SHRIMP 4-S isotope systematics of two pyrite generations in the 3.49 Ga Dresser Formation
Affiliations | Corresponding Author | Cite as | Funding information- Share this article
-
Article views:434Cumulative count of HTML views and PDF downloads.
- Download Citation
- Rights & Permissions
top
Abstract
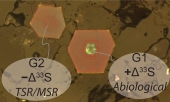
1Δ33S and Δ36S quantify the magnitudes of sulfur mass-independent fractionations (S-MIFs) during a reaction, which are the deviations of the 34S/32S, 33S/32S, and 36S/32S isotopic ratios from those expected solely based on their mass differences (see section 3.2 in the Supplementary Information).
Figures and Tables
![]() Figure 1 (a) BSE image of tiny pyrites aligned in an array within barite. (b) Photomicrograph (reflected light, NaOCl-etched pyrite) and (c–f) BSE images of core-rim textured pyrites. (a, c–f) are from PDP2c_97.17–97.20 m; (b) is from PDP2b_90.94–90.97 m. | ![]() Figure 2 (b) δ34S-Δ33S and (c) Δ33S-Δ36S plots for G1, G2, G2′ pyrites, with (a) BSE image of a Py-ii showing four generations. Archaean Reference Array: Δ36S ≈ −Δ33S (Farquhar et al., 2001); Biological Fractionation Line: Δ36S ≈ −7Δ33S (Ono et al., 2006). 1σ = [(SE)2 + (SD)2]0.5, where SE is standard error of the analysis, and SD is standard deviation of Ruttan pyrite in the associated session. | ![]() Figure 3 (δ34Ssulfide − δ34Ssulfate) – (Δ33S + Δ36S) plot for G2, along with the initial sulfates, MSR and TSR. A δ34Ssulfate value of 4.91 ‰ (the mean of Dresser barites) was used. Error bar 1σ of (δ34Ssulfide − δ34Ssulfate) is that of δ34Ssulfide (G2), and 1σ of (Δ33S + Δ36S) is (σΔ33S2 + σΔ36S2)0.5. | ![]() Table 1 Classification and quadruple S isotopic compositions of pyrites. |
Figure 1 | Figure 2 | Figure 3 | Table 1 |
top
Introduction
The 3.49 Ga Dresser Formation in the Pilbara Craton, Western Australia has been widely considered to preserve palaeontological and geochemical evidence of Earth’s earliest life (e.g., Buick et al., 1981
Buick, R., Dunlop, J.S.R., Groves, D.I. (1981) Stromatolite recognition in ancient rocks: an appraisal of irregularly laminated structures in an Early Archaean chert-barite unit from North Pole, Western Australia. Alcheringa 5, 161–181.
; Ueno et al., 2006Ueno, Y., Yamada, K., Yoshida, N., Maruyama, S., Isozaki, Y. (2006) Evidence from fluid inclusions for microbial methanogenesis in the early Archaean era. Nature 440, 516–519.
; Shen et al., 2009Shen, Y., Farquhar, J., Masterson, A., Kaufman, A.J., Buick, R. (2009) Evaluating the role of microbial sulfate reduction in the early Archean using quadruple isotope systematics. Earth and Planetary Science Letters 279, 383–391.
; Noffke et al., 2013Noffke, N., Christian, D., Wacey, D., Hazen, R.M. (2013) Microbially Induced Sedimentary Structures Recording an Ancient Ecosystem in the ca. 3.48 Billion-Year-Old Dresser Formation, Pilbara, Western Australia. Astrobiology 13, 1103–1124.
). The multiple sulfur isotopes of sulfate and sulfide in the Dresser Formation have been measured by both bulk and in situ analytical methods. Results of bulk methods support microbial sulfate reduction (MSR; Ueno et al., 2008Ueno, Y., Ono, S., Rumble, D., Maruyama, S. (2008) Quadruple sulfur isotope analysis of ca. 3.5 Ga Dresser Formation: New evidence for microbial sulfate reduction in the early Archean. Geochimica et Cosmochimica Acta 72, 5675–5691.
; Shen et al., 2009Shen, Y., Farquhar, J., Masterson, A., Kaufman, A.J., Buick, R. (2009) Evaluating the role of microbial sulfate reduction in the early Archean using quadruple isotope systematics. Earth and Planetary Science Letters 279, 383–391.
) while in situ measurements support elemental sulfur disproportionation (Philippot et al., 2007Philippot, P., Van Zuilen, M., Lepot, K., Thomazo, C., Farquhar, J., Van Kranendonk, M.J. (2007) Early Archaean Microorganisms Preferred Elemental Sulfur, Not Sulfate. Science 317, 1534–1537.
). The differences in these data sets are profound. Although recent in situ studies have confirmed separately the δ34S–Δ33S positively correlated Δ33S-negative (Baumgartner et al., 2020Baumgartner, R.J., Caruso, S., Fiorentini, M.L., Van Kranendonk, M.J., Martin, L., Jeon, H., Pagès, A., Wacey, D. (2020) Sulfidization of 3.48 billion-year-old stromatolites of the Dresser Formation, Pilbara Craton: Constraints from in-situ sulfur isotope analysis of pyrite. Chemical Geology 538, 119488.
) and Δ33S-positive (Wacey et al., 2015Wacey, D., Noffke, N., Cliff, J., Barley, M.E., Farquhar, J. (2015) Micro-scale quadruple sulfur isotope analysis of pyrite from the ∼3480 Ma Dresser Formation: New insights into sulfur cycling on the early Earth. Precambrian Research 258, 24–35.
) pyrites, the relationship between the two groups remains unknown.The chert-barite unit of Dresser Formation was generated by a complex hydrothermal system, thus the pyrites are likely to preserve multiple generations resulting from multiple stages of hydrothermal events (Van Kranendonk et al., 2008
Van Kranendonk, M.J., Philippot, P., Lepot, K., Bodorkos, S., Pirajno, F. (2008) Geological setting of Earth’s oldest fossils in the ca. 3.5 Ga Dresser Formation, Pilbara Craton, Western Australia. Precambrian Research 167, 93–124.
). This study established the generations of pyrite formation, and then measured the multiple sulfur isotopes in situ. We exploited the in situ capabilities of SHRIMP-SI allowing 36S measurements in pyrite with a precision at a similar level to conventional bulk methods. In this way, we uniquely identified the sulfur isotopic composition of each generation of pyrites, and provided constraints on their formation pathways.top
Geological Setting
The 3.49 Ga (Tessalina et al., 2010
Tessalina, S.G., Bourdon, B., Van Kranendonk, M., Birck, J.L., Philippot, P. (2010) Influence of Hadean crust evident in basalts and cherts from the Pilbara Craton. Nature Geoscience 3, 214–217.
) Dresser Formation crops out as a ring of the North Pole Dome in the Pilbara Craton. It consists of metabasalt interlayered with three chert horizons (Ueno et al., 2008Ueno, Y., Ono, S., Rumble, D., Maruyama, S. (2008) Quadruple sulfur isotope analysis of ca. 3.5 Ga Dresser Formation: New evidence for microbial sulfate reduction in the early Archean. Geochimica et Cosmochimica Acta 72, 5675–5691.
). The lowermost chert hosts abundant barite, referred to as the chert-barite unit, and is composed of chert, barite, carbonate, sandstone and conglomerate. Below this unit are thousands of silica ± sulfides (pyrite and sphalerite) ± organic matter veins and barite veins intruding and transecting the underlying komatiitic basalt. The chert-barite unit and the veins are generally considered to be formed in a volcanic caldera, with sedimentation accompanied by volcanism, hydrothermal circulation and precipitation, and tectonic activity (Van Kranendonk et al., 2008Van Kranendonk, M.J., Philippot, P., Lepot, K., Bodorkos, S., Pirajno, F. (2008) Geological setting of Earth’s oldest fossils in the ca. 3.5 Ga Dresser Formation, Pilbara Craton, Western Australia. Precambrian Research 167, 93–124.
). The hydrothermal fluids ascended along growth faults and deposited as concordant layers in a feeder-deposit manner. Detailed geological setting is in Supplementary Information (SI).top
Samples and Methods
The investigated samples are from two drill cores: PDP2b and PDP2c. Detailed sample descriptions are in Table S-1. The drill core sections were made into polished mounts, which were immersed in sodium hypochlorite solution (8–12.5 % NaOCl) to reveal the internal textures of pyrites. The principle of pyrite etching is in SI. Appropriate pyrites were selected for subsequent BSE (Back Scattered Electron) imaging, EDS (Energy Dispersive X-ray Spectroscopy) and Raman spectroscopy analyses. Then the pyrites were measured for multiple sulfur isotopes using SHRIMP-SI. Detailed methods are in SI.
top
Results
Pyrites occur as tiny grains aligned in an array within barite (Py-i), pyrite laminae (Py-ii), individual grains (IG) or clusters associated with silica veinlets/beads in barite (within veinlet, Py-iii; on the wall of veinlet, Py-iv; adjacent to both barite and chert, Py-v), IG in barite with silica veinlets/beads (Py-vi), IG in chert with barite crystal fans (Py-vii), and IG in silica-barite-carbonate veins in hydrothermally altered komatiitic basalt (Py-viii) (Fig. S-2). Some pyrites show core-rim textures (portions of Py-i to vi and Py-viii) while the others are homogeneous (Figs. 1, S-3). The sulfur isotopic composition exhibits evident dependence on the internal texture and petrography of pyrite. The cores show a tendency towards positive δ34S and Δ33S, whereas the rims have more negative δ34S and Δ33S (Fig. S-8). Py-ii are mostly core-rim textured and display both negative and positive δ34S, Δ33S and Δ36S, whereas Py-iii to vi are characterised by negative δ34S and Δ33S and positive Δ36S, with only a few exceptions. Py-vii show characteristic positive δ34S and Δ33S, and Py-viii exhibit negative Δ33S and positive δ34S (Table 1, Fig. S-9). Detailed petrography, internal texture, and sulfur isotopic compositions are in SI.
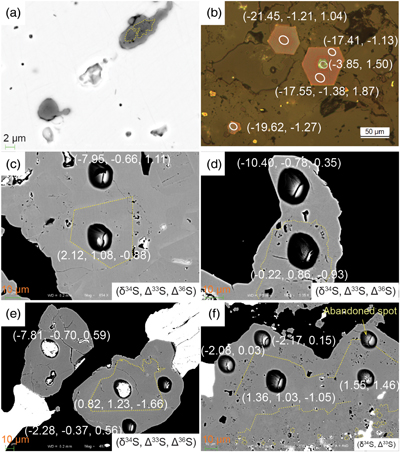
Figure 1 (a) BSE image of tiny pyrites aligned in an array within barite. (b) Photomicrograph (reflected light, NaOCl-etched pyrite) and (c–f) BSE images of core-rim textured pyrites. (a, c–f) are from PDP2c_97.17–97.20 m; (b) is from PDP2b_90.94–90.97 m.
Table 1 Classification and quadruple S isotopic compositions of pyrites.
Pyrite type | Description | δ34S (‰) | Δ33S (‰) | Δ36S (‰) | |
Py-i | Pyrites occur as tiny grains aligned in an array within barite. | ||||
Py-ii | Pyrite laminae. | −10.40 to 2.12 | −1.19 to 1.46 | −2.14 to 1.11 | |
Py-iii | Individual grains or clusters associated with silica veinlets/beads in barite | within veinlet | −21.45 to −0.79 | −1.38 to 1.50 | −0.31 to 1.87 |
Py-iv | on the wall of veinlet | ||||
Py-v | adjacent to both barite and chert | ||||
Py-vi | Individual grains in barite with silica veinlets/beads | −18.99 to −1.54 | −1.11 to 0.10 | −0.16 to 1.20 | |
Py-vii | Individual grains in chert with barite crystal fans | 2.81 to 5.09 | 2.52 to 3.62 | −2.92 to −2.63 | |
Py-viii | Individual grains in silica-barite-carbonate vein in hydrothermally altered komatiitic basalt | 1.67 to 2.39 | −1.01 to −0.67 |
top
Discussion
The core-rim relationship combined with sulfur isotopic composition reveals two main generations (Δ33S-positive, euhedral to subhedral, locally porous G1 and Δ33S-negative, anhedral G2) in the core-rim textured pyrites. The majority have Δ33S-positive cores and Δ33S-negative rims, indicating that Δ33S-positive precede Δ33S-negative pyrites. Δ33S-0 (close to 0, considering uncertainties) pyrites are widespread, and can be formed via the sulfide pathway (Fe2+ + S2– = [FeS], [FeS] + H2S = FeS2 + H2; Rickard and Luther, 1997
Rickard, D., Luther, G.W. (1997) Kinetics of pyrite formation by the H2S oxidation of iron (II) monosulfide in aqueous solutions between 25 and 125 °C: The mechanism. Geochimica et Cosmochimica Acta 61, 135–147.
) with magmatic H2S abundant in the Dresser hydrothermal system throughout the multiple stages of hydrothermal events (Ueno et al., 2008Ueno, Y., Ono, S., Rumble, D., Maruyama, S. (2008) Quadruple sulfur isotope analysis of ca. 3.5 Ga Dresser Formation: New evidence for microbial sulfate reduction in the early Archean. Geochimica et Cosmochimica Acta 72, 5675–5691.
and references therein). Δ33S-0 pyrites can be synchronous with Δ33S-positive and Δ33S-negative pyrites, because both Δ33S-positive and Δ33S-negative pyrites show evident positive correlations between δ34S and Δ33S (Fig. 2b), indicating two separate mixing trends between two respective end members, with one being Δ33S-0. Minor pyrites show Δ33S-negative cores and rims, suggesting two stages of G2.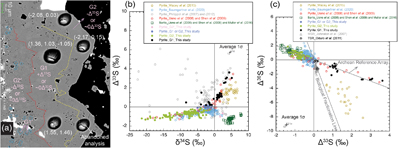
Figure 2 (b) δ34S-Δ33S and (c) Δ33S-Δ36S plots for G1, G2, G2′ pyrites, with (a) BSE image of a Py-ii showing four generations. Archaean Reference Array: Δ36S ≈ −Δ33S (Farquhar et al., 2001
Farquhar, J., Savarino, J., Airieau, S., Thiemens, M.H. (2001) Observation of wavelength-sensitive mass-independent sulfur isotope effects during SO2 photolysis: Implications for the early atmosphere. Journal of Geophysical Research 106, 32829–32839.
); Biological Fractionation Line: Δ36S ≈ −7Δ33S (Ono et al., 2006Ono, S., Wing, B., Johnston, D., Farquhar, J., Rumble, D. (2006) Mass-dependent fractionation of quadruple stable sulfur isotope system as a new tracer of sulfur biogeochemical cycles. Geochimica et Cosmochimica Acta 70, 2238–2252.
). 1σ = [(SE)2 + (SD)2]0.5, where SE is standard error of the analysis, and SD is standard deviation of Ruttan pyrite in the associated session.Some Py-ii show two additional growth zones prior to G1 and G2 (Fig. 2a). The earlier zones (G1′) are tiny porous grains, the Δ33S of which is beyond the resolution ability of SHRIMP spots. However, the textures of these pyrites are similar to the cores of core-rim textured Py-i (Fig. 1a) that have been shown to be Δ33S-positive (Philippot et al., 2007
Philippot, P., Van Zuilen, M., Lepot, K., Thomazo, C., Farquhar, J., Van Kranendonk, M.J. (2007) Early Archaean Microorganisms Preferred Elemental Sulfur, Not Sulfate. Science 317, 1534–1537.
). The later zones (G2′) are partly oscillatory zoned and porous, and these have been measured by Baumgartner et al. (2020)Baumgartner, R.J., Caruso, S., Fiorentini, M.L., Van Kranendonk, M.J., Martin, L., Jeon, H., Pagès, A., Wacey, D. (2020) Sulfidization of 3.48 billion-year-old stromatolites of the Dresser Formation, Pilbara Craton: Constraints from in-situ sulfur isotope analysis of pyrite. Chemical Geology 538, 119488.
and are mostly Δ33S-negative. One Py-ii (Fig. S-3d) has a Δ33S-negative core (oscillatory zoned) and Δ33S-positive rim, confirming G2′ precedes G1. Collectively, four generations are recorded in core-rim textured pyrites: Δ33S-positive G1′, Δ33S-negative G2′ (Baumgartner et al., 2020Baumgartner, R.J., Caruso, S., Fiorentini, M.L., Van Kranendonk, M.J., Martin, L., Jeon, H., Pagès, A., Wacey, D. (2020) Sulfidization of 3.48 billion-year-old stromatolites of the Dresser Formation, Pilbara Craton: Constraints from in-situ sulfur isotope analysis of pyrite. Chemical Geology 538, 119488.
), Δ33S-positive G1 and Δ33S-negative G2 (this study). The multiple generations of pyrites in the same sample can be attributed to multiple pulses of hydrothermal fluids, with the later generation overgrown on the earlier generation.The pyrites with no internal texture (Py-ii to vi) have the same petrography as the core-rim textured counterparts, and show similar multiple sulfur isotope systematics to the cores and rims, thus the homogeneous pyrites (Py-ii to vi) are also categorised into the four generations based on Δ33S and internal texture. The homogeneous Py-ii to vi lack the characteristic textures of G1′ and G2′, thus are all classified to G1 and G2. Δ33S-positive Py-vii are Ni-free and not porous, distinct from G1′ while similar to G1. The negative Δ33S and positive δ34S of Py-viii are similar to some G2′.
Photochemical reactions of gaseous sulfur species have been widely proposed to produce S-MIF, and the products are Δ33S-positive elemental sulfur and Δ33S-negative sulfate (Ono, 2017
Ono, S. (2017) Photochemistry of Sulfur Dioxide and the Origin of Mass-Independent Isotope Fractionation in Earth’s Atmosphere. Annual Review of Earth and Planetary Sciences 45, 301–329.
and references therein). Δ33S-positive G1 and Δ33S-negative barites yield a line of Δ36S = −0.98Δ33S + 0.05 (Fig. S-10a). The slope is close to that for products of SO2 photolysis under 193 nm UV (Farquhar et al., 2001Farquhar, J., Savarino, J., Airieau, S., Thiemens, M.H. (2001) Observation of wavelength-sensitive mass-independent sulfur isotope effects during SO2 photolysis: Implications for the early atmosphere. Journal of Geophysical Research 106, 32829–32839.
). Thus the photochemical products sulfate and elemental sulfur are likely to have been mostly sequestered in barites and G1 pyrites via reactions with Ba2+-rich fluids and Fe2+-, S2−-rich fluids, respectively, with the magnitudes diluted by magmatic and/or Δ33S-opposite sign sulfur species in the atmosphere/water mass (detailed explanation in SI).G1 yield a line of Δ36S = −0.86Δ33S − 0.20 (Fig. S-10b) close to the Archean Reference Array (ARA). Some G1 show slight deviations from ARA, both upwards and downwards (one analysis exhibits a downward offset of >1 ‰), suggesting possible microbial elemental sulfur disproportionation or sulfate reduction, respectively (Johnston et al., 2007
Johnston, D.T., Farquhar, J., Canfield, D.E. (2007) Sulfur isotope insights into microbial sulfate reduction: When microbes meet models. Geochimica et Cosmochimica Acta 71, 3929–3947.
). However, neither is likely since microbial activities prefer 32S over 34S (i.e. negative δ34S) while G1 have positive δ34S.G2 are most likely formed via the sulfide pathway, with S2– derived from sulfate reduction. In addition to the sulfate in fluids and water mass, barite is also a possible sulfate source. G2 are closely associated with barites (e.g., G2 occur within barites, in the silica veinlets of barites, or contain barite residue), and have similar Δ33S despite smaller magnitudes. The solubility of barite can be increased by NaCl (Shi et al., 2012
Shi, W., Kan, A.T., Fan, C., Tomson, M.B. (2012) Solubility of Barite up to 250 °C and 1500 bar in up to 6 m NaCl Solution. Industrial and Engineering Chemistry Research 51, 3119–3128.
) and silica (Cui et al., 2019Cui, H., Zhong, R., Xie, Y., Yuan, X., Liu, W., Brugger, J., Yu, C. (2019) Forming sulfate- and REE-rich fluids in the presence of quartz. Geology 48, 145–148.
) that are present in the Dresser hydrothermal fluids (Harris et al., 2009Harris, A.C., White, N.C., McPhie, J., Bull, S.W., Line, M.A., Skrzeczynski, R., Mernagh, T.P., Tosdal, R.M. (2009) Early Archean Hot Springs above Epithermal Veins, North Pole, Western Australia: New Insights from Fluid Inclusion Microanalysis. Economic Geology 104, 793–814.
). Therefore, G2 can be derived from barite reduction, with Fe2+ and reductants brought in by hydrothermal fluids penetrated into barites as indicated by the widespread silica veinlets in barites. Δ33S of G2 can have been diluted by (1) the involvement of magmatic H2S/HS–/S2– during pyrite formation via the sulfide pathway as indicated by the positive correlation between negative δ34S and Δ33S (Fig. 2b), and (2) the positive Δ33S induced by sulfate reduction (thermochemical sulfate reduction, TSR; Oduro et al., 2011Oduro, H., Harms, B., Sintim, H.O., Kaufman, A.J., Cody, G., Farquhar, J. (2011) Evidence of magnetic isotope effects during thermochemical sulfate reduction. Proceedings of the National Academy of Sciences 108, 17635–17638.
; MSR, Johnston et al., 2007Johnston, D.T., Farquhar, J., Canfield, D.E. (2007) Sulfur isotope insights into microbial sulfate reduction: When microbes meet models. Geochimica et Cosmochimica Acta 71, 3929–3947.
).The essential effects of MSR are the depletions in 34S and downward shifts in Δ36S/Δ33S (i.e. lower (Δ33S + Δ36S)2 values) relative to the initial sulfate (Johnston et al., 2007
Johnston, D.T., Farquhar, J., Canfield, D.E. (2007) Sulfur isotope insights into microbial sulfate reduction: When microbes meet models. Geochimica et Cosmochimica Acta 71, 3929–3947.
). Previous bulk analyses prefer MSR based on such signatures (Ueno et al., 2008Ueno, Y., Ono, S., Rumble, D., Maruyama, S. (2008) Quadruple sulfur isotope analysis of ca. 3.5 Ga Dresser Formation: New evidence for microbial sulfate reduction in the early Archean. Geochimica et Cosmochimica Acta 72, 5675–5691.
; Shen et al., 2009Shen, Y., Farquhar, J., Masterson, A., Kaufman, A.J., Buick, R. (2009) Evaluating the role of microbial sulfate reduction in the early Archean using quadruple isotope systematics. Earth and Planetary Science Letters 279, 383–391.
). Admittedly, some G2 show negative (Δ33S + Δ36S) (−0.03 to −0.67 ‰) and δ34S fractionation of −7 to −26 ‰ from sulfate (the composition of sulfate is represented by that of barite), with portions plotted within the MSR area defined by culture experiments with sulfate reducers (2–36 °C; Figs. 2c, 3). However, the negative (Δ33S + Δ36S) of the MSR area are relative to (Δ33S + Δ36S) of 0 for initial sulfate. Bulk barite data are homogeneous with a restricted range of (Δ33S + Δ36S) near 0 (Ueno et al., 2008Ueno, Y., Ono, S., Rumble, D., Maruyama, S. (2008) Quadruple sulfur isotope analysis of ca. 3.5 Ga Dresser Formation: New evidence for microbial sulfate reduction in the early Archean. Geochimica et Cosmochimica Acta 72, 5675–5691.
; Shen et al., 2009Shen, Y., Farquhar, J., Masterson, A., Kaufman, A.J., Buick, R. (2009) Evaluating the role of microbial sulfate reduction in the early Archean using quadruple isotope systematics. Earth and Planetary Science Letters 279, 383–391.
), while in situ analyses results are variable with (Δ33S + Δ36S) ranging from −0.60 to 0.92 ‰ (Muller et al., 2016Muller, É., Philippot, P., Rollion-Bard, C., Cartigny, P. (2016) Multiple sulfur-isotope signatures in Archean sulfates and their implications for the chemistry and dynamics of the early atmosphere. Proceedings of the National Academy of Sciences 113, 7432–7437.
; Fig. 3). The (Δ33S + Δ36S) range of G2 is similar to that of barites (in situ data, Fig. 3), and no significant downward shifts of G2 relative to initial barite are observed. Additionally, barites and G2 pyrites yield a line of Δ36S = −0.99Δ33S − 0.02 close to ARA while distinct from the Biological Fractionation Line (BFL; Fig. S-10c). Therefore, MSR signal in G2 is weak, although we do not exclude its presence because two data points of Shen et al. (2009)Shen, Y., Farquhar, J., Masterson, A., Kaufman, A.J., Buick, R. (2009) Evaluating the role of microbial sulfate reduction in the early Archean using quadruple isotope systematics. Earth and Planetary Science Letters 279, 383–391.
show negative (Δ33S + Δ36S) even relative to the lowest (Δ33S + Δ36S) value of in situ barite data.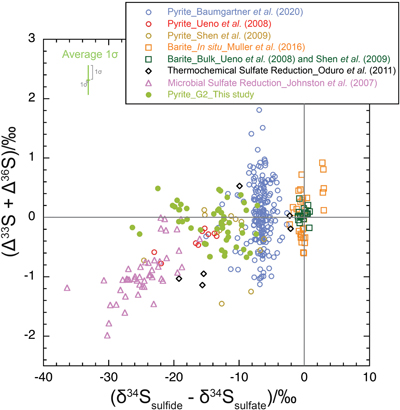
Figure 3 (δ34Ssulfide − δ34Ssulfate) – (Δ33S + Δ36S) plot for G2, along with the initial sulfates, MSR and TSR. A δ34Ssulfate value of 4.91 ‰ (the mean of Dresser barites) was used. Error bar 1σ of (δ34Ssulfide − δ34Ssulfate) is that of δ34Ssulfide (G2), and 1σ of (Δ33S + Δ36S) is (σΔ33S2 + σΔ36S2)0.5.
Furthermore, some G2 show positive (Δ33S + Δ36S) with larger magnitudes of positive shifts in Δ33S than negative shifts in Δ36S, which has not been observed in MSR but has been discovered in TSR (Figs. 2c, 3). TSR can induce positive and negative (Δ33S + Δ36S) depending on reductant and temperature (Oduro et al., 2011
Oduro, H., Harms, B., Sintim, H.O., Kaufman, A.J., Cody, G., Farquhar, J. (2011) Evidence of magnetic isotope effects during thermochemical sulfate reduction. Proceedings of the National Academy of Sciences 108, 17635–17638.
), in addition to significant depletions in δ34S relative to initial sulfate (Ohmoto and Goldhaber, 1997Ohmoto, H., Goldhaber, M.B. (1997) Sulfur and carbon isotopes. In: Barnes, H.L. (Ed.) Geochemistry of Hydrothermal Ore Deposits. Wiley, New York, 517–611.
). Therefore, the S2− of G2 can also be derived from TSR apart from MSR. TSR could be in operation 3.49 Ga ago in the Dresser hydrothermal system. This is consistent with the highest temperature of 110 °C where sulfate reducers (discovered so far) can live (Jørgensen et al., 1992Jørgensen, B.B., Isaksen, M.F., Jannasch, H.W. (1992) Bacterial Sulfate Reduction Above 100 °C in Deep-Sea Hydrothermal Vent Sediments. Science 258, 1756–1757.
) while the temperature of the hydrothermal fluids associated with the Dresser Formation ranges from 300 °C at depth to 120 °C near the palaeosurface (Harris et al., 2009Harris, A.C., White, N.C., McPhie, J., Bull, S.W., Line, M.A., Skrzeczynski, R., Mernagh, T.P., Tosdal, R.M. (2009) Early Archean Hot Springs above Epithermal Veins, North Pole, Western Australia: New Insights from Fluid Inclusion Microanalysis. Economic Geology 104, 793–814.
).top
Conclusions
A combination of sodium hypochlorite etching, BSE imaging and multiple sulfur isotopes reveals two main generations of pyrites associated with the barites of the 3.49 Ga Dresser Formation: Δ33S-positive G1 and δ34S- and Δ33S-negative G2. Diluted sulfate and elemental sulfur produced by photochemical reactions were sequestered in barites and G1, respectively. The positive δ34S of G1 indicates an abiological genesis. G2 were formed via the sulfide pathway, with the sulfur derived from magmatic H2S and S2− produced by thermochemical and possible microbial sulfate reduction.
2(Δ33S + Δ36S) is derived from Δ36S ≈ −Δ33S (ARA),
top
Acknowledgements
We sincerely thank the Perth Core Library for approving the access to drill cores and publishing the results. This work was supported by the Australian Research Council DP140103393 to TRI. The constructive comments from Dr. Boswell Wing and two anonymous reviewers are sincerely appreciated, which have improved this manuscript significantly.
Editor: Karim Benzerara
top
References
Baumgartner, R.J., Caruso, S., Fiorentini, M.L., Van Kranendonk, M.J., Martin, L., Jeon, H., Pagès, A., Wacey, D. (2020) Sulfidization of 3.48 billion-year-old stromatolites of the Dresser Formation, Pilbara Craton: Constraints from in-situ sulfur isotope analysis of pyrite. Chemical Geology 538, 119488.

The later zones (G2′) are partly oscillatory zoned and porous, and these have been measured by Baumgartner et al. (2020) and are mostly Δ33S-negative. One Py-ii (Fig. S-3d) has a Δ33S-negative core (oscillatory zoned) and Δ33S-positive rim, confirming G2′ precedes G1.
View in article
Collectively, four generations are recorded in core-rim textured pyrites: Δ33S-positive G1′, Δ33S-negative G2′ (Baumgartner et al., 2020), Δ33S-positive G1 and Δ33S-negative G2 (this study).
View in article
The differences in these data sets are profound. Although recent in situ studies have confirmed separately the δ34S–Δ33S positively correlated Δ33S-negative (Baumgartner et al., 2020) and Δ33S-positive (Wacey et al., 2015) pyrites, the relationship between the two groups remains unknown.
View in article
Buick, R., Dunlop, J.S.R., Groves, D.I. (1981) Stromatolite recognition in ancient rocks: an appraisal of irregularly laminated structures in an Early Archaean chert-barite unit from North Pole, Western Australia. Alcheringa 5, 161–181.

The 3.49 Ga Dresser Formation in the Pilbara Craton, Western Australia has been widely considered to preserve palaeontological and geochemical evidence of Earth’s earliest life (e.g., Buick et al., 1981; Ueno et al., 2006; Shen et al., 2009; Noffke et al., 2013).
View in article
Cui, H., Zhong, R., Xie, Y., Yuan, X., Liu, W., Brugger, J., Yu, C. (2019) Forming sulfate- and REE-rich fluids in the presence of quartz. Geology 48, 145–148.

The solubility of barite can be increased by NaCl (Shi et al., 2012) and silica (Cui et al., 2019) that are present in the Dresser hydrothermal fluids (Harris et al., 2009).
View in article
Farquhar, J., Savarino, J., Airieau, S., Thiemens, M.H. (2001) Observation of wavelength-sensitive mass-independent sulfur isotope effects during SO2 photolysis: Implications for the early atmosphere. Journal of Geophysical Research 106, 32829–32839.

The slope is close to that for products of SO2 photolysis under 193 nm UV (Farquhar et al., 2001).
View in article
(b) δ34S-Δ33S and (c) Δ33S-Δ36S plots for G1, G2, G2′ pyrites, with (a) BSE image of a Py-ii showing four generations. Archaean Reference Array: Δ36S ≈ −Δ33S (Farquhar et al., 2001); Biological Fractionation Line: Δ36S ≈ −7Δ33S (Ono et al., 2006).
View in article
Harris, A.C., White, N.C., McPhie, J., Bull, S.W., Line, M.A., Skrzeczynski, R., Mernagh, T.P., Tosdal, R.M. (2009) Early Archean Hot Springs above Epithermal Veins, North Pole, Western Australia: New Insights from Fluid Inclusion Microanalysis. Economic Geology 104, 793–814.

This is consistent with the highest temperature of 110 °C where sulfate reducers (discovered so far) can live (Jørgensen et al., 1992) while the temperature of the hydrothermal fluids associated with the Dresser Formation ranges from 300 °C at depth to 120 °C near the palaeosurface (Harris et al., 2009).
View in article
The solubility of barite can be increased by NaCl (Shi et al., 2012) and silica (Cui et al., 2019) that are present in the Dresser hydrothermal fluids (Harris et al., 2009).
View in article
Johnston, D.T., Farquhar, J., Canfield, D.E. (2007) Sulfur isotope insights into microbial sulfate reduction: When microbes meet models. Geochimica et Cosmochimica Acta 71, 3929–3947.

Some G1 show slight deviations from ARA, both upwards and downwards (one analysis exhibits a downward offset of >1 ‰), suggesting possible microbial elemental sulfur disproportionation or sulfate reduction, respectively (Johnston et al., 2007).
View in article
The essential effects of MSR are the depletions in 34S and downward shifts in Δ36S/Δ33S (i.e. lower (Δ33S + Δ36S)2 values) relative to the initial sulfate (Johnston et al., 2007).
View in article
Δ33S of G2 can have been diluted by (1) the involvement of magmatic H2S/HS–/S2– during pyrite formation via the sulfide pathway as indicated by the positive correlation between negative δ34S and Δ33S (Fig. 2b), and (2) the positive Δ33S induced by sulfate reduction (thermochemical sulfate reduction, TSR; Oduro et al., 2011; MSR, Johnston et al., 2007).
View in article
Jørgensen, B.B., Isaksen, M.F., Jannasch, H.W. (1992) Bacterial Sulfate Reduction Above 100 °C in Deep-Sea Hydrothermal Vent Sediments. Science 258, 1756–1757.

This is consistent with the highest temperature of 110 °C where sulfate reducers (discovered so far) can live (Jørgensen et al., 1992) while the temperature of the hydrothermal fluids associated with the Dresser Formation ranges from 300 °C at depth to 120 °C near the palaeosurface (Harris et al., 2009).
View in article
Muller, É., Philippot, P., Rollion-Bard, C., Cartigny, P. (2016) Multiple sulfur-isotope signatures in Archean sulfates and their implications for the chemistry and dynamics of the early atmosphere. Proceedings of the National Academy of Sciences 113, 7432–7437.

However, the negative (Δ33S + Δ36S) of the MSR area are relative to (Δ33S + Δ36S) of 0 for initial sulfate. Bulk barite data are homogeneous with a restricted range of (Δ33S + Δ36S) near 0 (Ueno et al., 2008; Shen et al., 2009), while in situ analyses results are variable with (Δ33S + Δ36S) ranging from −0.60 to 0.92 ‰ (Muller et al., 2016; Fig. 3).
View in article
Noffke, N., Christian, D., Wacey, D., Hazen, R.M. (2013) Microbially Induced Sedimentary Structures Recording an Ancient Ecosystem in the ca. 3.48 Billion-Year-Old Dresser Formation, Pilbara, Western Australia. Astrobiology 13, 1103–1124.

The 3.49 Ga Dresser Formation in the Pilbara Craton, Western Australia has been widely considered to preserve palaeontological and geochemical evidence of Earth’s earliest life (e.g., Buick et al., 1981; Ueno et al., 2006; Shen et al., 2009; Noffke et al., 2013).
View in article
Oduro, H., Harms, B., Sintim, H.O., Kaufman, A.J., Cody, G., Farquhar, J. (2011) Evidence of magnetic isotope effects during thermochemical sulfate reduction. Proceedings of the National Academy of Sciences 108, 17635–17638.

Δ33S of G2 can have been diluted by (1) the involvement of magmatic H2S/HS–/S2– during pyrite formation via the sulfide pathway as indicated by the positive correlation between negative δ34S and Δ33S (Fig. 2b), and (2) the positive Δ33S induced by sulfate reduction (thermochemical sulfate reduction, TSR; Oduro et al., 2011; MSR, Johnston et al., 2007).
View in article
TSR can induce positive and negative (Δ33S + Δ36S) depending on reductant and temperature (Oduro et al., 2011), in addition to significant depletions in δ34S relative to initial sulfate (Ohmoto and Goldhaber, 1997).
View in article
Ohmoto, H., Goldhaber, M.B. (1997) Sulfur and carbon isotopes. In: Barnes, H.L. (Ed.) Geochemistry of Hydrothermal Ore Deposits. Wiley, New York, 517–611.

TSR can induce positive and negative (Δ33S + Δ36S) depending on reductant and temperature (Oduro et al., 2011), in addition to significant depletions in δ34S relative to initial sulfate (Ohmoto and Goldhaber, 1997).
View in article
Ono, S. (2017) Photochemistry of Sulfur Dioxide and the Origin of Mass-Independent Isotope Fractionation in Earth’s Atmosphere. Annual Review of Earth and Planetary Sciences 45, 301–329.

Photochemical reactions of gaseous sulfur species have been widely proposed to produce S-MIF, and the products are Δ33S-positive elemental sulfur and Δ33S-negative sulfate (Ono, 2017 and references therein).
View in article
Ono, S., Wing, B., Johnston, D., Farquhar, J., Rumble, D. (2006) Mass-dependent fractionation of quadruple stable sulfur isotope system as a new tracer of sulfur biogeochemical cycles. Geochimica et Cosmochimica Acta 70, 2238–2252.

(b) δ34S-Δ33S and (c) Δ33S-Δ36S plots for G1, G2, G2′ pyrites, with (a) BSE image of a Py-ii showing four generations. Archaean Reference Array: Δ36S ≈ −Δ33S (Farquhar et al., 2001); Biological Fractionation Line: Δ36S ≈ −7Δ33S (Ono et al., 2006).
View in article
Philippot, P., Van Zuilen, M., Lepot, K., Thomazo, C., Farquhar, J., Van Kranendonk, M.J. (2007) Early Archaean Microorganisms Preferred Elemental Sulfur, Not Sulfate. Science 317, 1534–1537.

However, the textures of these pyrites are similar to the cores of core-rim textured Py-i (Fig. 1a) that have been shown to be Δ33S-positive (Philippot et al., 2007).
View in article
Results of bulk methods support microbial sulfate reduction (MSR; Ueno et al., 2008; Shen et al., 2009) while in situ measurements support elemental sulfur disproportionation (Philippot et al., 2007).
View in article
Philippot, P., van Zuilen, M., Rollion-Bard, C. (2012) Variations in atmospheric sulphur chemistry on early Earth linked to volcanic activity. Nature Geoscience 5, 668–674.

Figure 2View in article
Rickard, D., Luther, G.W. (1997) Kinetics of pyrite formation by the H2S oxidation of iron (II) monosulfide in aqueous solutions between 25 and 125 °C: The mechanism. Geochimica et Cosmochimica Acta 61, 135–147.

Δ33S-0 (close to 0, considering uncertainties) pyrites are widespread, and can be formed via the sulfide pathway (Fe2+ + S2– = [FeS], [FeS] + H2S = FeS2 + H2; Rickard and Luther, 1997) with magmatic H2S abundant in the Dresser hydrothermal system throughout the multiple stages of hydrothermal events (Ueno et al., 2008 and references therein).
View in article
Shen, Y., Farquhar, J., Masterson, A., Kaufman, A.J., Buick, R. (2009) Evaluating the role of microbial sulfate reduction in the early Archean using quadruple isotope systematics. Earth and Planetary Science Letters 279, 383–391.

Results of bulk methods support microbial sulfate reduction (MSR; Ueno et al., 2008; Shen et al., 2009) while in situ measurements support elemental sulfur disproportionation (Philippot et al., 2007).
View in article
Previous bulk analyses prefer MSR based on such signatures (Ueno et al., 2008; Shen et al., 2009).
View in article
However, the negative (Δ33S + Δ36S) of the MSR area are relative to (Δ33S + Δ36S) of 0 for initial sulfate. Bulk barite data are homogeneous with a restricted range of (Δ33S + Δ36S) near 0 (Ueno et al., 2008; Shen et al., 2009), while in situ analyses results are variable with (Δ33S + Δ36S) ranging from −0.60 to 0.92 ‰ (Muller et al., 2016; Fig. 3).
View in article
Therefore, MSR signal in G2 is weak, although we do not exclude its presence because two data points of Shen et al. (2009) show negative (Δ33S + Δ36S) even relative to the lowest (Δ33S + Δ36S) value of in situ barite data.
View in article
The 3.49 Ga Dresser Formation in the Pilbara Craton, Western Australia has been widely considered to preserve palaeontological and geochemical evidence of Earth’s earliest life (e.g., Buick et al., 1981; Ueno et al., 2006; Shen et al., 2009; Noffke et al., 2013).
View in article
Shi, W., Kan, A.T., Fan, C., Tomson, M.B. (2012) Solubility of Barite up to 250 °C and 1500 bar in up to 6 m NaCl Solution. Industrial and Engineering Chemistry Research 51, 3119–3128.

The solubility of barite can be increased by NaCl (Shi et al., 2012) and silica (Cui et al., 2019) that are present in the Dresser hydrothermal fluids (Harris et al., 2009).
View in article
Tessalina, S.G., Bourdon, B., Van Kranendonk, M., Birck, J.L., Philippot, P. (2010) Influence of Hadean crust evident in basalts and cherts from the Pilbara Craton. Nature Geoscience 3, 214–217.

The 3.49 Ga (Tessalina et al., 2010) Dresser Formation crops out as a ring of the North Pole Dome in the Pilbara Craton. It consists of metabasalt interlayered with three chert horizons (Ueno et al., 2008).
View in article
Ueno, Y., Yamada, K., Yoshida, N., Maruyama, S., Isozaki, Y. (2006) Evidence from fluid inclusions for microbial methanogenesis in the early Archaean era. Nature 440, 516–519.

The 3.49 Ga Dresser Formation in the Pilbara Craton, Western Australia has been widely considered to preserve palaeontological and geochemical evidence of Earth’s earliest life (e.g., Buick et al., 1981; Ueno et al., 2006; Shen et al., 2009; Noffke et al., 2013).
View in article
Ueno, Y., Ono, S., Rumble, D., Maruyama, S. (2008) Quadruple sulfur isotope analysis of ca. 3.5 Ga Dresser Formation: New evidence for microbial sulfate reduction in the early Archean. Geochimica et Cosmochimica Acta 72, 5675–5691.

Results of bulk methods support microbial sulfate reduction (MSR; Ueno et al., 2008; Shen et al., 2009) while in situ measurements support elemental sulfur disproportionation (Philippot et al., 2007).
View in article
The 3.49 Ga (Tessalina et al., 2010) Dresser Formation crops out as a ring of the North Pole Dome in the Pilbara Craton. It consists of metabasalt interlayered with three chert horizons (Ueno et al., 2008).
View in article
Previous bulk analyses prefer MSR based on such signatures (Ueno et al., 2008; Shen et al., 2009).
View in article
However, the negative (Δ33S + Δ36S) of the MSR area are relative to (Δ33S + Δ36S) of 0 for initial sulfate. Bulk barite data are homogeneous with a restricted range of (Δ33S + Δ36S) near 0 (Ueno et al., 2008; Shen et al., 2009), while in situ analyses results are variable with (Δ33S + Δ36S) ranging from −0.60 to 0.92 ‰ (Muller et al., 2016; Fig. 3).
View in article
Δ33S-0 (close to 0, considering uncertainties) pyrites are widespread, and can be formed via the sulfide pathway (Fe2+ + S2– = [FeS], [FeS] + H2S = FeS2 + H2; Rickard and Luther, 1997) with magmatic H2S abundant in the Dresser hydrothermal system throughout the multiple stages of hydrothermal events (Ueno et al., 2008 and references therein).
View in article
Wacey, D., Noffke, N., Cliff, J., Barley, M.E., Farquhar, J. (2015) Micro-scale quadruple sulfur isotope analysis of pyrite from the ∼3480 Ma Dresser Formation: New insights into sulfur cycling on the early Earth. Precambrian Research 258, 24–35.

The differences in these data sets are profound. Although recent in situ studies have confirmed separately the δ34S–Δ33S positively correlated Δ33S-negative (Baumgartner et al., 2020) and Δ33S-positive (Wacey et al., 2015) pyrites, the relationship between the two groups remains unknown.
View in article
Van Kranendonk, M.J., Philippot, P., Lepot, K., Bodorkos, S., Pirajno, F. (2008) Geological setting of Earth’s oldest fossils in the ca. 3.5 Ga Dresser Formation, Pilbara Craton, Western Australia. Precambrian Research 167, 93–124.

The chert-barite unit of Dresser Formation was generated by a complex hydrothermal system, thus the pyrites are likely to preserve multiple generations resulting from multiple stages of hydrothermal events (Van Kranendonk et al., 2008).
View in article
The chert-barite unit and the veins are generally considered to be formed in a volcanic caldera, with sedimentation accompanied by volcanism, hydrothermal circulation and precipitation, and tectonic activity (Van Kranendonk et al., 2008).
View in article
top
Supplementary Information
The Supplementary Information includes:
- 1. Geological Setting
- 2. Samples
- 3. Methods
- 4. Results
- 5. The Dependence of Multiple Sulfur Isotopic Compositions on Pyrite Texture and Petrography
- 6. The Dilution of S-MIF in Original Photochemical Products
- 7. Pyrites with Positive Δ33S and Negative δ34S
- Tables S-1 to S-6
- Figures S-1 to S-11
- Supplementary Information References
Download Tables S-4 to S-6 (Excel).
Download the Supplementary Information (PDF).
Figures
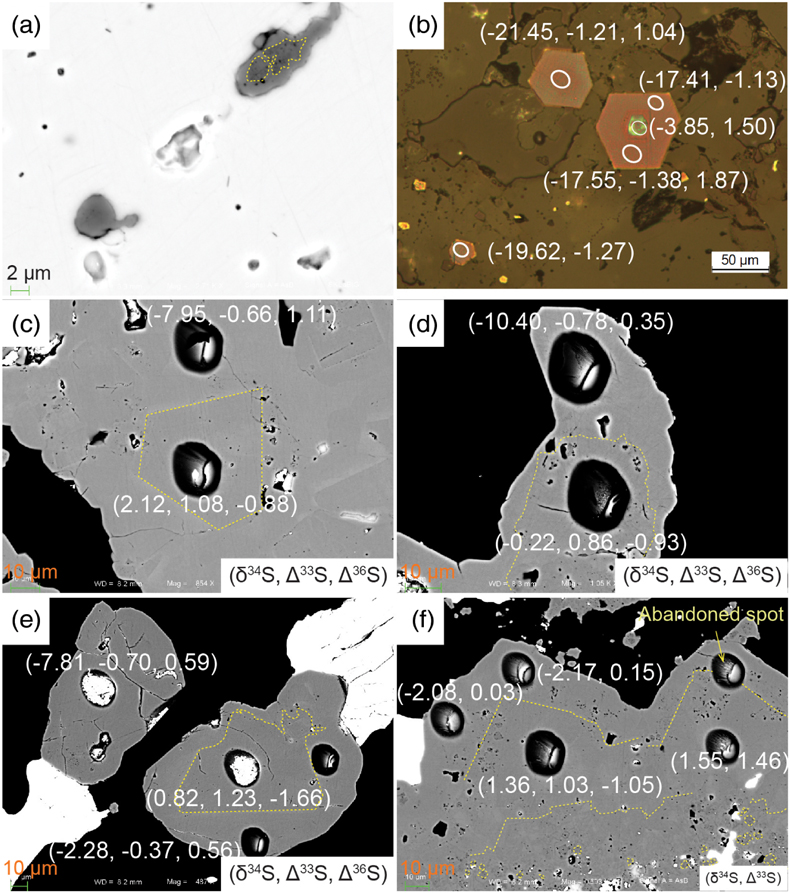
Figure 1 (a) BSE image of tiny pyrites aligned in an array within barite. (b) Photomicrograph (reflected light, NaOCl-etched pyrite) and (c–f) BSE images of core-rim textured pyrites. (a, c–f) are from PDP2c_97.17–97.20 m; (b) is from PDP2b_90.94–90.97 m.
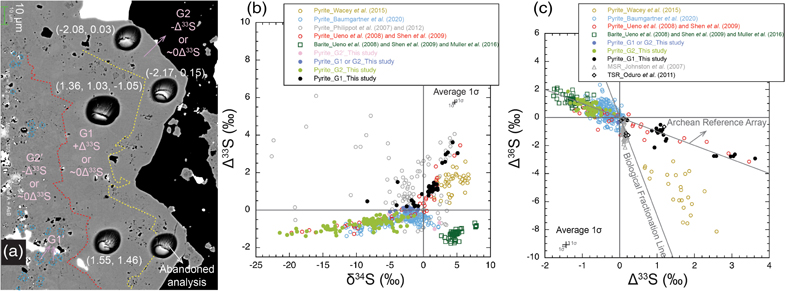
Figure 2 (b) δ34S-Δ33S and (c) Δ33S-Δ36S plots for G1, G2, G2′ pyrites, with (a) BSE image of a Py-ii showing four generations. Archaean Reference Array: Δ36S ≈ −Δ33S (Farquhar et al., 2001
Farquhar, J., Savarino, J., Airieau, S., Thiemens, M.H. (2001) Observation of wavelength-sensitive mass-independent sulfur isotope effects during SO2 photolysis: Implications for the early atmosphere. Journal of Geophysical Research 106, 32829–32839.
); Biological Fractionation Line: Δ36S ≈ −7Δ33S (Ono et al., 2006Ono, S., Wing, B., Johnston, D., Farquhar, J., Rumble, D. (2006) Mass-dependent fractionation of quadruple stable sulfur isotope system as a new tracer of sulfur biogeochemical cycles. Geochimica et Cosmochimica Acta 70, 2238–2252.
). 1σ = [(SE)2 + (SD)2]0.5, where SE is standard error of the analysis, and SD is standard deviation of Ruttan pyrite in the associated session.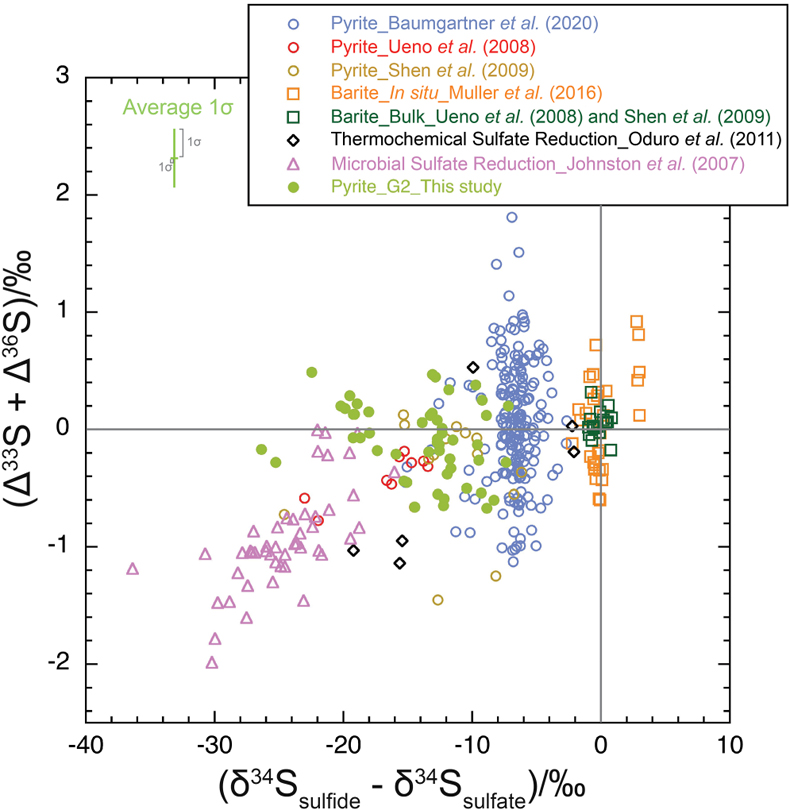
Figure 3 (δ34Ssulfide − δ34Ssulfate) – (Δ33S + Δ36S) plot for G2, along with the initial sulfates, MSR and TSR. A δ34Ssulfate value of 4.91 ‰ (the mean of Dresser barites) was used. Error bar 1σ of (δ34Ssulfide − δ34Ssulfate) is that of δ34Ssulfide (G2), and 1σ of (Δ33S + Δ36S) is (σΔ33S2 + σΔ36S2)0.5.