, E.D., Konhauser, K.O., Kappler, A. (2016) Evaluation of siderite and magnetite formation in BIFs by pressure–temperature experiments of Fe(III) minerals and microbial biomass. Earth and Planetary Science Letters 450, 243–253.
). In addition, there is evidence that the depositional environment was most likely scarce in organic compounds (Dodd et al., 2019Dodd, M.S., Papineau, D., Pirajno, F., Wan, Y., Karhu, J.A. (2019) Minimal biomass deposition in banded iron formations inferred from organic matter and clay relationships. Nature Communications 10, 1–13.
). Thus, the majority of the organic compounds was likely lost prior to deposition.Hematite is a major component of IFs found today (Konhauser et al., 2017
Konhauser, K.O., Planavsky, N.J., Hardisty, D.S., Robbins, L.J., Warchola, T.J., Haugaard, R., Lalonde, S.V., Partin, C.A., Oonk, P.B.H., Tsikos, H., Lyons, T.W., Bekker, A., Johnson, C.M. (2017) Iron formations: A global record of Neoarchaean to Palaeoproterozoic environmental history. Earth-Science Reviews 172, 140–177.
) but, initially, the FeOx in the FeOx-polymer complexes were most likely composed of ferrihydrite (Chan et al., 2004Chan, C.S., Stasio, G.D., Welch, S.A., Girasole, M., Frazer, B.H., Nesterova, M.V., Fakra, S., Banfield, J.F. (2004) Microbial Polysaccharides Template Assembly of Nanocrystal Fibers. Science 303, 1656–1658.
). The transformation to hematite would have happened in aqueous solutions both before (Sun et al., 2015Sun, S., Konhauser, K.O., Kappler, A., Li, Y.-L. (2015) Primary hematite in Neoarchean to Paleoproterozoic oceans. GSA Bulletin 127, 850–861.
) and after deposition on the seabed. Depending on the solution conditions, ferrihydrite to hematite transformation can involve intermediary phases such as lepidocrocite and goethite, e.g., through Fe(II) catalysed transformation (Hansel et al., 2003Hansel, C.M., Benner, S.G., Neiss, J., Dohnalkova, A., Kukkadapu, R.K., Fendorf, S. (2003) Secondary mineralization pathways induced by dissimilatory iron reduction of ferrihydrite under advective flow. Geochimica et Cosmochimica Acta 67, 2977–2992.
), or it can be direct (Cudennec and Lecerf, 2006Cudennec, Y., Lecerf, A. (2006) The transformation of ferrihydrite into goethite or hematite, revisited. Journal of Solid State Chemistry 179, 716–722.
). Transformation involving intermediary phases is a dissolution-precipitation process (Schwertmann and Murad, 1983Schwertmann, U., Murad, E. (1983) Effect of pH on the Formation of Goethite and Hematite from Ferrihydrite. Clays and Clay Minerals 31, 277–284.
) implying that the interface between ferrihydrite and organic compounds is eliminated. In this scenario, organic compounds would have been released to solution where they could have been re-adsorbed but less strongly bound to newly formed phases (Chen et al., 2015Chen, C., Kukkadapu, R., Sparks, D.L. (2015) Influence of Coprecipitated Organic Matter on Fe2+(aq)-Catalyzed Transformation of Ferrihydrite: Implications for Carbon Dynamics. Environmental Science & Technology 49, 10927–10936.
), or released in the water column and subsequently degraded by various biotic and abiotic processes (Kleber et al., 2015Kleber, M., Eusterhues, K., Keiluweit, M., Mikutta, C., Mikutta, R., Nico, P.S. (2015) Chapter One – Mineral–Organic Associations: Formation, Properties, and Relevance in Soil Environments. In: Sparks, D.L. (Ed.) Advances in Agronomy. Academic Press, Cambridge, 1–140.
). The direct transformation from ferrihydrite to hematite is a solid-state transition where atoms move only locally to occupy new structural positions (Cudennec and Lecerf, 2006Cudennec, Y., Lecerf, A. (2006) The transformation of ferrihydrite into goethite or hematite, revisited. Journal of Solid State Chemistry 179, 716–722.
), without the loss of an interface with adsorbed complexes. Thus, the direct transformation of ferrihydrite to hematite is not necessarily accompanied by a removal of organic compounds. However, the Gibbs free energy of binding (ΔGbu) between ferrihydrite and EPS is larger than between hematite and EPS (Sand et al., 2020Sand, K.K., Jelavić, S., Dobberschütz, S., Ashby, P.D., Marshall, M.J., Dideriksen, K., Stipp, S.L.S., Kerisit, S.N., Friddle, R.W., DeYoreo, J.J. (2020) Mechanistic insight into biopolymer induced iron oxide mineralization through quantification of molecular bonding. Nanoscale Advances 2, 3323–3333.
) implying that the polymers were more likely to desorb from hematite than from ferrihydrite. In such a case, the absence of organic compounds in IFs cannot be an argument against their biogenic origin.To understand better the fate of adsorbed organic compounds during direct transformation from ferrihydrite to hematite, we used dynamic force spectroscopy (DFS) to measure the energy of binding between hematite and representative organic functional groups found in EPS. Subsequently we identified the functional group least likely to desorb during transformation and carried out transformation experiments where we used thermogravimetric analysis (TGA) to measure the loss of organic compounds during transformation.
top
Materials and Methods
DFS. We used an Asylum MFP3D atomic force microscope (AFM) and functionalised gold coated AFM tips (MSCT, Bruker) with carboxyl (COO−), hydroxyl (OH), and phosphate (

Jelavić, S., Tobler, D.J., Hassenkam, T., Yoreo, J.J.D., Stipp, S.L.S., Sand, K.K. (2017) Prebiotic RNA polymerisation: energetics of nucleotide adsorption and polymerisation on clay mineral surfaces. Chemical Communications 53, 12700–12703.
(details in SI).
Figure 1 (a) Schematics of the DFS (Dynamic Force Spectroscopy): self assembled monolayers with carboxyl, hydroxyl and phosphate headgroups covalently bonded to AFM tip. (b) A scheme of a force curve. (c) In transformation experiments, ferrihydrite and glycerol were mixed at room temperature and left to equilibrate overnight. One sample was then taken for TGA (equilibrated sample) and the rest was placed in the oven at 90 °C until the transformation was complete (aged sample).
We used the {0001} face for DFS because it is one of the most common hematite surfaces in the environment (Hartman, 1989
Hartman, P. (1989) The effect of surface relaxation on crystal habit: Cases of corundum (α-Al2O3) and Hematite (α-Fe2O3). Journal of Crystal Growth 96, 667–672.
) and because it is a predominant face on hematite produced by transformation of ferrihydrite (Cornell and Schwertmann, 2004Cornell, R.M., Schwertmann, U. (2004) Crystal Morphology and Size. In: The Iron Oxides. Wiley-VCH Verlag Gmbh & Co., Weinheim, 59–94.
). The specular hematite monocrystal {0001} face was cleaned prior to analysis following the protocol described in Jelavić et al. (2017)Jelavić, S., Tobler, D.J., Hassenkam, T., Yoreo, J.J.D., Stipp, S.L.S., Sand, K.K. (2017) Prebiotic RNA polymerisation: energetics of nucleotide adsorption and polymerisation on clay mineral surfaces. Chemical Communications 53, 12700–12703.
(details in the SI). For DFS measurements, we collected >500 force curves per experiment. The tip approaching velocity was set to 500 nms−1 and the retraction velocity varied between 10–10000 nms−1. The trigger force was set to 100 pN and dwell time to 0.5 s. During the measurement, the head groups bound to the hematite surface (Fig. 1a) and we measured the forces applied to break the bond (i.e. the force curve, Fig. 1b). We fit the rupture forces vs. the loading rate to a multibond model (Friddle et al., 2012Friddle, R.W., Noy, A., Yoreo, J.J.D. (2012) Interpreting the widespread nonlinear force spectra of intermolecular bonds. Proceedings of the National Academy of Sciences 109, 13573–13578.
) enabling calculation of ΔGbu (Eq. S-1 to Eq. S-4). The measurements were done in 10 mM NaCl solution at pH = 5.6. We chose these conditions, rather than conditions at which the transformation experiments were conducted, to maximise the interaction forces between the tip and hematite surface (Lützenkirchen et al., 2013Lützenkirchen, J., Preočanin, T., Stipić, F., Heberling, F., Rosenqvist, J., Kallay, N. (2013) Surface potential at the hematite (001) crystal plane in aqueous environments and the effects of prolonged aging in water. Geochimica et Cosmochimica Acta 120, 479–486.
; Newcomb et al., 2017Newcomb, C.J., Qafoku, N.P., Grate, J.W., Bailey, V.L., Yoreo, J.J.D. (2017) Developing a molecular picture of soil organic matter–mineral interactions by quantifying organo–mineral binding. Nature Communications 8, 396.
).Transformation experiments. Ferrihydrite was synthesised using the method of Schwertmann and Cornell (2000)
Schwertmann, U., Cornell, R.M. (2000) Ferrihydrite. In: Iron Oxides in the Laboratory. John Wiley & Sons, Ltd, Weinheim, 103–112.
. We used 0.5 M NaCl solution and pH = 7 as a proxy for Precambrian seawater. We chose glycerol (CH2OH-CHOH-CH2OH) as a model for an OH-rich molecule. Adsorption of polymers is a complex function of degree of branching, length and hydrophobicity (van Oss, 1997van Oss, C.J. (1997) Hydrophobicity and hydrophilicity of biosurfaces. Current Opinion in Colloid & Interface Science 2, 503–512.
). The aim here is not to account for such variations but to isolate the effect of ΔGbu, which is independent of those parameters, on the magnitude of desorption during transformation. Coprecipitation of ferrihydrite-polymer aggregates results in a high organic content compared to adsorbed polymers (Mikutta et al., 2014Mikutta, R., Lorenz, D., Guggenberger, G., Haumaier, L., Freund, A. (2014) Properties and reactivity of Fe-organic matter associations formed by coprecipitation versus adsorption: Clues from arsenate batch adsorption. Geochimica et Cosmochimica Acta 144, 258–276.
) and can cause variations in grain size and aggregation. To avoid any influence from such variations and to avoid low transformation rates related to high surface coverage, we adsorbed glycerol to ferrihydrite in this study. We mixed 15 mg of ferrihydrite with 15 ml of 0.5 M NaCl and added glycerol to a final concentration of 0.5 % (Fig. 1c). In control samples, we omitted glycerol. Samples were shaken at 100 rpm overnight to equilibrate. The next day, a batch of samples was rinsed with 20 ml of rinsing stock solution (0.5 M NaCl adjusted to pH = 7 with 1 M NaOH) by ultra-centrifugation (equilibrated sample). Another batch was placed in the oven heated to 90 °C. The samples from the oven were sampled at specific time steps to follow the transformation pathway using X-ray diffraction (XRD). When sampled, a batch was rinsed with 20 ml of 0.5 M NaCl that was adjusted to pH = 7 (aged sample). All samples were freeze dried after rinsing.XRD. Samples for XRD were washed with ultra-deionised water (resistivity >18.2 MΩcm). A volume of 1.5 ml of suspension was pipetted on the zero background Si holders and left to dry at room temperature. Such sample preparation results in large preferred orientation of anisotropic grains such as goethite and lepidocrocite and allows us to detect minute quantities of crystalline material in a poorly ordered matrix of ferrihydrite. We collected diffractograms in reflection mode on the Bruker D8 Advance instrument using Cu Kα radiation (λ = 1.5418 Å) operated at 40 kV and 40 mA, and a LynxEye detector. Diffractograms were collected from 10–90° 2θ with step size of 0.02° and 1.7 s counting time per step. The sample was spun at 20 rpm. We used 0.3° divergence and 3° antiscatter slits, 2.5° Soller slits on incident and diffracted beams and a 0.02 mm thick Ni-filter. The opening of detector window was 2.94°.
TGA. We used Netzsch TG 209 F1 Libra. Samples were heated at a rate of 10 °C min−1 from 30 to 1000 °C under N2 atmosphere. ∼15 mg of sample were placed into the Pt crucible and the weight loss was measured as a function of temperature.
TEM. Images were taken with a Philips CM 20 TEM equipped with a thermionic LaB6 filament. We used an accelerating voltage of 200 kV. Samples were prepared by placing a droplet of sample suspension on a formvar coated TEM grid and left for 5–10 s. Subsequently, the grid was washed in ultra-deionised water and water droplets were removed with the edge of a paper towel.
top
Results and Discussion
Energy of binding. The force spectra (Fig. 2) show that the rupture force between hematite and the OH group is ∼6 times higher than between COO− and

Israelachvili, J.N. (2011) Intermolecular and Surface Forces. Third edition, Elsevier, Burlington.
). Considering that the {0001} hematite surface is completely hydroxylated in water (Trainor et al., 2004Trainor, T.P., Chaka, A.M., Eng, P.J., Newville, M., Waychunas, G.A., Catalano, J.G., Brown, G.E. (2004) Structure and reactivity of the hydrated hematite (0001) surface. Surface Science 573, 204–224.
), our results follow the trend where OH-OH bonds are twice as strong as COO−-OH bonds at circumneutral pH (Vezenov et al., 1997Vezenov, D.V., Noy, A., Rozsnyai, L.F., Lieber, C.M. (1997) Force Titrations and Ionization State Sensitive Imaging of Functional Groups in Aqueous Solutions by Chemical Force Microscopy. Journal of the American Chemical Society 119, 2006–2015.
) present in Precambrian ocean. Thus, irrespective of the transformation pathway, biopolymers rich in acidic groups desorb more easily from hematite than those rich in hydroxyl groups.
Figure 2 Multibond fit (Friddle et al., 2012
Friddle, R.W., Noy, A., Yoreo, J.J.D. (2012) Interpreting the widespread nonlinear force spectra of intermolecular bonds. Proceedings of the National Academy of Sciences 109, 13573–13578.
) to the dynamic force spectra between hematite surface and alkyl thiol self assembled monolayers with OH,
Transformation pathway. To investigate the fate of a ferrihydrite-associated OH-containing molecule during transformation to hematite, we transformed the ferrihydrite-glycerol complex, monitored the transformation pathway with XRD and determined the weight loss with TGA. The ferrihydrite-glycerol complex transformed directly to hematite without any intermediate phases detectable by XRD (Fig. S-3) suggesting a solid-state transformation pathway. As a control, we transformed ferrihydrite in absence of glycerol using the same solution conditions. Even though the hematite was the first phase to occur after 17 hr, goethite started forming after 43 hr (Fig. S-2) indicating that, in pure systems, some ferrihydrite dissolves and reprecipitates as goethite, as previously shown (Das et al., 2011
Das, S., Hendry, M.J., Essilfie-Dughan, J. (2011) Transformation of Two-Line Ferrihydrite to Goethite and Hematite as a Function of pH and Temperature. Environmental Science & Technology 45, 268–275.
).The transformation is accompanied by glycerol release. In general, the ferrihydrite-gycerol complex lost more weight than the pure ferrihydrite (Fig. 3, Table 1). Ferrihydrite is more hydrated than hematite so the loss of loosely adsorbed water (<125 °C) and tightly bound water (125–700 °C) is higher for ferrihydrite than hematite (Hiemstra and Van Riemsdijk, 2009
Hiemstra, T., Van Riemsdijk, W.H. (2009) A surface structural model for ferrihydrite I: Sites related to primary charge, molar mass, and mass density. Geochimica et Cosmochimica Acta 73, 4423–4436.
). Comparing the weight loss of ferrihydrite-glycerol (5.4 %; Table 1) to pure ferrihydrite (4.7 %) in the 125–700 °C range, shows that the tightly bound water accounts only for 87 % of the weight loss in this region, indicating that the remaining 13 % is the loss of glycerol and associated interfacial water. This implies that hematite produced by aging ferrihydrite-glycerol contains less glycerol than the original ferrihydrite-glycerol (equilibrated), and that glycerol is released during transformation. Considering that the initial amount of adsorbed glycerol is ∼5 mg/g of ferrihydrite (Fig. S-1), TGA indicates that all glycerol has been desorbed during the transformation.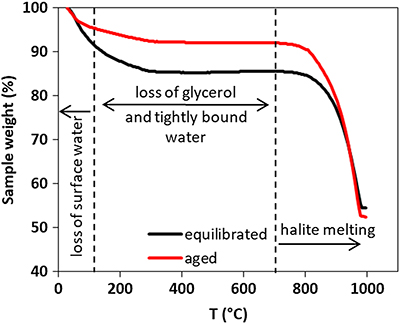
Figure 3 Comparison of thermogravimetry curves of ferrihydrite-glycerol (equilibrium-black curve) and resulting hematite-glycerol (aged-red curve). The smaller mass loss in the region 125–700 °C (dashed vertical lines) for the aged sample compared to the equilibrium sample suggests bigger loss of tightly bound water and glycerol during the transformation.
Table 1 Weight loss during the TGA analysis in <125 °C and 125–700 °C regions.
Sample | % weight loss | ||
<125 °C | 125–700 °C | ||
Glycerol | equilibrated | 8.9 | 5.4 |
aged | 4.8 | 3.2 | |
Control | equilibrated | 9.8 | 4.7 |
aged | n.a. | n.a. |
* transformation yielded both goethite and hematite making comparison impossible
The reason for the loss of the glycerol during transformation can also be the smaller specific surface area (SSA) of the produced hematite (Fig. S-4) and not only the lower affinity for glycerol compared to ferrihydrite. From TEM images, we estimated the SSA of ferrihydrite to be 374–790 m2g−1 and of hematite to be 10–46 m2g−1. Thus, the decrease in the SSA because of grain coarsening during transformation is between 9–70 times which alone might explain the decrease in glycerol content. However, the loss must be amplified by the lower ΔGbu for hematite-OH than for ferrihydrite-OH system. In addition, our thermodynamic results suggest that the loss of organic compounds would have happened because of decreased ΔGbu even if the subsequent grain coarsening does not occur, e.g., in case of hematite growth inhibition caused by organic compounds. However, both scenarios are likely to have contributed to the loss of organic compounds during the formation of IFs.
We have demonstrated that glycerol, a molecule with a high ΔGbu to hematite, desorbs during the transformation of ferrihydrite to hematite. We propose that a significant mass of organic compounds from FeOx-EPS composites is desorbed early in the process of FeOx sedimentation because of the transformation from ferrihydrite to hematite. This loss of organic compounds is probably further enhanced by the grain coarsening of hematite during diagenetic and metamorphic processes and concomitant reactions in the microenvironment that result in secondary mineralisation, e.g., Posth et al. (2013)
Posth, N.R., Köhler, I., Swanner, E.D., Schröder, C., Wellmann, E., Binder, B., Konhauser, K.O., Neumann, U., Berthold, C., Nowak, M., Kappler, A. (2013) Simulating Precambrian banded iron formation diagenesis. Chemical Geology, Special Issue dedicated to H.D. Holland: Evolution of the atmosphere and ocean through time 362, 66–73.
. Our experiments were designed to determine the loss of strongly bound molecules from FeOx during the direct transformation, which is least likely to result in desorption of adsorbed organic compounds. The less strongly bound organic compounds or organic compounds adsorbed to ferrihydrite that transformed via dissolution-precipitation pathway would likely have desorbed in higher proportion than that we report here. Thus, our results highlight that the absence of organic compounds in IF should not be used as evidence against their biogenic origin.top
Acknowledgements
We thank Heloisa N. Bordallo for access to TGA instrument (Carlsbergfondets, grant no. 2013_01_0589). KKS and ACM are grateful for funding from the European Union’s Horizon 2020 Research and Innovation Programme under Marie Skłodowska-Curie Grant Agreement No 663830 and the Welsh Government and Higher Education Funding Council for Wales through the Sêr Cymru National Research Network for Low Carbon, Energy and Environment. KKS is grateful for funding from the Danish Council for Independent Research Sapere Aude Programs (0602-02654B).
Editor: Karim Benzerara
top
References
Chan, C.S., Stasio, G.D., Welch, S.A., Girasole, M., Frazer, B.H., Nesterova, M.V., Fakra, S., Banfield, J.F. (2004) Microbial Polysaccharides Template Assembly of Nanocrystal Fibers. Science 303, 1656–1658.

Extracellular polymeric substances (EPS) promote FeOx nucleation (Sand et al., 2020) where microbially formed FeOx are often found in close association with the EPS (Chan et al., 2004).
View in article
This prevents the encrustation of the microbe itself (Phoenix et al., 2000; Chan et al., 2004).
View in article
Hematite is a major component of IFs found today (Konhauser et al., 2017) but, initially, the FeOx in the FeOx-polymer complexes were most likely composed of ferrihydrite (Chan et al., 2004).
View in article
Chen, C., Kukkadapu, R., Sparks, D.L. (2015) Influence of Coprecipitated Organic Matter on Fe2+(aq)-Catalyzed Transformation of Ferrihydrite: Implications for Carbon Dynamics. Environmental Science & Technology 49, 10927–10936.

In this scenario, organic compounds would have been released to solution where they could have been re-adsorbed but less strongly bound to newly formed phases (Chen et al., 2015), or released in the water column and subsequently degraded by various biotic and abiotic processes (Kleber et al., 2015).
View in article
Cornell, R.M., Schwertmann, U. (2004) Crystal Morphology and Size. In: The Iron Oxides. Wiley-VCH Verlag Gmbh & Co., Weinheim, 59–94.

We used the {0001} face for DFS because it is one of the most common hematite surfaces in the environment (Hartman, 1989) and because it is a predominant face on hematite produced by transformation of ferrihydrite (Cornell and Schwertmann, 2004).
View in article
Cudennec, Y., Lecerf, A. (2006) The transformation of ferrihydrite into goethite or hematite, revisited. Journal of Solid State Chemistry 179, 716–722.

Depending on the solution conditions, ferrihydrite to hematite transformation can involve intermediary phases such as lepidocrocite and goethite, e.g., through Fe(II) catalysed transformation (Hansel et al., 2003), or it can be direct (Cudennec and Lecerf, 2006).
View in article
The direct transformation from ferrihydrite to hematite is a solid-state transition where atoms move only locally to occupy new structural positions (Cudennec and Lecerf, 2006), without the loss of an interface with adsorbed complexes.
View in article
Das, S., Hendry, M.J., Essilfie-Dughan, J. (2011) Transformation of Two-Line Ferrihydrite to Goethite and Hematite as a Function of pH and Temperature. Environmental Science & Technology 45, 268–275.

Even though the hematite was the first phase to occur after 17 hr, goethite started forming after 43 hr (Fig. S-2) indicating that, in pure systems, some ferrihydrite dissolves and reprecipitates as goethite, as previously shown (Das et al., 2011).
View in article
Dodd, M.S., Papineau, D., Pirajno, F., Wan, Y., Karhu, J.A. (2019) Minimal biomass deposition in banded iron formations inferred from organic matter and clay relationships. Nature Communications 10, 1–13.

In addition, there is evidence that the depositional environment was most likely scarce in organic compounds (Dodd et al., 2019). Thus, the majority of the organic compounds was likely lost prior to deposition.
View in article
Friddle, R.W., Noy, A., Yoreo, J.J.D. (2012) Interpreting the widespread nonlinear force spectra of intermolecular bonds. Proceedings of the National Academy of Sciences 109, 13573–13578.

We fit the rupture forces vs. the loading rate to a multibond model (Friddle et al., 2012) enabling calculation of ΔGbu (Eq. S-1 to Eq. S-4).
View in article
Multibond fit (Friddle et al., 2012) to the dynamic force spectra between hematite surface and alkyl thiol self assembled monolayers with OH, and COO− head groups.
View in article
Garrels, R.M., Perry, E.A., Mackenzie, F.T. (1973) Genesis of Precambrian Iron-Formations and the Development of Atmospheric Oxygen. Economic Geology 68, 1173–1179.

The role of anoxygenic phototrophic bacteria in the formation of IFs has been the object of speculation since the work of Garrels et al. (1973).
View in article
Halama, M., Swanner, E.D., Konhauser, K.O., Kappler, A. (2016) Evaluation of siderite and magnetite formation in BIFs by pressure–temperature experiments of Fe(III) minerals and microbial biomass. Earth and Planetary Science Letters 450, 243–253.

Some of the organic compounds were degraded by diagenetic and metamorphic processes (Köhler et al., 2013; Posth et al., 2013; Halama et al., 2016).
View in article
Hansel, C.M., Benner, S.G., Neiss, J., Dohnalkova, A., Kukkadapu, R.K., Fendorf, S. (2003) Secondary mineralization pathways induced by dissimilatory iron reduction of ferrihydrite under advective flow. Geochimica et Cosmochimica Acta 67, 2977–2992.

Depending on the solution conditions, ferrihydrite to hematite transformation can involve intermediary phases such as lepidocrocite and goethite, e.g., through Fe(II) catalysed transformation (Hansel et al., 2003), or it can be direct (Cudennec and Lecerf, 2006).
View in article
Hartman, P. (1989) The effect of surface relaxation on crystal habit: Cases of corundum (α-Al2O3) and Hematite (α-Fe2O3). Journal of Crystal Growth 96, 667–672.

We used the {0001} face for DFS because it is one of the most common hematite surfaces in the environment (Hartman, 1989) and because it is a predominant face on hematite produced by transformation of ferrihydrite (Cornell and Schwertmann, 2004).
View in article
Hegler, F., Posth, N.R., Jiang, J., Kappler, A. (2008) Physiology of phototrophic iron(II)-oxidizing bacteria: implications for modern and ancient environments. FEMS Microbiology Ecology 66, 250–260.

Further, mass balance calculations have shown that Fe(II)-oxidising phototrophic bacteria have the capacity to oxidise all Fe(II) from the Precambrian ocean, causing formation of iron (oxyhydr)oxide (FeOx) (Hegler et al., 2008).
View in article
Hiemstra, T., Van Riemsdijk, W.H. (2009) A surface structural model for ferrihydrite I: Sites related to primary charge, molar mass, and mass density. Geochimica et Cosmochimica Acta 73, 4423–4436.

Ferrihydrite is more hydrated than hematite so the loss of loosely adsorbed water (<125 °C) and tightly bound water (125–700 °C) is higher for ferrihydrite than hematite (Hiemstra and Van Riemsdijk, 2009).
View in article
Israelachvili, J.N. (2011) Intermolecular and Surface Forces. Third edition, Elsevier, Burlington.

These binding energies suggest that the interaction is dominated by strong Van der Waals or weak electrostatic forces (Israelachvili, 2011).
View in article
Jelavić, S., Tobler, D.J., Hassenkam, T., Yoreo, J.J.D., Stipp, S.L.S., Sand, K.K. (2017) Prebiotic RNA polymerisation: energetics of nucleotide adsorption and polymerisation on clay mineral surfaces. Chemical Communications 53, 12700–12703.

Tips were functionalised following the protocol described in Jelavić et al. (2017) (details in SI).
View in article
The specular hematite monocrystal {0001} face was cleaned prior to analysis following the protocol described in Jelavić et al. (2017) (details in the SI).
View in article
Kappler, A., Pasquero, C., Konhauser, K.O., Newman, D.K. (2005) Deposition of banded iron formations by anoxygenic phototrophic Fe(II)-oxidizing bacteria. Geology 33, 865–868.

An improved understanding of microbially induced Fe(II) oxidation highlights that oxidising bacteria, such as Rhodobacter ferrooxidans, could likely drive the formation of IFs (Kappler et al., 2005).
View in article
Kleber, M., Eusterhues, K., Keiluweit, M., Mikutta, C., Mikutta, R., Nico, P.S. (2015) Chapter One – Mineral–Organic Associations: Formation, Properties, and Relevance in Soil Environments. In: Sparks, D.L. (Ed.) Advances in Agronomy. Academic Press, Cambridge, 1–140.

In this scenario, organic compounds would have been released to solution where they could have been re-adsorbed but less strongly bound to newly formed phases (Chen et al., 2015), or released in the water column and subsequently degraded by various biotic and abiotic processes (Kleber et al., 2015).
View in article
Klein, C. (2005) Some Precambrian banded iron-formations (BIFs) from around the world: Their age, geologic setting, mineralogy, metamorphism, geochemistry, and origins. American Mineralogist 90, 1473–1499.

However, the low concentration of organic compounds in IFs and their diagenetic or metamorphic derivatives, challenges the hypothesis of their biogenic origin (Klein, 2005).
View in article
Koehler, I., Konhauser, K., Kappler, A. (2010) Role of Microorganisms in Banded Iron Formations. In: Barton, L.L., Mandl, M., Loy, A (Eds.) Geomicrobiology: Molecular and Environmental Perspective. Springer Netherlands, Dordrecht, 309–324.

Traditionally, iron formations (IFs) have been considered as abiotically generated chemical sediments but an increasing body of evidence suggests the active role of microbes in their precipitation (Koehler et al., 2010).
View in article
Köhler, I., Konhauser, K.O., Papineau, D., Bekker, A., Kappler, A. (2013) Biological carbon precursor to diagenetic siderite with spherical structures in iron formations. Nature Communications 4, 1–7.

Some of the organic compounds were degraded by diagenetic and metamorphic processes (Köhler et al., 2013; Posth et al., 2013; Halama et al., 2016).
View in article
Konhauser, K.O., Planavsky, N.J., Hardisty, D.S., Robbins, L.J., Warchola, T.J., Haugaard, R., Lalonde, S.V., Partin, C.A., Oonk, P.B.H., Tsikos, H., Lyons, T.W., Bekker, A., Johnson, C.M. (2017) Iron formations: A global record of Neoarchaean to Palaeoproterozoic environmental history. Earth-Science Reviews 172, 140–177.

Hematite is a major component of IFs found today (Konhauser et al., 2017) but, initially, the FeOx in the FeOx-polymer complexes were most likely composed of ferrihydrite (Chan et al., 2004).
View in article
Lützenkirchen, J., Preočanin, T., Stipić, F., Heberling, F., Rosenqvist, J., Kallay, N. (2013) Surface potential at the hematite (001) crystal plane in aqueous environments and the effects of prolonged aging in water. Geochimica et Cosmochimica Acta 120, 479–486.

We chose these conditions, rather than conditions at which the transformation experiments were conducted, to maximise the interaction forces between the tip and hematite surface (Lützenkirchen et al., 2013; Newcomb et al., 2017).
View in article
Mikutta, R., Lorenz, D., Guggenberger, G., Haumaier, L., Freund, A. (2014) Properties and reactivity of Fe-organic matter associations formed by coprecipitation versus adsorption: Clues from arsenate batch adsorption. Geochimica et Cosmochimica Acta 144, 258–276.

Coprecipitation of ferrihydrite-polymer aggregates results in a high organic content compared to adsorbed polymers (Mikutta et al., 2014) and can cause variations in grain size and aggregation.
View in article
Newcomb, C.J., Qafoku, N.P., Grate, J.W., Bailey, V.L., Yoreo, J.J.D. (2017) Developing a molecular picture of soil organic matter–mineral interactions by quantifying organo–mineral binding. Nature Communications 8, 396.

We chose these conditions, rather than conditions at which the transformation experiments were conducted, to maximise the interaction forces between the tip and hematite surface (Lützenkirchen et al., 2013; Newcomb et al., 2017).
View in article
Phoenix, V.R., Adams, D.G., Konhauser, K.O. (2000) Cyanobacterial viability during hydrothermal biomineralisation. Chemical Geology 169, 329–338.

This prevents the encrustation of the microbe itself (Phoenix et al., 2000; Chan et al., 2004).
View in article
Posth, N.R., Köhler, I., Swanner, E.D., Schröder, C., Wellmann, E., Binder, B., Konhauser, K.O., Neumann, U., Berthold, C., Nowak, M., Kappler, A. (2013) Simulating Precambrian banded iron formation diagenesis. Chemical Geology, Special Issue dedicated to H.D. Holland: Evolution of the atmosphere and ocean through time 362, 66–73.

Some of the organic compounds were degraded by diagenetic and metamorphic processes (Köhler et al., 2013; Posth et al., 2013; Halama et al., 2016).
View in article
This loss of organic compounds is probably further enhanced by the grain coarsening of hematite during diagenetic and metamorphic processes and concomitant reactions in the microenvironment that result in secondary mineralisation, e.g., Posth et al. (2013).
View in article
Sand, K.K., Jelavić, S., Dobberschütz, S., Ashby, P.D., Marshall, M.J., Dideriksen, K., Stipp, S.L.S., Kerisit, S.N., Friddle, R.W., DeYoreo, J.J. (2020) Mechanistic insight into biopolymer induced iron oxide mineralization through quantification of molecular bonding. Nanoscale Advances 2, 3323–3333.

Extracellular polymeric substances (EPS) promote FeOx nucleation (Sand et al., 2020) where microbially formed FeOx are often found in close association with the EPS (Chan et al., 2004).
View in article
However, the Gibbs free energy of binding (ΔGbu) between ferrihydrite and EPS is larger than between hematite and EPS (Sand et al., 2020) implying that the polymers were more likely to desorb from hematite than from ferrihydrite.
View in article
Schwertmann, U., Cornell, R.M. (2000) Ferrihydrite. In: Iron Oxides in the Laboratory. John Wiley & Sons, Ltd, Weinheim, 103–112.

Ferrihydrite was synthesised using the method of Schwertmann and Cornell (2000).
View in article
Schwertmann, U., Murad, E. (1983) Effect of pH on the Formation of Goethite and Hematite from Ferrihydrite. Clays and Clay Minerals 31, 277–284.

Transformation involving intermediary phases is a dissolution-precipitation process (Schwertmann and Murad, 1983) implying that the interface between ferrihydrite and organic compounds is eliminated.
View in article
Sun, S., Konhauser, K.O., Kappler, A., Li, Y.-L. (2015) Primary hematite in Neoarchean to Paleoproterozoic oceans. GSA Bulletin 127, 850–861.

The transformation to hematite would have happened in aqueous solutions both before (Sun et al., 2015) and after deposition on the seabed.
View in article
Trainor, T.P., Chaka, A.M., Eng, P.J., Newville, M., Waychunas, G.A., Catalano, J.G., Brown, G.E. (2004) Structure and reactivity of the hydrated hematite (0001) surface. Surface Science 573, 204–224.

Considering that the {0001} hematite surface is completely hydroxylated in water (Trainor et al., 2004), our results follow the trend where OH-OH bonds are twice as strong as COO−-OH bonds at circumneutral pH (Vezenov et al., 1997) present in Precambrian ocean.
View in article
van Oss, C.J. (1997) Hydrophobicity and hydrophilicity of biosurfaces. Current Opinion in Colloid & Interface Science 2, 503–512.

Adsorption of polymers is a complex function of degree of branching, length and hydrophobicity (van Oss, 1997).
View in article
Vezenov, D.V., Noy, A., Rozsnyai, L.F., Lieber, C.M. (1997) Force Titrations and Ionization State Sensitive Imaging of Functional Groups in Aqueous Solutions by Chemical Force Microscopy. Journal of the American Chemical Society 119, 2006–2015.

Considering that the {0001} hematite surface is completely hydroxylated in water (Trainor et al., 2004), our results follow the trend where OH-OH bonds are twice as strong as COO−-OH bonds at circumneutral pH (Vezenov et al., 1997) present in Precambrian ocean.
View in article
top
Supplementary Information
The Supplementary Information includes:
- Tip Functionalisation
- Cleaning of Hematite Substrate
- Dynamic Force Spectroscopy (DFS)
- The Effect of Temperature on ΔGbu
- Estimating the Adsorption Capacity of Ferrihydrite for Glycerol
- X-ray Diffraction (XRD)
- Estimation of the Specific Surface Area (SSA)
- Supplementary Information References
Download the Supplementary Information (PDF).
Figures and Tables
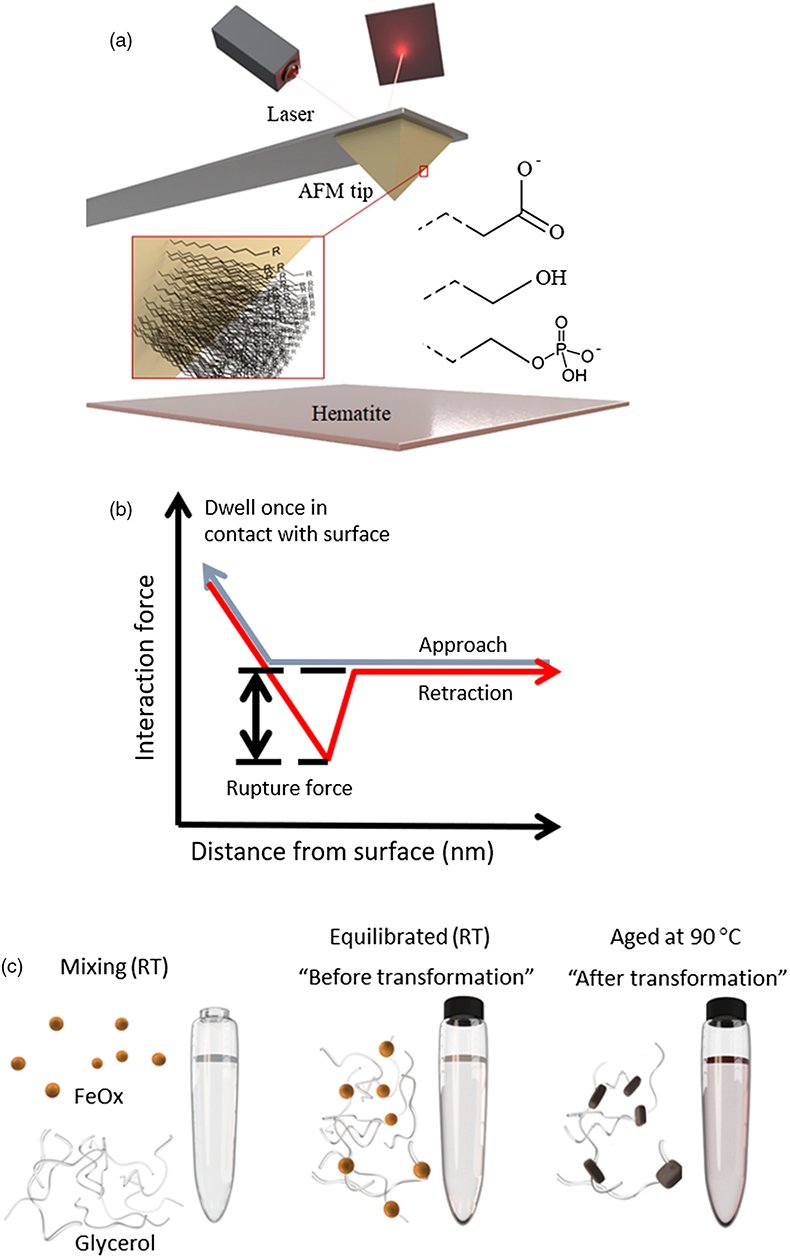
Figure 1 (a) Schematics of the DFS (Dynamic Force Spectroscopy): self assembled monolayers with carboxyl, hydroxyl and phosphate headgroups covalently bonded to AFM tip. (b) A scheme of a force curve. (c) In transformation experiments, ferrihydrite and glycerol were mixed at room temperature and left to equilibrate overnight. One sample was then taken for TGA (equilibrated sample) and the rest was placed in the oven at 90 °C until the transformation was complete (aged sample).

Figure 2 Multibond fit (Friddle et al., 2012
Friddle, R.W., Noy, A., Yoreo, J.J.D. (2012) Interpreting the widespread nonlinear force spectra of intermolecular bonds. Proceedings of the National Academy of Sciences 109, 13573–13578.
) to the dynamic force spectra between hematite surface and alkyl thiol self assembled monolayers with OH,
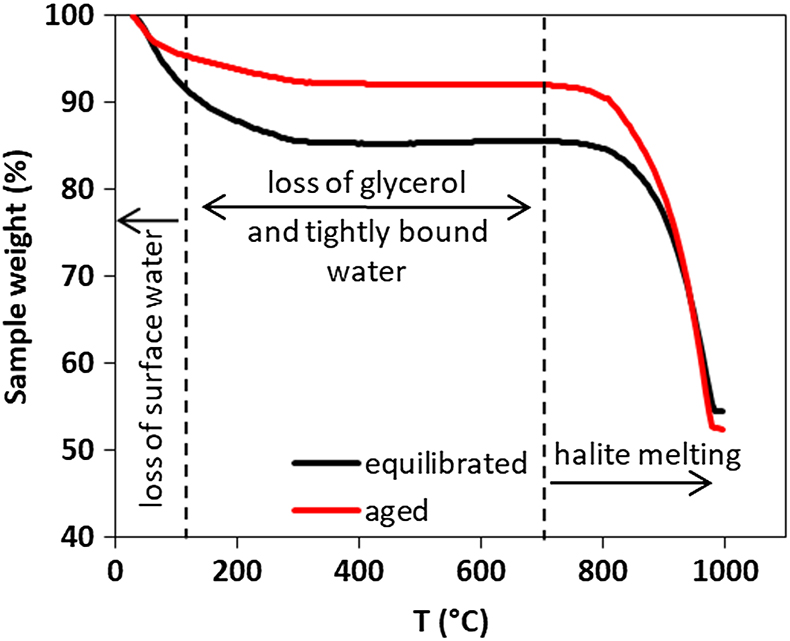
Figure 3 Comparison of thermogravimetry curves of ferrihydrite-glycerol (equilibrium-black curve) and resulting hematite-glycerol (aged-red curve). The smaller mass loss in the region 125–700 °C (dashed vertical lines) for the aged sample compared to the equilibrium sample suggests bigger loss of tightly bound water and glycerol during the transformation.