Medieval and recent SO2 budgets in the Reykjanes Peninsula: implication for future hazard
Affiliations | Corresponding Author | Cite as | Funding information- Share this article
-
Article views:780Cumulative count of HTML views and PDF downloads.
- Download Citation
- Rights & Permissions
top
Abstract

Figures and Tables
![]() Figure 1 Variations of S contents in groundmass glasses (filled circles) and PEP-corrected MIs (filled triangles) as a function of Mg# [Mg# = 100·Mg/(Mg+Fe2+), Fe2+/Fetot = 0.9] in samples from (a,b,d,e) the 800–1240 AD Fires, (c) the 2021–2023 Fagradalsfjall eruptions and (b) the 2023–2024 eruptions at Sundhnúksgígar. Data from the 2021 Fagradalsfjall eruption are from Halldórsson et al. (2022). Red and blue solid lines indicate fractional crystallisation paths calculated for geochemically enriched and depleted initial melt compositions, respectively (see Supplementary Information). The black dotted curve indicates SCSS along an empirical fractional crystallisation path calculated after Smythe et al. (2017), implemented in PySulfSat (Wieser and Gleeson, 2022). | ![]() Figure 2 (a) Variation of vent MS. (b) Magnitude of potential MS as a function of eruption volume for the 800–1240 AD Fires, the 2021–2023 Fagradalsfjall eruptions and the 2023 Sundhnúkar eruption. At a given volume, straight lines allow to calculate potential MS corresponding to maximum and minimum pre-eruptive S concentrations measured across the RP. Inset plot shows most common potential MS across the RP. (c) Daily SO2 emissions are calculated using MOR values and associated uncertainties from Óskarsson et al. (2024). Blue histogram indicates measured SO2 emissions during the 2021 Fagradalsfjall eruption (Esse et al., 2023). Data are coloured according to the volcanic system and only lavas with known volumes or MORs are included in the plots. | ![]() Figure 3 Simplified geological map of the RP and lava flows emplaced during the 800–1240 AD Fires. The map also illustrates the aerial extent of the 2021 (Pedersen et al., 2022), 2022 (Gunnarson et al., 2023) and 2023 (Belart et al., 2023) Fagradalsfjall lavas. Data from the 2023–2024 eruptions at Sundhnúksgígar are from the Landmælingar Íslands geoserver (gis.lmi.is/geoserver). When possible, lava flows are coloured according to calculated syneruptive SO2 emissions, ranging from 0.1 to 6 Mt. Orange shaded areas indicate urban areas. Numbers reflect the different erupted units as listed in Table 1. Seasonal wind roses reflect data at 900 mPa (∼1000 m a.s.l), which was the most common SO2 injection altitude during the 2021 Fagradalsfjall eruption (Esse et al., 2023). Spokes indicate the direction the wind is blowing from, and the length of each spoke shows the frequency. Wind data were extracted from ERA5 (Hersbach et al., 2023). | ![]() Table 1 Eruptive units studied in this work and summary of main results. CS MI, pre-eruptive S concentration; CS glass, post-eruptive S concentration; ΔCS, sulfur emissions at the vent per unit mass of melt, accounting for crystallinity; V, bulk lava volume; VDRE, vesicle-free lava volume; MS, syneruptive SO2 emissions at the vent; potential MS, potential SO2 emissions; MOR, mean output rates. Lava volumes for the medieval eruptions are from Einarsson et al. (1991), Jónsson (1978) and Sigurgeirsson (2004). ‘No.’ indicates the number of eruptive units, as indicated in Figure 3. |
Figure 1 | Figure 2 | Figure 3 | Table 1 |
top
Introduction
The release of volcanic gases and aerosols during volcanic eruptions can significantly impact the air quality and climate (e.g., Ilyinskaya et al., 2017
Ilyinskaya, E., Schmidt, A., Mather, T.A., Pope, F.D., Witham, C., Baxter, P., Jóhannsson, T., Pfeffer, M., Barsotti, S., Singh, A., Sanderson, P., Bergsson, B., McCormick Kilbride, B., Donovan, A., Peters, N., Oppenheimer, C., Edmonds, M. (2017) Understanding the environmental impacts of large fissure eruptions: Aerosol and gas emissions from the 2014–2015 Holuhraun eruption (Iceland). Earth and Planetary Science Letters 472, 309–322. https://doi.org/10.1016/j.epsl.2017.05.025
), as well as biodiversity (e.g., Weiser et al., 2022Weiser, F., Baumann, E., Jentsch, A., Medina, F.M., Lu, M., Nogales, M., Beierkuhnlein, C. (2022) Impact of Volcanic Sulfur Emissions on the Pine Forest of La Palma, Spain. Forests 13, 299. https://doi.org/10.3390/f13020299
). Among volcanic gases, sulfur species (SO2, H2S) and associated aerosols (SO4, H2SO4) are the most critical airborne hazards to human health, with short- and long-term impacts that have been recorded at variable distances from eruptive vents (e.g., Schmidt et al., 2015Schmidt, A., Leadbetter, S., Theys, N., Carboni, E., Witham, C.S., Stevenson, J.A., Birch, C.E., Thordarson, T., Turnock, S., Barsotti, S., Delaney, L., Feng, W., Grainger, R.G., Hort, M.C., Höskuldsson, Á., Ialongo, I., Ilyinskaya, E., Jóhannsson, T., Kenny, P., Mather, T.A., Richards, N.A.D., Shepherd, J. (2015) Satellite detection, long-range transport, and air quality impacts of volcanic sulfur dioxide from the 2014–2015 flood lava eruption at Bárðarbunga (Iceland). Journal of Geophysical Research: Atmospheres 120, 9739–9757. https://doi.org/10.1002/2015JD023638
; Ilyinskaya et al., 2017Ilyinskaya, E., Schmidt, A., Mather, T.A., Pope, F.D., Witham, C., Baxter, P., Jóhannsson, T., Pfeffer, M., Barsotti, S., Singh, A., Sanderson, P., Bergsson, B., McCormick Kilbride, B., Donovan, A., Peters, N., Oppenheimer, C., Edmonds, M. (2017) Understanding the environmental impacts of large fissure eruptions: Aerosol and gas emissions from the 2014–2015 Holuhraun eruption (Iceland). Earth and Planetary Science Letters 472, 309–322. https://doi.org/10.1016/j.epsl.2017.05.025
; Stewart et al., 2022Stewart, C., Damby, D.E., Horwell, C.J., Elias, T., Ilyinskaya, E., Tomašek, I., Longo, B.M., Schmidt, A., Carlsen, H.K., Mason, E., Baxter, P.J., Cronin, S., Witham, C. (2022) Volcanic air pollution and human health: recent advances and future directions. Bulletin of Volcanology 84, 11. https://doi.org/10.1007/s00445-021-01513-9
; Horwell et al., 2023Horwell, C.J., Baxter, P.J., Damby, D.E., Elias, T., Ilyinskaya, E., Sparks, R.S.J., Stewart, C., Tomašek, I. (2023) The International Volcanic Health Hazard Network (IVHHN): reflections on 20 years of progress. Frontiers in Earth Science 11, 1213363. https://doi.org/10.3389/feart.2023.1213363
). For example, several studies have associated cardiorespiratory issues with volcanic sulfur emissions (e.g., Carlsen et al., 2021Carlsen, H.K., Valdimarsdóttir, U., Briem, H., Dominici, F., Finnbjornsdottir, R.G., Jóhannsson, T., Aspelund, T., Gislason, T., Gudnason, T. (2021) Severe volcanic SO2 exposure and respiratory morbidity in the Icelandic population – a register study. Environmental Health 20, 23. https://doi.org/10.1186/s12940-021-00698-y
, and references therein). Hence, a detailed knowledge of potential sulfur releases of active volcanoes located in densely populated areas is critical to understand air quality hazards of future volcanic eruptions. This is the case of the Reykjanes Peninsula (RP) in southwest Iceland, an active spreading area segmented into five volcanic systems, which from west to east are Reykjanes, Svartsengi, Fagradalsfjall, Krýsuvík and Brennisteinsfjöll. The latest magmatic period in the RP occurred ∼800 years ago (Sæmundsson et al., 2020Sæmundsson, K., Sigurgeirsson, M.Á., Friðleifsson, G.Ó. (2020) Geology and structure of the Reykjanes volcanic system, Iceland. Journal of Volcanology and Geothermal Research 391, 106501. https://doi.org/10.1016/j.jvolgeores.2018.11.022
), but knowledge about sulfur outputs during those eruptions has been lacking thus far. Each volcanic system on the RP tends to activate during individual magmatic periods (Sæmundsson et al., 2020Sæmundsson, K., Sigurgeirsson, M.Á., Friðleifsson, G.Ó. (2020) Geology and structure of the Reykjanes volcanic system, Iceland. Journal of Volcanology and Geothermal Research 391, 106501. https://doi.org/10.1016/j.jvolgeores.2018.11.022
), and the recent 2021–2024 Fagradalsfjall and Svartsengi eruptions (Barsotti et al., 2023Barsotti, S., Parks, M.M., Pfeffer, M.A., Óladóttir, B.A., Barnie, T., Titos, M.M., Jónsdóttir, K., Pedersen, G.B.M., Hjartardóttir, Á.R., Stefansdóttir, G., Johannsson, T., Arason, Þ., Gudmundsson, M.T., Oddsson, B., Þrastarson, R.H., Ófeigsson, B.G., Vogfjörd, K., Geirsson, H., Hjörvar, T., von Löwis, S., Petersen, G.N., Sigurðsson, E.M. (2023) The eruption in Fagradalsfjall (2021, Iceland): how the operational monitoring and the volcanic hazard assessment contributed to its safe access. Natural Hazards 116, 3063–3092. https://doi.org/10.1007/s11069-022-05798-7
; Sigmundsson et al., 2024Sigmundsson, F., Parks, M., Geirsson, H., Hooper, A., Drouin, V., Vogfjörd, K.S., Ófeigsson, B.G., Greiner, S.H.M., Yang, Y., Lanzi, C., De Pascale, G.P., Jónsdóttir, K., Hreinsdóttir, S., Tolpekin, V., Friðriksdóttir, H.M., Einarsson, P., Barsotti, S. (2024) Fracturing and tectonic stress drives ultrarapid magma flow into dikes. Science 383, 1228–1235. https://doi.org/10.1126/science.adn2838
) suggest the potential initiation of a new eruptive period in an area that hosts ∼70 % of the Icelandic population. Consequently, there is an increased societal need for a deeper understanding of sulfur emissions across the RP, which is crucial for a comprehensive assessment of sulfur’s impact during future eruptions and its potential consequences for human health.Here, we focus on magmatic units erupted in the volcanic systems of Reykjanes, Svartsengi, Krýsuvík and Brennisteinsfjöll in the RP during the last medieval eruptive cycle, which occurred between the 8th century and 1240 AD, hereafter referred to as the 800–1240 AD Fires (Peate et al., 2009
Peate, D.W., Baker, J.A., Jakobsson, S.P., Waight, T.E., Kent, A.J.R., Grassineau, N.V., Skovgaard, A.C. (2009) Historic magmatism on the Reykjanes Peninsula, Iceland: a snap-shot of melt generation at a ridge segment. Contributions to Mineralogy and Petrology 157, 359–382. https://doi.org/10.1007/s00410-008-0339-4
; Caracciolo et al., 2023Caracciolo, A., Bali, E., Halldórsson, S.A., Guðfinnsson, G.H., Kahl, M., Þórðardóttir, I., Pálmadóttir, G.L., Silvestri, V. (2023) Magma plumbing architectures and timescales of magmatic processes during historical magmatism on the Reykjanes Peninsula, Iceland. Earth and Planetary Science Letters 621, 118378. https://doi.org/10.1016/j.epsl.2023.118378
). Additionally, we target the 2021–2023 Fagradalsfjall eruptions and the December 2023, January 2024 and February 2024 eruptions at Sundhnúksgígar in Svartsengi. We calculate syneruptive sulfur release and potential sulfur emissions of 19 geologically and petrochemically well characterised magmatic units (Peate et al., 2009Peate, D.W., Baker, J.A., Jakobsson, S.P., Waight, T.E., Kent, A.J.R., Grassineau, N.V., Skovgaard, A.C. (2009) Historic magmatism on the Reykjanes Peninsula, Iceland: a snap-shot of melt generation at a ridge segment. Contributions to Mineralogy and Petrology 157, 359–382. https://doi.org/10.1007/s00410-008-0339-4
; Caracciolo et al., 2023Caracciolo, A., Bali, E., Halldórsson, S.A., Guðfinnsson, G.H., Kahl, M., Þórðardóttir, I., Pálmadóttir, G.L., Silvestri, V. (2023) Magma plumbing architectures and timescales of magmatic processes during historical magmatism on the Reykjanes Peninsula, Iceland. Earth and Planetary Science Letters 621, 118378. https://doi.org/10.1016/j.epsl.2023.118378
) and compare those with sulfur emissions from the 2021 Fagradalsfjall eruption (Halldórsson et al., 2022Halldórsson, S.A., Marshall, E.W., Caracciolo, A., Matthews, S., Bali, E., Rasmussen, M.B., Ranta, E., Robin, J.G., Guðfinnsson, G.H., Sigmarsson, O., Maclennan, J., Jackson, M.G., Whitehouse, M.J., Jeon, H., van der Meer, Q.H.A., Mibei, G.K., Kalliokoski, M.H., Repczynska, M.M., Rúnarsdóttir, R.H., Sigurðsson, G., Pfeffer, M.A., Scott, S.W., Kjartansdóttir, R., Kleine, B.I., Oppenheimer, C., Aiuppa, A., Ilyinskaya, E., Bitetto, M., Giudice, G., Stefánsson, A. (2022) Rapid shifting of a deep magmatic source at Fagradalsfjall volcano, Iceland. Nature 609, 529–534. https://doi.org/10.1038/s41586-022-04981-x
; Barsotti et al., 2023Barsotti, S., Parks, M.M., Pfeffer, M.A., Óladóttir, B.A., Barnie, T., Titos, M.M., Jónsdóttir, K., Pedersen, G.B.M., Hjartardóttir, Á.R., Stefansdóttir, G., Johannsson, T., Arason, Þ., Gudmundsson, M.T., Oddsson, B., Þrastarson, R.H., Ófeigsson, B.G., Vogfjörd, K., Geirsson, H., Hjörvar, T., von Löwis, S., Petersen, G.N., Sigurðsson, E.M. (2023) The eruption in Fagradalsfjall (2021, Iceland): how the operational monitoring and the volcanic hazard assessment contributed to its safe access. Natural Hazards 116, 3063–3092. https://doi.org/10.1007/s11069-022-05798-7
). Also, we estimate daily SO2 emissions and develop an empirical approach to calculate worst- and best-case potential sulfur emissions for any eruption of a given volume emplaced in the RP.top
Samples and Methods
Scoria samples were collected from multiple vents within individual eruptive units of the 800–1240 AD Fires (Table S-1) (Caracciolo et al., 2023
Caracciolo, A., Bali, E., Halldórsson, S.A., Guðfinnsson, G.H., Kahl, M., Þórðardóttir, I., Pálmadóttir, G.L., Silvestri, V. (2023) Magma plumbing architectures and timescales of magmatic processes during historical magmatism on the Reykjanes Peninsula, Iceland. Earth and Planetary Science Letters 621, 118378. https://doi.org/10.1016/j.epsl.2023.118378
). Here, we present new sulfur (S) data for the same groundmass glass (n = 889) and melt inclusions (MIs) (n = 416) dataset published in Caracciolo et al. (2023)Caracciolo, A., Bali, E., Halldórsson, S.A., Guðfinnsson, G.H., Kahl, M., Þórðardóttir, I., Pálmadóttir, G.L., Silvestri, V. (2023) Magma plumbing architectures and timescales of magmatic processes during historical magmatism on the Reykjanes Peninsula, Iceland. Earth and Planetary Science Letters 621, 118378. https://doi.org/10.1016/j.epsl.2023.118378
. Additionally, we include new MI and groundmass glass data from quenched lavas and tephra erupted during the 2022 and 2023 Fagradalsfjall eruptions, as well as data from the eruptions at Sundhnúksgígar that occurred in the Svartsengi volcanic system in December 2023, January 2024 and February 2024. S was analysed by electron microprobe analyser (EMPA) at the University of Iceland, using the same analytical settings as in Caracciolo et al. (2020)Caracciolo, A., Bali, E., Guðfinnsson, G.H., Kahl, M., Halldórsson, S.A., Hartley, M.E., Gunnarsson, H. (2020) Temporal evolution of magma and crystal mush storage conditions in the Bárðarbunga-Veiðivötn volcanic system, Iceland. Lithos 352–353, 105234. https://doi.org/10.1016/j.lithos.2019.105234
, and MI compositions have been corrected for post-entrapment processes (PEP) (Tables S-2–S-4) (Caracciolo et al., 2023Caracciolo, A., Bali, E., Halldórsson, S.A., Guðfinnsson, G.H., Kahl, M., Þórðardóttir, I., Pálmadóttir, G.L., Silvestri, V. (2023) Magma plumbing architectures and timescales of magmatic processes during historical magmatism on the Reykjanes Peninsula, Iceland. Earth and Planetary Science Letters 621, 118378. https://doi.org/10.1016/j.epsl.2023.118378
).Here, we use the ‘petrological method’ (Devine et al., 1984
Devine, J.D., Sigurdsson, H., Davis, A.N., Self, S. (1984) Estimates of sulfur and chlorine yield to the atmosphere from volcanic eruptions and potential climatic effects. Journal of Geophysical Research: Solid Earth 89, 6309–6325. https://doi.org/10.1029/JB089iB07p06309
) to calculate eruptive sulfur emissions based on the difference between S concentrations in mineral-hosted MIs and S concentrations measured in groundmass glass (ΔCS). The idea behind this reconstruction method is that melt inclusions with similar composition to erupted melts preserve the pre-eruptive volatile content, and quenched groundmass glasses provide an estimate of the post-eruptive volatile content. For the different magmatic units, the highest S concentration measured in PEP-corrected MIs (CS MI) is selected as the pre-eruptive S concentration, whereas the lowest S concentration in groundmass glasses (CS glass) is chosen as the post-eruptive S concentration. By combining the mass of erupted magmas with the mass of S released, we can assess vent syneruptive SO2 emissions (MS) of individual eruptions (see Eqs. S-1, S-2) (e.g., Bali et al., 2018Bali, E., Hartley, M.E., Halldórsson, S.A., Gudfinnsson, G.H., Jakobsson, S. (2018) Melt inclusion constraints on volatile systematics and degassing history of the 2014–2015 Holuhraun eruption, Iceland. Contributions to Mineralogy and Petrology 173, 9. https://doi.org/10.1007/s00410-017-1434-1
, and references therein). Furthermore, we calculate the magnitude of potential SO2 emissions (potential MS), which refers to complete degassing of all pre-eruptive sulfur (CS glass = 0) and reflects the maximum amount of SO2 that a specific eruption could potentially have released, assuming that there is no degassing of unerupted magma. This reconstruction method has been shown to have matched field-based volatile measurements exceptionally well during the 2014–2015 Holuhraun eruption (Bali et al., 2018Bali, E., Hartley, M.E., Halldórsson, S.A., Gudfinnsson, G.H., Jakobsson, S. (2018) Melt inclusion constraints on volatile systematics and degassing history of the 2014–2015 Holuhraun eruption, Iceland. Contributions to Mineralogy and Petrology 173, 9. https://doi.org/10.1007/s00410-017-1434-1
; Pfeffer et al., 2018Pfeffer, M.A., Bergsson, B., Barsotti, S., Stefánsdóttir, G., Galle, B., Arellano, S., Conde, V., Donovan, A., Ilyinskaya, E., Burton, M., Aiuppa, A., Whitty, R.C.W., Simmons, I.C., Arason, Þ., Jónasdóttir, E.B., Keller, N.S., Yeo, R.F., Arngrímsson, H., Jóhannsson, Þ., Butwin, M.K., Askew, R.A., Dumont, S., Von Löwis, S., Ingvarsson, Þ., La Spina, A., Thomas, H., Prata, F., Grassa, F., Giudice, G., Stefánsson, A., Marzano, F., Montopoli, M., Mereu, L. (2018) Ground-Based Measurements of the 2014–2015 Holuhraun Volcanic Cloud (Iceland). Geosciences 8, 29. https://doi.org/10.3390/geosciences8010029
) and the 2021 Fagradalsfjall eruption (this work, Table 1).Table 1 Eruptive units studied in this work and summary of main results. CS MI, pre-eruptive S concentration; CS glass, post-eruptive S concentration; ΔCS, sulfur emissions at the vent per unit mass of melt, accounting for crystallinity; V, bulk lava volume; VDRE, vesicle-free lava volume; MS, syneruptive SO2 emissions at the vent; potential MS, potential SO2 emissions; MOR, mean output rates. Lava volumes for the medieval eruptions are from Einarsson et al. (1991)
Einarsson, S., Johannesson, H., Sveinbjörnsdóttir, Á.E. (1991) Krísuvíkureldar II. Kapelluhraun og gátan um aldur Hellnahrauns. Jökull 41, 61–80. https://doi.org/10.33799/jokull1991.41.061
, Jónsson (1978)Jónsson, J. (1978) Jarðfræðikort af Reykjanesskaga : 1. Skýringar við jarðfræðikort ; 2. Jarðfræðikort. Orkustofnun jarðhitadeild, Reykjavík. http://hdl.handle.net/10802/4981
and Sigurgeirsson (2004)Sigurgeirsson, M.Á. (2004) Þáttur úr gossögu Reykjaness. Náttúrufræðingurinn 72, 21–28. https://timarit.is/gegnir/991000816469706886
. ‘No.’ indicates the number of eruptive units, as indicated in Figure 3.Eruptive unit | Volcanic system | Age | V | Mass | MS | MORb | Time of lava | Daily SO2 emissions | |||||||
A.D. | ppm | ppm | ppm | km3 | km3 | Kg | Mt | Mt | m3/s | days | t/day | ||||
1 | SO | Reykjanes | 1210–1240 | 1559 | 258 | 1275 | 0.10 | 0.09 | 2.3E+11 | 0.58 | 0.71 | 6.4–67.9 (17.9) | 17–193 (65) | 3570–40,450 (10,620) | |
2 | Arnarseturshraun | SÖ-A | Svartsengi | 1210–1240 | 1907 | 196 | 1667 | 0.55 | 0.47 | 1.3E+12 | 4.23 | 4.81 | 26.1–60.1 (33.5) | 106–244 (190) | 20,420–47,000 (26,200) |
3 | Eldvarpahraun | SÖ-E | Svartsengi | 1210–1240 | 1907 | 112 | 1759 | 0.28 | 0.24 | 6.4E+11 | 2.26 | 2.45 | 26–93.7 (33.3) | 35–125 (97) | 21,340–76,900 (27,300) |
4 | Illahraun | SÖ-I | Svartsengi | 1210–1240 | 1907 | 312 | 1563 | 0.05 | 0.04 | 1.1E+11 | 0.36 | 0.44 | 4–42.7 (11.2) | 14–145 (52) | 2920–31,140 (8180) |
5 | Sundhnúkar Dec. 2023 | Sund 2023 | Svartsengi | 2023 | 1610 | 210 | 1372 | 0.011d | 0.01 | 2.5E+10 | 0.07 | 0.08 | 50d | 2.5 | 32,000 |
6 | Sundhnúkar Jan. 2024* | Sund 2024a | Svartsengi | 2024 | 1400 | 160 | 1215 | - | - | - | - | - | - | <2 | - |
7 | Sundhnúkar Feb. 2024* | Sund 2024b | Svartsengi | 2024 | 1607 | 164 | 1414 | - | - | - | - | - | - | <2 | - |
8 | Fagradalsfjall 2021 | Fagra 2021 | Fagradalsfjall | 2021 | 1170 | 20 | 1127 | 0.15c | 0.13 | 4.1E+11 | 0.78 | 0.80 | 9.5c | 185 | 5000 |
9 | Fagradalsfjall 2022 | Fagra 2022 | Fagradalsfjall | 2022 | 1300 | 120 | 1180 | 0.011d | 0.01 | 2.5E+10 | 0.06 | 0.07 | 7.0d | 18 | 3780 |
10 | Fagradalsfjall 2023 | Fagra 2023 | Fagradalsfjall | 2023 | 1170 | 120 | 1050 | 0.015d | 0.013 | 3.4E+10 | 0.07 | 0.08 | 7.0d | 26 | 3360 |
11 | Ögmundarhraun | ÖGM | Krýsuvík | 1151–1188 | 1517 | 138 | 1351 | 0.13 | 0.11 | 3.0E+11 | 0.81 | 0.90 | 31.9–73.3 (40.8) | 21–47 (37) | 20,110–46,220 (25,750) |
12 | Kapelluhraun | KAP | Krýsuvík | 1151–1188 | 1482 | 183 | 1273 | 0.07 | 0.06 | 1.6E+11 | 0.41 | 0.48 | 20.2–213 (56) | 4–40 (14) | 12,000–126,500 (33,240) |
13 | Mávahlíðarhraun | MÁH | Krýsuvík | 1151–1188 | 1297 | 280 | 997 | 0.02 | 0.02 | 4.6E+10 | 0.09 | 0.12 | 5.8–61 (16) | 4–40 (14) | 2700–28,370 (7450) |
14 | Hrútafellshrauna | HRF | Krýsuvík | 8th–9th century | 1546 | 252 | 1268 | 0.04a | 0.03 | 9.0E+10 | 0.23 | 0.28 | 11.4–120 (31.5) | 4–40 (14) | 6750–71,000 (18,660) |
15 | Hvammahraun | h128 | Brennisteinsfjöll | 8th–9th century | 1900 | 90 | 1810 | 0.72 | 0.61 | 1.7E+12 | 5.86 | 6.27 | 48.3–510.6 (134.1) | 16–173 (62) | 39,970–422,560 (111,000) |
16 | Kistuhraun | h130 | Brennisteinsfjöll | 900–1100 | 1494 | 94 | 1372 | 0.08 | 0.07 | 1.8E+11 | 0.50 | 0.55 | 6.1–22 (7.8) | 42–152 (119) | 3900–14,000 (5000) |
17 | Selvogshraun | h138 | Brennisteinsfjöll | 10th–11th century | 1504 | 126 | 1350 | 0.19 | 0.16 | 4.4E+11 | 1.18 | 1.31 | 14.2–149.9 (39.4) | 15–155 (56) | 8950–94,450 (24,810) |
18 | Tvíbollahraun | tv | Brennisteinsfjöll | 950 | 1367 | 129 | 1213 | 0.37 | 0.31 | 8.5E+11 | 2.06 | 2.32 | 18.7–43 (24) | 100–229 (178) | 10,580–24,340 (13,580) |
19 | Svartihryggura | h142 | Brennisteinsfjöll | 900–1200 | 1341 | 147 | 1170 | 0.0005a | 0.0004 | 1.3E+09 | 0.003 | 0.003 | 0.5–3.3 (2) | 2–13 (3) | 270–1800 (1100) |
20 | Húsfellsbrunia | Hú1 & Hú2 | Brennisteinsfjöll | 9th–13th century | 1739 | 55 | 1650 | 0.20a | 0.17 | 4.9E+11 | 1.62 | 1.70 | 50.6–116.4 (64.9) | 21–49 (38) | 38,960–89,620 (50,000) |
21 | Kristnitökuhrauna | KRT | Brennisteinsfjöll | 1000 | 1640 | 118 | 1492 | 0.06a | 0.05 | 1.4E+11 | 0.41 | 0.45 | 14.1–50.9 (18) | 14–50 (39) | 9800–35,420 (12,570) |
aLava volume estimated by assuming a thickness of 5 m, consistent with average thicknesses of lava flows of known volumes with a similar aerial extent.
bMOR values (within brackets) and uncertainty ranges for the medieval eruptions are from Óskarsson et al. (2024)Óskarsson, B.V., Askew, R.A., Guðmundsson, H. (2024) Assessing the mean output rate (MOR) of past effusive basaltic eruptions - a look at the postglacial volcanism of the Reykjanes Peninsula in Iceland. EarthArXiv Preprint v1. https://doi.org/10.31223/X5CH68.
cV and MOR from Pedersen et al. (2022)Pedersen, G.B.M., Belart, J.M.C., Óskarsson, B.V., Gudmundsson, M.T., Gies, N., Högnadóttir, T., Hjartardóttir, Á.R., Pinel, V., Berthier, E., Dürig, T., Reynolds, H.I., Hamilton, C.W., Valsson, G., Einarsson, P., Ben-Yehosua, D., Gunnarsson, A., Oddsson, B. (2022) Volume, Effusion Rate, and Lava Transport During the 2021 Fagradalsfjall Eruption: Results From Near Real-Time Photogrammetric Monitoring. Geophysical Research Letters 49, e2021GL097125. https://doi.org/10.1029/2021GL097125.
dV and MOR from Pedersen et al. (2024)Pedersen, G.B.M., Belart, J.M.C., Óskarsson, B.V., Gunnarson, S.R., Gudmundsson, M.T., Reynolds, H.I., Valsson, G., Högnadóttir, T., Pinel, V., Parks, M.M., Drouin, V., Askew, R.A., Dürig, T., Þrastarson, R.H. (2024) Volume, effusion rates and lava hazards of the 2021, 2022 and 2023 Reykjanes fires: Lessons learned from near real-time photogrammetric monitoring. EGU General Assembly 2024, Vienna, Austria, 14–19 April 2024, Abstract EGU24-10724. https://doi.org/10.5194/egusphere-egu24-10724.
*Lava volumes for the 2024 eruptions at Sundhnúksgígar are not available at the current stage.
top
Sulfur Concentrations in MIs and Groundmass Glass
Sulfur concentration in MIs is in the range of 200–1900 ppm, with a relatively large variability of S at a given MI Mg#. Particularly, the most primitive MIs (Mg# > 65), exclusively preserved in Reykjanes and Krýsuvík, record S contents in the range of 580–1070 ppm (Fig. 1). S concentration in PEP-corrected MI compositions increases with decreasing MI Mg#, as expected for melt compositions controlled by fractional crystallisation. MIs from the 2023–2024 eruptions at Sundhnúksgígar record pre-eruptive S concentrations in the range of 1400–1600 ppm, in agreement with MI data from the medieval eruptions (Fig. 1b). MIs from the 2022–2023 Fagradalsfjall eruptions closely match S concentrations measured in the 2021 products (Fig. 1c). Groundmass glasses from Brennisteinsfjöll have mean S contents in the range of 150–280 ppm, lower than mean S contents measured in glasses from the other volcanic systems (280–450 ppm) (Fig. 1, Table 1). For comparison, MIs from the 2021 Fagradalsfjall eruption contain maximum S concentrations of 1200 ppm, whereas the groundmass glasses contain 20–200 ppm S. Sulfide globules were not observed in the erupted samples.
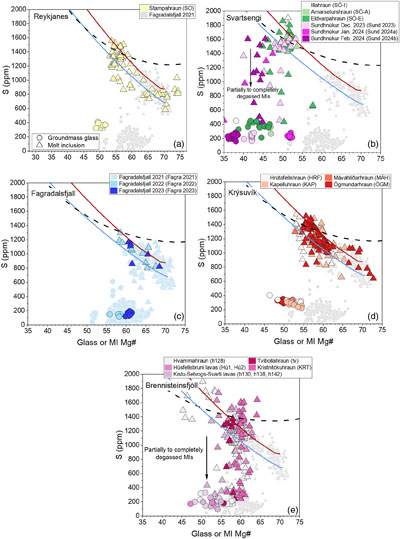
Figure 1 Variations of S contents in groundmass glasses (filled circles) and PEP-corrected MIs (filled triangles) as a function of Mg# [Mg# = 100·Mg/(Mg+Fe2+), Fe2+/Fetot = 0.9] in samples from (a,b,d,e) the 800–1240 AD Fires, (c) the 2021–2023 Fagradalsfjall eruptions and (b) the 2023–2024 eruptions at Sundhnúksgígar. Data from the 2021 Fagradalsfjall eruption are from Halldórsson et al. (2022)
Halldórsson, S.A., Marshall, E.W., Caracciolo, A., Matthews, S., Bali, E., Rasmussen, M.B., Ranta, E., Robin, J.G., Guðfinnsson, G.H., Sigmarsson, O., Maclennan, J., Jackson, M.G., Whitehouse, M.J., Jeon, H., van der Meer, Q.H.A., Mibei, G.K., Kalliokoski, M.H., Repczynska, M.M., Rúnarsdóttir, R.H., Sigurðsson, G., Pfeffer, M.A., Scott, S.W., Kjartansdóttir, R., Kleine, B.I., Oppenheimer, C., Aiuppa, A., Ilyinskaya, E., Bitetto, M., Giudice, G., Stefánsson, A. (2022) Rapid shifting of a deep magmatic source at Fagradalsfjall volcano, Iceland. Nature 609, 529–534. https://doi.org/10.1038/s41586-022-04981-x
. Red and blue solid lines indicate fractional crystallisation paths calculated for geochemically enriched and depleted initial melt compositions, respectively (see Supplementary Information). The black dotted curve indicates SCSS along an empirical fractional crystallisation path calculated after Smythe et al. (2017)Smythe, D.J., Wood, B.J., Kiseeva, E.S. (2017) The S content of silicate melts at sulfide saturation: New experiments and a model incorporating the effects of sulfide composition. American Mineralogist 102, 795–803. https://doi.org/10.2138/am-2017-5800CCBY
, implemented in PySulfSat (Wieser and Gleeson, 2022Wieser, P.E., Gleeson, M. (2022) PySulfSat: An open-source Python3 tool for modeling sulfide and sulfate saturation. Volcanica 6, 107–127. https://doi.org/10.30909/vol.06.01.107127
).top
Assessing Sulfur Variability and Degassing during the 800–1240 AD Fires
Considering that medieval and recent eruptions on the RP are likely sourced from mantle-derived melts of diverse compositions (Peate et al., 2009
Peate, D.W., Baker, J.A., Jakobsson, S.P., Waight, T.E., Kent, A.J.R., Grassineau, N.V., Skovgaard, A.C. (2009) Historic magmatism on the Reykjanes Peninsula, Iceland: a snap-shot of melt generation at a ridge segment. Contributions to Mineralogy and Petrology 157, 359–382. https://doi.org/10.1007/s00410-008-0339-4
; Halldórsson et al., 2022Halldórsson, S.A., Marshall, E.W., Caracciolo, A., Matthews, S., Bali, E., Rasmussen, M.B., Ranta, E., Robin, J.G., Guðfinnsson, G.H., Sigmarsson, O., Maclennan, J., Jackson, M.G., Whitehouse, M.J., Jeon, H., van der Meer, Q.H.A., Mibei, G.K., Kalliokoski, M.H., Repczynska, M.M., Rúnarsdóttir, R.H., Sigurðsson, G., Pfeffer, M.A., Scott, S.W., Kjartansdóttir, R., Kleine, B.I., Oppenheimer, C., Aiuppa, A., Ilyinskaya, E., Bitetto, M., Giudice, G., Stefánsson, A. (2022) Rapid shifting of a deep magmatic source at Fagradalsfjall volcano, Iceland. Nature 609, 529–534. https://doi.org/10.1038/s41586-022-04981-x
; Harðardóttir et al., 2022Harðardóttir, S., Matthews, S., Halldórsson, S.A., Jackson, M.G. (2022) Spatial distribution and geochemical characterization of Icelandic mantle end-members: Implications for plume geometry and melting processes. Chemical Geology 604, 120930. https://doi.org/10.1016/j.chemgeo.2022.120930
; Caracciolo et al., 2023Caracciolo, A., Bali, E., Halldórsson, S.A., Guðfinnsson, G.H., Kahl, M., Þórðardóttir, I., Pálmadóttir, G.L., Silvestri, V. (2023) Magma plumbing architectures and timescales of magmatic processes during historical magmatism on the Reykjanes Peninsula, Iceland. Earth and Planetary Science Letters 621, 118378. https://doi.org/10.1016/j.epsl.2023.118378
), including melts with variable S contents (Ranta et al., 2022Ranta, E., Gunnarsson-Robin, J., Halldórsson, S.A., Ono, S., Izon, G., Jackson, M.G., Reekie, C.D.J., Jenner, F.E., Guðfinnsson, G.H., Jónsson, Ó.P., Stefánsson, A. (2022) Ancient and recycled sulfur sampled by the Iceland mantle plume. Earth and Planetary Science Letters 584, 117452. https://doi.org/10.1016/j.epsl.2022.117452
), we use our MI record to estimate S contents of the local enriched and depleted end member melt components. We distinguish between these components from the K2O/TiO2 variability, a robust tracer of mantle heterogeneities in Iceland (Halldórsson et al., 2022Halldórsson, S.A., Marshall, E.W., Caracciolo, A., Matthews, S., Bali, E., Rasmussen, M.B., Ranta, E., Robin, J.G., Guðfinnsson, G.H., Sigmarsson, O., Maclennan, J., Jackson, M.G., Whitehouse, M.J., Jeon, H., van der Meer, Q.H.A., Mibei, G.K., Kalliokoski, M.H., Repczynska, M.M., Rúnarsdóttir, R.H., Sigurðsson, G., Pfeffer, M.A., Scott, S.W., Kjartansdóttir, R., Kleine, B.I., Oppenheimer, C., Aiuppa, A., Ilyinskaya, E., Bitetto, M., Giudice, G., Stefánsson, A. (2022) Rapid shifting of a deep magmatic source at Fagradalsfjall volcano, Iceland. Nature 609, 529–534. https://doi.org/10.1038/s41586-022-04981-x
; Harðardóttir et al., 2022Harðardóttir, S., Matthews, S., Halldórsson, S.A., Jackson, M.G. (2022) Spatial distribution and geochemical characterization of Icelandic mantle end-members: Implications for plume geometry and melting processes. Chemical Geology 604, 120930. https://doi.org/10.1016/j.chemgeo.2022.120930
) (see Supplementary Information). Our modelling, considering that S behaves as an incompatible element in basaltic magmas, shows that most of the MI S variability can be explained by fractional crystallisation (FC) and mixing of, at least, two end member melt compositions (Fig. 1a–d).In order to evaluate S saturation during magma ascent and fractional crystallisation through the crust, we calculate sulfur content at sulfide saturation (SCSS) along a FC path, which reflects the amount of S2− present in a melt in equilibrium with a sulfide phase (Smythe et al., 2017
Smythe, D.J., Wood, B.J., Kiseeva, E.S. (2017) The S content of silicate melts at sulfide saturation: New experiments and a model incorporating the effects of sulfide composition. American Mineralogist 102, 795–803. https://doi.org/10.2138/am-2017-5800CCBY
) (see Supplementary Information). Our modelling suggests that melts are sulfide undersaturated during most of magmatic fractionation across the RP (Figs. 1, S-3, S-4). Only magmas from Svartsengi and Brennisteinsfjöll have a high likelihood to be sulfide saturated prior to eruptions. Furthermore, sulfide saturation is reached earlier during magmatic differentiation of enriched mantle-derived melts than depleted melts (Fig. 1).Modelling of S degassing with Sulfur_X (Ding et al., 2023
Ding, S., Plank, T., Wallace, P.J., Rasmussen, D.J. (2023) Sulfur_X: A Model of Sulfur Degassing During Magma Ascent. Geochemistry, Geophysics, Geosystems 24, e2022GC010552. https://doi.org/10.1029/2022GC010552
) suggests that the basaltic melts that erupted during the 800–1240 AD Reykjanes Fires are unlikely to degas significant amounts of S at known pre-eruptive magma storage depths (Caracciolo et al., 2023Caracciolo, A., Bali, E., Halldórsson, S.A., Guðfinnsson, G.H., Kahl, M., Þórðardóttir, I., Pálmadóttir, G.L., Silvestri, V. (2023) Magma plumbing architectures and timescales of magmatic processes during historical magmatism on the Reykjanes Peninsula, Iceland. Earth and Planetary Science Letters 621, 118378. https://doi.org/10.1016/j.epsl.2023.118378
) and that significant S degassing only takes place during magma ascent in the last 0.2 kbar (<700 m) (Fig. S-1).top
Sulfur Emissions across the RP
Sulfur release ranges between 1000 and 1770 ppm across the RP, a typical range for Icelandic rift basalts (Ranta et al., 2024
Ranta, E., Halldórsson, S.A., Óladóttir, B.A., Pfeffer, M.A., Caracciolo, A., Bali, E., Guðfinnsson, G.H., Kahl, M., Barsotti, S. (2024) Magmatic Controls on Volcanic Sulfur Emissions at the Iceland Hotspot. EarthArXiv Preprint v1. https://doi.org/10.31223/X51102
), with the largest ΔCS found in lavas from Svartsengi and Brennisteinsfjöll (Table 1). ΔCS values can be scaled by the mass of erupted material to estimate MS of individual eruptions, using published volumes of individual eruptive units, in the range of 0.01 km3 to 0.72 km3 (Table 1). Using a melt density of 2700 kg/m3 and assuming a bulk vesicularity of 15 vol. %, we calculate MS between 0.003 and 5.9 Mt (Fig. 2a). The most voluminous lavas found in Svartsengi and Brennisteinsfjöll released the highest mass of SO2 into the atmosphere during the medieval period. The syneruptive SO2 released by these latter voluminous lavas is approximately 2 to 6 times larger than syneruptive SO2 emissions during the 2021 Fagradalsfjall eruption, for which we estimated MS = 0.78 Mt (MS measured = 0.97 ± 0.5; Barsotti et al., 2023Barsotti, S., Parks, M.M., Pfeffer, M.A., Óladóttir, B.A., Barnie, T., Titos, M.M., Jónsdóttir, K., Pedersen, G.B.M., Hjartardóttir, Á.R., Stefansdóttir, G., Johannsson, T., Arason, Þ., Gudmundsson, M.T., Oddsson, B., Þrastarson, R.H., Ófeigsson, B.G., Vogfjörd, K., Geirsson, H., Hjörvar, T., von Löwis, S., Petersen, G.N., Sigurðsson, E.M. (2023) The eruption in Fagradalsfjall (2021, Iceland): how the operational monitoring and the volcanic hazard assessment contributed to its safe access. Natural Hazards 116, 3063–3092. https://doi.org/10.1007/s11069-022-05798-7
). These are roughly between 20 and 70 % of the syneruptive SO2 emissions estimated for the 2014–2015 Holuhraun eruption (MS = 10.5 Mt; Bali et al., 2018Bali, E., Hartley, M.E., Halldórsson, S.A., Gudfinnsson, G.H., Jakobsson, S. (2018) Melt inclusion constraints on volatile systematics and degassing history of the 2014–2015 Holuhraun eruption, Iceland. Contributions to Mineralogy and Petrology 173, 9. https://doi.org/10.1007/s00410-017-1434-1
). We calculate SO2 release of 0.06–0.07 Mt for the 2022 and 2023 Fagradalsfjall eruptions, respectively. However, for a given mass of melt, the 2021–2023 Fagradalsfjall eruptions released a comparable mass of SO2 (Table 1). Conversely, the 2023–2024 eruptions at Sundhnúksgígar slightly exceeded SO2 emissions during the 2021–2023 Fagradalsfjall eruptions (Table 1). Similarly, we have calculated potential MS, the maximum mass of SO2 that could potentially have been released during each eruption. Potential MS across the RP ranges between 0.003 and 6.3 Mt and is only slightly higher than vent MS, as most of the S is released into the atmosphere during eruptions rather than staying dissolved in the lava (Table 1).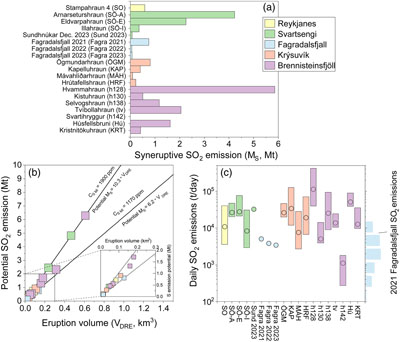
Figure 2 (a) Variation of vent MS. (b) Magnitude of potential MS as a function of eruption volume for the 800–1240 AD Fires, the 2021–2023 Fagradalsfjall eruptions and the 2023 Sundhnúkar eruption. At a given volume, straight lines allow to calculate potential MS corresponding to maximum and minimum pre-eruptive S concentrations measured across the RP. Inset plot shows most common potential MS across the RP. (c) Daily SO2 emissions are calculated using MOR values and associated uncertainties from Óskarsson et al. (2024)
Óskarsson, B.V., Askew, R.A., Guðmundsson, H. (2024) Assessing the mean output rate (MOR) of past effusive basaltic eruptions - a look at the postglacial volcanism of the Reykjanes Peninsula in Iceland. EarthArXiv Preprint v1. https://doi.org/10.31223/X5CH68
. Blue histogram indicates measured SO2 emissions during the 2021 Fagradalsfjall eruption (Esse et al., 2023Esse, B., Burton, M., Hayer, C., Pfeffer, M.A., Barsotti, S., Theys, N., Barnie, T., Titos, M. (2023) Satellite derived SO2 emissions from the relatively low-intensity, effusive 2021 eruption of Fagradalsfjall, Iceland. Earth and Planetary Science Letters 619, 118325. https://doi.org/10.1016/j.epsl.2023.118325
). Data are coloured according to the volcanic system and only lavas with known volumes or MORs are included in the plots.top
Evaluating End Member Scenarios of SO2 Emissions and Hazard Potential for Future Eruptions across the RP
Based on the MI record of the 2021–2023 Fagradalsfjall eruptions (Halldórsson et al., 2022
Halldórsson, S.A., Marshall, E.W., Caracciolo, A., Matthews, S., Bali, E., Rasmussen, M.B., Ranta, E., Robin, J.G., Guðfinnsson, G.H., Sigmarsson, O., Maclennan, J., Jackson, M.G., Whitehouse, M.J., Jeon, H., van der Meer, Q.H.A., Mibei, G.K., Kalliokoski, M.H., Repczynska, M.M., Rúnarsdóttir, R.H., Sigurðsson, G., Pfeffer, M.A., Scott, S.W., Kjartansdóttir, R., Kleine, B.I., Oppenheimer, C., Aiuppa, A., Ilyinskaya, E., Bitetto, M., Giudice, G., Stefánsson, A. (2022) Rapid shifting of a deep magmatic source at Fagradalsfjall volcano, Iceland. Nature 609, 529–534. https://doi.org/10.1038/s41586-022-04981-x
; this work), the 2023–2024 eruptions at Sundhnúksgígar and the 800–1240 AD Fires (this work), we constrain potential maximum (1900 ppm) and minimum (1170 ppm) pre-eruptive S concentrations and use these to estimate potential MS of future eruptions in the RP. With these constraints, we developed an empirical approach to assess potential MS for a given eruption of known lava volume, with important applications for forecasting the worst- and best-case scenarios of potential MS of future eruptive events (Fig. 2b). For example, based on our approach, an eruption with an eruptive volume of 0.4 km3 could release between 2.9 Mt and 4.1 Mt SO2. This method also has an application when it comes to evaluating the long-term SO2 impact of ongoing eruptions in the RP. If the mean magma output rate (MOR) is known and fixed, one can roughly estimate the volume of the lava flow and calculate potential MS at any given moment from the onset of the eruption. This provides a valuable tool to assess best- and worst-case scenarios for SO2 pollution during ongoing events.Eruptive MS calculations are strongly dependent on lava flow volumes. Hence, when it comes to comparing the 800–1240 AD Fires with the 2021–2024 eruptions, a more relevant parameter is the mean daily SO2 emissions, which also is an important parameter from a hazard perspective. We have estimated daily SO2 emissions for the 800–1240 AD Fires using MOR values calculated by Oskarsson et al. (2024)
Óskarsson, B.V., Askew, R.A., Guðmundsson, H. (2024) Assessing the mean output rate (MOR) of past effusive basaltic eruptions - a look at the postglacial volcanism of the Reykjanes Peninsula in Iceland. EarthArXiv Preprint v1. https://doi.org/10.31223/X5CH68
, in the range of 3–119 m3/s (Table 1, Eq. S-3). Mean daily SO2 emissions during the medieval eruptions likely ranged between 1000 t/day and 111,000 t/day (Fig. 2c). In comparison, during the 2021, 2022 and 2023 Fagradalsfjall eruptions, we calculate average daily SO2 emissions of 5000, 3780 and 3360 t/day, respectively. The estimate for the 2021 Fagradalsfjall eruption is in agreement with the majority of measured daily SO2 emissions throughout the 2021 Fagradalsfjall eruption, in the range of 1000–7600 t/day (Esse et al., 2023Esse, B., Burton, M., Hayer, C., Pfeffer, M.A., Barsotti, S., Theys, N., Barnie, T., Titos, M. (2023) Satellite derived SO2 emissions from the relatively low-intensity, effusive 2021 eruption of Fagradalsfjall, Iceland. Earth and Planetary Science Letters 619, 118325. https://doi.org/10.1016/j.epsl.2023.118325
), and with daily SO2 emissions of 5240 ± 2700 t/day, calculated assuming 0.97 ± 0.5 Mt total mass of SO2 (Barsotti et al., 2023Barsotti, S., Parks, M.M., Pfeffer, M.A., Óladóttir, B.A., Barnie, T., Titos, M.M., Jónsdóttir, K., Pedersen, G.B.M., Hjartardóttir, Á.R., Stefansdóttir, G., Johannsson, T., Arason, Þ., Gudmundsson, M.T., Oddsson, B., Þrastarson, R.H., Ófeigsson, B.G., Vogfjörd, K., Geirsson, H., Hjörvar, T., von Löwis, S., Petersen, G.N., Sigurðsson, E.M. (2023) The eruption in Fagradalsfjall (2021, Iceland): how the operational monitoring and the volcanic hazard assessment contributed to its safe access. Natural Hazards 116, 3063–3092. https://doi.org/10.1007/s11069-022-05798-7
). In contrast, the December 2023 Sundhnúkar eruption released 32,000 t/day SO2 (Table 1). Our calculations highlight that future eruptions in the RP may have the potential to release significantly more SO2 on a daily basis than the 2021–2024 eruptions.SO2 emissions during the 800–1240 AD Fires and the 2021–2024 eruptions are small compared to those during the 2014–2015 Holuhraun basaltic eruption (9.2 Mt SO2; Pfeffer et al., 2018
Pfeffer, M.A., Bergsson, B., Barsotti, S., Stefánsdóttir, G., Galle, B., Arellano, S., Conde, V., Donovan, A., Ilyinskaya, E., Burton, M., Aiuppa, A., Whitty, R.C.W., Simmons, I.C., Arason, Þ., Jónasdóttir, E.B., Keller, N.S., Yeo, R.F., Arngrímsson, H., Jóhannsson, Þ., Butwin, M.K., Askew, R.A., Dumont, S., Von Löwis, S., Ingvarsson, Þ., La Spina, A., Thomas, H., Prata, F., Grassa, F., Giudice, G., Stefánsson, A., Marzano, F., Montopoli, M., Mereu, L. (2018) Ground-Based Measurements of the 2014–2015 Holuhraun Volcanic Cloud (Iceland). Geosciences 8, 29. https://doi.org/10.3390/geosciences8010029
). However, volcanic eruptions in the RP are potentially considered to be more hazardous due to their proximity to inhabited areas, to the international airport and to the large number of visitors expected at eruption sites (Fig. 3) (Barsotti et al., 2023Barsotti, S., Parks, M.M., Pfeffer, M.A., Óladóttir, B.A., Barnie, T., Titos, M.M., Jónsdóttir, K., Pedersen, G.B.M., Hjartardóttir, Á.R., Stefansdóttir, G., Johannsson, T., Arason, Þ., Gudmundsson, M.T., Oddsson, B., Þrastarson, R.H., Ófeigsson, B.G., Vogfjörd, K., Geirsson, H., Hjörvar, T., von Löwis, S., Petersen, G.N., Sigurðsson, E.M. (2023) The eruption in Fagradalsfjall (2021, Iceland): how the operational monitoring and the volcanic hazard assessment contributed to its safe access. Natural Hazards 116, 3063–3092. https://doi.org/10.1007/s11069-022-05798-7
). To assess the health hazard for potential future eruptions, we built seasonal wind roses, for the period 2012–2022, reflecting dominant wind speeds and directions in the RP (Hersbach et al., 2023Hersbach, H., Bell, B., Berrisford, P., Biavati, G., Horányi, A., Muñoz Sabater, J., Nicolas, J., Peubey, C., Radu, R., Rozum, I., Schepers, D., Simmons, A., Soci, C., Dee, D., Thépaut, J.-N. (2023) ERA5 hourly data on pressure levels from 1940 to present. Copernicus Climate Change Service (C3S) Climate Data Store (CDS). Accessed 26 October 2023. https://doi.org/10.24381/cds.bd0915c6
). We find that, most of the time, prevailing winds blow towards the NW–NE, suggesting different SO2 health hazard potentials associated with eruptions within different volcanic systems (Fig. 3). The prevalent NW wind blowing direction suggests that volcanic SO2 emissions could still be disruptive to the Keflavik Airport area if there were a long-duration eruption. Even if eruptions in the RP produce little ash, sulfate aerosol in the atmosphere could reduce visibility and air quality (Pattantyus et al., 2018Pattantyus, A.K., Businger, S., Howell, S.G. (2018) Review of sulfur dioxide to sulfate aerosol chemistry at Kīlauea Volcano, Hawai‘i. Atmospheric Environment 185, 262–271. https://doi.org/10.1016/j.atmosenv.2018.04.055
). Eruptions in Brennisteinsfjöll are the most hazardous for Reykjavík, especially in spring and autumn seasons, as SO2 is likely to be blown towards the capital area. Eruptions in Reykjanes pose a minimal hazard as winds tend to blow away from inhabited areas. During assessment of possible eruptive scenarios in the RP, our estimates provide key input parameters to model the release and dispersion of volcanic SO2 into the atmosphere. Our results can be used to inform SO2 pollution hazard assessments for potential eruptive scenarios and prompt action and mitigation plans during ongoing volcanic crises in the RP.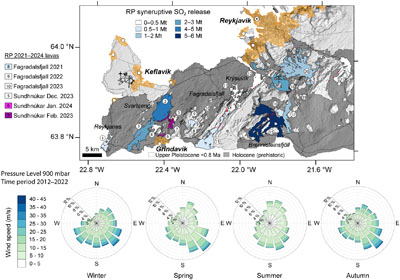
Figure 3 Simplified geological map of the RP and lava flows emplaced during the 800–1240 AD Fires. The map also illustrates the aerial extent of the 2021 (Pedersen et al., 2022)
Pedersen, G.B.M., Belart, J.M.C., Óskarsson, B.V., Gudmundsson, M.T., Gies, N., Högnadóttir, T., Hjartardóttir, Á.R., Pinel, V., Berthier, E., Dürig, T., Reynolds, H.I., Hamilton, C.W., Valsson, G., Einarsson, P., Ben-Yehosua, D., Gunnarsson, A., Oddsson, B. (2022) Volume, Effusion Rate, and Lava Transport During the 2021 Fagradalsfjall Eruption: Results From Near Real-Time Photogrammetric Monitoring. Geophysical Research Letters 49, e2021GL097125. https://doi.org/10.1029/2021GL097125
, 2022 (Gunnarson et al., 2023Gunnarson, S.R., Belart, J.M.C., Óskarsson, B.V., Gudmundsson, M.T., Högnadóttir, T., Pedersen, G.B.M., Dürig, T., Pinel, V. (2023) Automated processing of aerial imagery for geohazards monitoring: Results from Fagradalsfjall eruption, SW Iceland, August 2022 [Dataset]. Zenodo. https://doi.org/10.5281/zenodo.7871187
) and 2023 (Belart et al., 2023Belart, J.M.C., Pinel, V., Reynolds, H.I., Berthier, E., Gunnarson, S.R. (2023) Digital Elevation Models (DEMs) and lava outlines from the 2023 Litla-Hrútur eruption, Iceland, from Pléiades satellite stereoimages [Data set]. Zenodo. https://doi.org/10.5281/zenodo.10133203
) Fagradalsfjall lavas. Data from the 2023–2024 eruptions at Sundhnúksgígar are from the Landmælingar Íslands geoserver (gis.lmi.is/geoserver). When possible, lava flows are coloured according to calculated syneruptive SO2 emissions, ranging from 0.1 to 6 Mt. Orange shaded areas indicate urban areas. Numbers reflect the different erupted units as listed in Table 1. Seasonal wind roses reflect data at 900 mPa (∼1000 m a.s.l), which was the most common SO2 injection altitude during the 2021 Fagradalsfjall eruption (Esse et al., 2023Esse, B., Burton, M., Hayer, C., Pfeffer, M.A., Barsotti, S., Theys, N., Barnie, T., Titos, M. (2023) Satellite derived SO2 emissions from the relatively low-intensity, effusive 2021 eruption of Fagradalsfjall, Iceland. Earth and Planetary Science Letters 619, 118325. https://doi.org/10.1016/j.epsl.2023.118325
). Spokes indicate the direction the wind is blowing from, and the length of each spoke shows the frequency. Wind data were extracted from ERA5 (Hersbach et al., 2023Hersbach, H., Bell, B., Berrisford, P., Biavati, G., Horányi, A., Muñoz Sabater, J., Nicolas, J., Peubey, C., Radu, R., Rozum, I., Schepers, D., Simmons, A., Soci, C., Dee, D., Thépaut, J.-N. (2023) ERA5 hourly data on pressure levels from 1940 to present. Copernicus Climate Change Service (C3S) Climate Data Store (CDS). Accessed 26 October 2023. https://doi.org/10.24381/cds.bd0915c6
).top
Acknowledgements
This research was financially supported by a NordVulk fellowship awarded to AC and by the Icelandic Research Fund (grant 228933-052). We acknowledge support from the Gosvá project, a research programme on the assessment of volcanic hazard risks in Iceland led by the Icelandic Meteorological Office (IMO). SAH acknowledges support from the Icelandic Research Fund (Grant #196139-051). We thank Christoph Kern, two anonymous reviewers, and editor Ambre Luguet for their constructive comments, which significantly improved the quality of the manuscript.
Editor: Ambre Luguet
top
References
Bali, E., Hartley, M.E., Halldórsson, S.A., Gudfinnsson, G.H., Jakobsson, S. (2018) Melt inclusion constraints on volatile systematics and degassing history of the 2014–2015 Holuhraun eruption, Iceland. Contributions to Mineralogy and Petrology 173, 9. https://doi.org/10.1007/s00410-017-1434-1

By combining the mass of erupted magmas with the mass of S released, we can assess vent syneruptive SO2 emissions (MS) of individual eruptions (see Eqs. S-1, S-2) (e.g., Bali et al., 2018, and references therein). Furthermore, we calculate the magnitude of potential SO2 emissions (potential MS), which refers to complete degassing of all pre-eruptive sulfur (CS glass = 0) and reflects the maximum amount of SO2 that a specific eruption could potentially have released, assuming that there is no degassing of unerupted magma.
View in article
This reconstruction method has been shown to have matched field-based volatile measurements exceptionally well during the 2014–2015 Holuhraun eruption (Bali et al., 2018; Pfeffer et al., 2018) and the 2021 Fagradalsfjall eruption (this work, Table 1).
View in article
These are roughly between 20 and 70 % of the syneruptive SO2 emissions estimated for the 2014–2015 Holuhraun eruption (MS = 10.5 Mt; Bali et al., 2018).
View in article
Barsotti, S., Parks, M.M., Pfeffer, M.A., Óladóttir, B.A., Barnie, T., Titos, M.M., Jónsdóttir, K., Pedersen, G.B.M., Hjartardóttir, Á.R., Stefansdóttir, G., Johannsson, T., Arason, Þ., Gudmundsson, M.T., Oddsson, B., Þrastarson, R.H., Ófeigsson, B.G., Vogfjörd, K., Geirsson, H., Hjörvar, T., von Löwis, S., Petersen, G.N., Sigurðsson, E.M. (2023) The eruption in Fagradalsfjall (2021, Iceland): how the operational monitoring and the volcanic hazard assessment contributed to its safe access. Natural Hazards 116, 3063–3092. https://doi.org/10.1007/s11069-022-05798-7

Each volcanic system on the RP tends to activate during individual magmatic periods (Sæmundsson et al., 2020), and the recent 2021–2024 Fagradalsfjall and Svartsengi eruptions (Barsotti et al., 2023; Sigmundsson et al., 2024) suggest the potential initiation of a new eruptive period in an area that hosts ∼70 % of the Icelandic population.
View in article
We calculate syneruptive sulfur release and potential sulfur emissions of 19 geologically and petrochemically well characterised magmatic units (Peate et al., 2009; Caracciolo et al., 2023) and compare those with sulfur emissions from the 2021 Fagradalsfjall eruption (Halldórsson et al., 2022; Barsotti et al., 2023).
View in article
The syneruptive SO2 released by these latter voluminous lavas is approximately 2 to 6 times larger than syneruptive SO2 emissions during the 2021 Fagradalsfjall eruption, for which we estimated MS = 0.78 Mt (MS measured = 0.97 ± 0.5; Barsotti et al., 2023).
View in article
The estimate for the 2021 Fagradalsfjall eruption is in agreement with the majority of measured daily SO2 emissions throughout the 2021 Fagradalsfjall eruption, in the range of 1000–7600 t/day (Esse et al., 2023), and with daily SO2 emissions of 5240 ± 2700 t/day, calculated assuming 0.97 ± 0.5 Mt total mass of SO2 (Barsotti et al., 2023).
View in article
However, volcanic eruptions in the RP are potentially considered to be more hazardous due to their proximity to inhabited areas, to the international airport and to the large number of visitors expected at eruption sites (Fig. 3) (Barsotti et al., 2023).
View in article
Belart, J.M.C., Pinel, V., Reynolds, H.I., Berthier, E., Gunnarson, S.R. (2023) Digital Elevation Models (DEMs) and lava outlines from the 2023 Litla-Hrútur eruption, Iceland, from Pléiades satellite stereoimages [Data set]. Zenodo. https://doi.org/10.5281/zenodo.10133203

Simplified geological map of the RP and lava flows emplaced during the 800–1240 AD Fires. The map also illustrates the aerial extent of the 2021 (Pedersen et al., 2022), 2022 (Gunnarson et al., 2023) and 2023 (Belart et al., 2023) Fagradalsfjall lavas.
View in article
Caracciolo, A., Bali, E., Guðfinnsson, G.H., Kahl, M., Halldórsson, S.A., Hartley, M.E., Gunnarsson, H. (2020) Temporal evolution of magma and crystal mush storage conditions in the Bárðarbunga-Veiðivötn volcanic system, Iceland. Lithos 352–353, 105234. https://doi.org/10.1016/j.lithos.2019.105234

S was analysed by electron microprobe analyser (EMPA) at the University of Iceland, using the same analytical settings as in Caracciolo et al. (2020), and MI compositions have been corrected for post-entrapment processes (PEP) (Tables S-2–S-4) (Caracciolo et al., 2023).
View in article
Caracciolo, A., Bali, E., Halldórsson, S.A., Guðfinnsson, G.H., Kahl, M., Þórðardóttir, I., Pálmadóttir, G.L., Silvestri, V. (2023) Magma plumbing architectures and timescales of magmatic processes during historical magmatism on the Reykjanes Peninsula, Iceland. Earth and Planetary Science Letters 621, 118378. https://doi.org/10.1016/j.epsl.2023.118378

Here, we focus on magmatic units erupted in the volcanic systems of Reykjanes, Svartsengi, Krýsuvík and Brennisteinsfjöll in the RP during the last medieval eruptive cycle, which occurred between the 8th century and 1240 AD, hereafter referred to as the 800–1240 AD Fires (Peate et al., 2009; Caracciolo et al., 2023).
View in article
We calculate syneruptive sulfur release and potential sulfur emissions of 19 geologically and petrochemically well characterised magmatic units (Peate et al., 2009; Caracciolo et al., 2023) and compare those with sulfur emissions from the 2021 Fagradalsfjall eruption (Halldórsson et al., 2022; Barsotti et al., 2023).
View in article
Scoria samples were collected from multiple vents within individual eruptive units of the 800–1240 AD Fires (Table S-1) (Caracciolo et al., 2023).
View in article
Here, we present new sulfur (S) data for the same groundmass glass (n = 889) and melt inclusions (MIs) (n = 416) dataset published in Caracciolo et al. (2023).
View in article
S was analysed by electron microprobe analyser (EMPA) at the University of Iceland, using the same analytical settings as in Caracciolo et al. (2020), and MI compositions have been corrected for post-entrapment processes (PEP) (Tables S-2–S-4) (Caracciolo et al., 2023).
View in article
Considering that medieval and recent eruptions on the RP are likely sourced from mantle-derived melts of diverse compositions (Peate et al., 2009; Halldórsson et al., 2022; Harðardóttir et al., 2022; Caracciolo et al., 2023), including melts with variable S contents (Ranta et al., 2022), we use our MI record to estimate S contents of the local enriched and depleted end member melt components.
View in article
Modelling of S degassing with Sulfur_X (Ding et al., 2023) suggests that the basaltic melts that erupted during the 800–1240 AD Reykjanes Fires are unlikely to degas significant amounts of S at known pre-eruptive magma storage depths (Caracciolo et al., 2023) and that significant S degassing only takes place during magma ascent in the last 0.2 kbar (<700 m) (Fig. S-1).
View in article
Carlsen, H.K., Valdimarsdóttir, U., Briem, H., Dominici, F., Finnbjornsdottir, R.G., Jóhannsson, T., Aspelund, T., Gislason, T., Gudnason, T. (2021) Severe volcanic SO2 exposure and respiratory morbidity in the Icelandic population – a register study. Environmental Health 20, 23. https://doi.org/10.1186/s12940-021-00698-y

For example, several studies have associated cardiorespiratory issues with volcanic sulfur emissions (e.g., Carlsen et al., 2021, and references therein).
View in article
Devine, J.D., Sigurdsson, H., Davis, A.N., Self, S. (1984) Estimates of sulfur and chlorine yield to the atmosphere from volcanic eruptions and potential climatic effects. Journal of Geophysical Research: Solid Earth 89, 6309–6325. https://doi.org/10.1029/JB089iB07p06309

Here, we use the ‘petrological method’ (Devine et al., 1984) to calculate eruptive sulfur emissions based on the difference between S concentrations in mineral-hosted MIs and S concentrations measured in groundmass glass (ΔCS).
View in article
Ding, S., Plank, T., Wallace, P.J., Rasmussen, D.J. (2023) Sulfur_X: A Model of Sulfur Degassing During Magma Ascent. Geochemistry, Geophysics, Geosystems 24, e2022GC010552. https://doi.org/10.1029/2022GC010552

Modelling of S degassing with Sulfur_X (Ding et al., 2023) suggests that the basaltic melts that erupted during the 800–1240 AD Reykjanes Fires are unlikely to degas significant amounts of S at known pre-eruptive magma storage depths (Caracciolo et al., 2023) and that significant S degassing only takes place during magma ascent in the last 0.2 kbar (<700 m) (Fig. S-1).
View in article
Einarsson, S., Johannesson, H., Sveinbjörnsdóttir, Á.E. (1991) Krísuvíkureldar II. Kapelluhraun og gátan um aldur Hellnahrauns. Jökull 41, 61–80. https://doi.org/10.33799/jokull1991.41.061

Lava volumes for the medieval eruptions are from Einarsson et al. (1991), Jónsson (1978) and Sigurgeirsson (2004).
View in article
Esse, B., Burton, M., Hayer, C., Pfeffer, M.A., Barsotti, S., Theys, N., Barnie, T., Titos, M. (2023) Satellite derived SO2 emissions from the relatively low-intensity, effusive 2021 eruption of Fagradalsfjall, Iceland. Earth and Planetary Science Letters 619, 118325. https://doi.org/10.1016/j.epsl.2023.118325

Blue histogram indicates measured SO2 emissions during the 2021 Fagradalsfjall eruption (Esse et al., 2023). Data are coloured according to the volcanic system and only lavas with known volumes or MORs are included in the plots.
View in article
The estimate for the 2021 Fagradalsfjall eruption is in agreement with the majority of measured daily SO2 emissions throughout the 2021 Fagradalsfjall eruption, in the range of 1000–7600 t/day (Esse et al., 2023), and with daily SO2 emissions of 5240 ± 2700 t/day, calculated assuming 0.97 ± 0.5 Mt total mass of SO2 (Barsotti et al., 2023).
View in article
Seasonal wind roses reflect data at 900 mPa (∼1000 m a.s.l), which was the most common SO2 injection altitude during the 2021 Fagradalsfjall eruption (Esse et al., 2023).
View in article
Gunnarson, S.R., Belart, J.M.C., Óskarsson, B.V., Gudmundsson, M.T., Högnadóttir, T., Pedersen, G.B.M., Dürig, T., Pinel, V. (2023) Automated processing of aerial imagery for geohazards monitoring: Results from Fagradalsfjall eruption, SW Iceland, August 2022 [Dataset]. Zenodo. https://doi.org/10.5281/zenodo.7871187

Simplified geological map of the RP and lava flows emplaced during the 800–1240 AD Fires. The map also illustrates the aerial extent of the 2021 (Pedersen et al., 2022), 2022 (Gunnarson et al., 2023) and 2023 (Belart et al., 2023) Fagradalsfjall lavas.
View in article
Halldórsson, S.A., Marshall, E.W., Caracciolo, A., Matthews, S., Bali, E., Rasmussen, M.B., Ranta, E., Robin, J.G., Guðfinnsson, G.H., Sigmarsson, O., Maclennan, J., Jackson, M.G., Whitehouse, M.J., Jeon, H., van der Meer, Q.H.A., Mibei, G.K., Kalliokoski, M.H., Repczynska, M.M., Rúnarsdóttir, R.H., Sigurðsson, G., Pfeffer, M.A., Scott, S.W., Kjartansdóttir, R., Kleine, B.I., Oppenheimer, C., Aiuppa, A., Ilyinskaya, E., Bitetto, M., Giudice, G., Stefánsson, A. (2022) Rapid shifting of a deep magmatic source at Fagradalsfjall volcano, Iceland. Nature 609, 529–534. https://doi.org/10.1038/s41586-022-04981-x

We calculate syneruptive sulfur release and potential sulfur emissions of 19 geologically and petrochemically well characterised magmatic units (Peate et al., 2009; Caracciolo et al., 2023) and compare those with sulfur emissions from the 2021 Fagradalsfjall eruption (Halldórsson et al., 2022; Barsotti et al., 2023).
View in article
Data from the 2021 Fagradalsfjall eruption are from Halldórsson et al. (2022).
View in article
Considering that medieval and recent eruptions on the RP are likely sourced from mantle-derived melts of diverse compositions (Peate et al., 2009; Halldórsson et al., 2022; Harðardóttir et al., 2022; Caracciolo et al., 2023), including melts with variable S contents (Ranta et al., 2022), we use our MI record to estimate S contents of the local enriched and depleted end member melt components.
View in article
We distinguish between these components from the K2O/TiO2 variability, a robust tracer of mantle heterogeneities in Iceland (Halldórsson et al., 2022; Harðardóttir et al., 2022) (see Supplementary Information).
View in article
Based on the MI record of the 2021–2023 Fagradalsfjall eruptions (Halldórsson et al., 2022; this work), the 2023–2024 eruptions at Sundhnúksgígar and the 800–1240 AD Fires (this work), we constrain potential maximum (1900 ppm) and minimum (1170 ppm) pre-eruptive S concentrations and use these to estimate potential MS of future eruptions in the RP.
View in article
Harðardóttir, S., Matthews, S., Halldórsson, S.A., Jackson, M.G. (2022) Spatial distribution and geochemical characterization of Icelandic mantle end-members: Implications for plume geometry and melting processes. Chemical Geology 604, 120930. https://doi.org/10.1016/j.chemgeo.2022.120930

Considering that medieval and recent eruptions on the RP are likely sourced from mantle-derived melts of diverse compositions (Peate et al., 2009; Halldórsson et al., 2022; Harðardóttir et al., 2022; Caracciolo et al., 2023), including melts with variable S contents (Ranta et al., 2022), we use our MI record to estimate S contents of the local enriched and depleted end member melt components.
View in article
We distinguish between these components from the K2O/TiO2 variability, a robust tracer of mantle heterogeneities in Iceland (Halldórsson et al., 2022; Harðardóttir et al., 2022) (see Supplementary Information).
View in article
Hersbach, H., Bell, B., Berrisford, P., Biavati, G., Horányi, A., Muñoz Sabater, J., Nicolas, J., Peubey, C., Radu, R., Rozum, I., Schepers, D., Simmons, A., Soci, C., Dee, D., Thépaut, J.-N. (2023) ERA5 hourly data on pressure levels from 1940 to present. Copernicus Climate Change Service (C3S) Climate Data Store (CDS). Accessed 26 October 2023. https://doi.org/10.24381/cds.bd0915c6

To assess the health hazard for potential future eruptions, we built seasonal wind roses, for the period 2012–2022, reflecting dominant wind speeds and directions in the RP (Hersbach et al., 2023).
View in article
Spokes indicate the direction the wind is blowing from, and the length of each spoke shows the frequency. Wind data were extracted from ERA5 (Hersbach et al., 2023).
View in article
Horwell, C.J., Baxter, P.J., Damby, D.E., Elias, T., Ilyinskaya, E., Sparks, R.S.J., Stewart, C., Tomašek, I. (2023) The International Volcanic Health Hazard Network (IVHHN): reflections on 20 years of progress. Frontiers in Earth Science 11, 1213363. https://doi.org/10.3389/feart.2023.1213363

Among volcanic gases, sulfur species (SO2, H2S) and associated aerosols (SO4, H2SO4) are the most critical airborne hazards to human health, with short- and long-term impacts that have been recorded at variable distances from eruptive vents (e.g., Schmidt et al., 2015; Ilyinskaya et al., 2017; Stewart et al., 2022; Horwell et al., 2023).
View in article
Ilyinskaya, E., Schmidt, A., Mather, T.A., Pope, F.D., Witham, C., Baxter, P., Jóhannsson, T., Pfeffer, M., Barsotti, S., Singh, A., Sanderson, P., Bergsson, B., McCormick Kilbride, B., Donovan, A., Peters, N., Oppenheimer, C., Edmonds, M. (2017) Understanding the environmental impacts of large fissure eruptions: Aerosol and gas emissions from the 2014–2015 Holuhraun eruption (Iceland). Earth and Planetary Science Letters 472, 309–322. https://doi.org/10.1016/j.epsl.2017.05.025

The release of volcanic gases and aerosols during volcanic eruptions can significantly impact the air quality and climate (e.g., Ilyinskaya et al., 2017), as well as biodiversity (e.g., Weiser et al., 2022).
View in article
Among volcanic gases, sulfur species (SO2, H2S) and associated aerosols (SO4, H2SO4) are the most critical airborne hazards to human health, with short- and long-term impacts that have been recorded at variable distances from eruptive vents (e.g., Schmidt et al., 2015; Ilyinskaya et al., 2017; Stewart et al., 2022; Horwell et al., 2023).
View in article
Jónsson, J. (1978) Jarðfræðikort af Reykjanesskaga : 1. Skýringar við jarðfræðikort ; 2. Jarðfræðikort. Orkustofnun jarðhitadeild, Reykjavík. http://hdl.handle.net/10802/4981.

Lava volumes for the medieval eruptions are from Einarsson et al. (1991), Jónsson (1978) and Sigurgeirsson (2004).
View in article
Óskarsson, B.V., Askew, R.A., Guðmundsson, H. (2024) Assessing the mean output rate (MOR) of past effusive basaltic eruptions - a look at the postglacial volcanism of the Reykjanes Peninsula in Iceland. EarthArXiv Preprint v1. https://doi.org/10.31223/X5CH68

(c) Daily SO2 emissions are calculated using MOR values and associated uncertainties from Óskarsson et al. (2024).
View in article
We have estimated daily SO2 emissions for the 800–1240 AD Fires using MOR values calculated by Oskarsson et al. (2024), in the range of 3–119 m3/s (Table 1, Eq. S-3).
View in article
Pattantyus, A.K., Businger, S., Howell, S.G. (2018) Review of sulfur dioxide to sulfate aerosol chemistry at Kīlauea Volcano, Hawai‘i. Atmospheric Environment 185, 262–271. https://doi.org/10.1016/j.atmosenv.2018.04.055

Even if eruptions in the RP produce little ash, sulfate aerosol in the atmosphere could reduce visibility and air quality (Pattantyus et al., 2018).
View in article
Peate, D.W., Baker, J.A., Jakobsson, S.P., Waight, T.E., Kent, A.J.R., Grassineau, N.V., Skovgaard, A.C. (2009) Historic magmatism on the Reykjanes Peninsula, Iceland: a snap-shot of melt generation at a ridge segment. Contributions to Mineralogy and Petrology 157, 359–382. https://doi.org/10.1007/s00410-008-0339-4

Here, we focus on magmatic units erupted in the volcanic systems of Reykjanes, Svartsengi, Krýsuvík and Brennisteinsfjöll in the RP during the last medieval eruptive cycle, which occurred between the 8th century and 1240 AD, hereafter referred to as the 800–1240 AD Fires (Peate et al., 2009; Caracciolo et al., 2023).
View in article
We calculate syneruptive sulfur release and potential sulfur emissions of 19 geologically and petrochemically well characterised magmatic units (Peate et al., 2009; Caracciolo et al., 2023) and compare those with sulfur emissions from the 2021 Fagradalsfjall eruption (Halldórsson et al., 2022; Barsotti et al., 2023).
View in article
Considering that medieval and recent eruptions on the RP are likely sourced from mantle-derived melts of diverse compositions (Peate et al., 2009; Halldórsson et al., 2022; Harðardóttir et al., 2022; Caracciolo et al., 2023), including melts with variable S contents (Ranta et al., 2022), we use our MI record to estimate S contents of the local enriched and depleted end member melt components.
View in article
Pedersen, G.B.M., Belart, J.M.C., Óskarsson, B.V., Gudmundsson, M.T., Gies, N., Högnadóttir, T., Hjartardóttir, Á.R., Pinel, V., Berthier, E., Dürig, T., Reynolds, H.I., Hamilton, C.W., Valsson, G., Einarsson, P., Ben-Yehosua, D., Gunnarsson, A., Oddsson, B. (2022) Volume, Effusion Rate, and Lava Transport During the 2021 Fagradalsfjall Eruption: Results From Near Real-Time Photogrammetric Monitoring. Geophysical Research Letters 49, e2021GL097125. https://doi.org/10.1029/2021GL097125

Simplified geological map of the RP and lava flows emplaced during the 800–1240 AD Fires. The map also illustrates the aerial extent of the 2021 (Pedersen et al., 2022), 2022 (Gunnarson et al., 2023) and 2023 (Belart et al., 2023) Fagradalsfjall lavas.
View in article
V and MOR from Pedersen et al. (2022).
View in article
Pedersen, G.B.M., Belart, J.M.C., Óskarsson, B.V., Gunnarson, S.R., Gudmundsson, M.T., Reynolds, H.I., Valsson, G., Högnadóttir, T., Pinel, V., Parks, M.M., Drouin, V., Askew, R.A., Dürig, T., Þrastarson, R.H. (2024) Volume, effusion rates and lava hazards of the 2021, 2022 and 2023 Reykjanes fires: Lessons learned from near real-time photogrammetric monitoring. EGU General Assembly 2024, Vienna, Austria, 14–19 April 2024, Abstract EGU24-10724. https://doi.org/10.5194/egusphere-egu24-10724

V and MOR from Pedersen et al. (2024).
View in article
Pfeffer, M.A., Bergsson, B., Barsotti, S., Stefánsdóttir, G., Galle, B., Arellano, S., Conde, V., Donovan, A., Ilyinskaya, E., Burton, M., Aiuppa, A., Whitty, R.C.W., Simmons, I.C., Arason, Þ., Jónasdóttir, E.B., Keller, N.S., Yeo, R.F., Arngrímsson, H., Jóhannsson, Þ., Butwin, M.K., Askew, R.A., Dumont, S., Von Löwis, S., Ingvarsson, Þ., La Spina, A., Thomas, H., Prata, F., Grassa, F., Giudice, G., Stefánsson, A., Marzano, F., Montopoli, M., Mereu, L. (2018) Ground-Based Measurements of the 2014–2015 Holuhraun Volcanic Cloud (Iceland). Geosciences 8, 29. https://doi.org/10.3390/geosciences8010029

This reconstruction method has been shown to have matched field-based volatile measurements exceptionally well during the 2014–2015 Holuhraun eruption (Bali et al., 2018; Pfeffer et al., 2018) and the 2021 Fagradalsfjall eruption (this work, Table 1).
View in article
SO2 emissions during the 800–1240 AD Fires and the 2021–2024 eruptions are small compared to those during the 2014–2015 Holuhraun basaltic eruption (9.2 Mt SO2; Pfeffer et al., 2018).
View in article
Ranta, E., Gunnarsson-Robin, J., Halldórsson, S.A., Ono, S., Izon, G., Jackson, M.G., Reekie, C.D.J., Jenner, F.E., Guðfinnsson, G.H., Jónsson, Ó.P., Stefánsson, A. (2022) Ancient and recycled sulfur sampled by the Iceland mantle plume. Earth and Planetary Science Letters 584, 117452. https://doi.org/10.1016/j.epsl.2022.117452

Considering that medieval and recent eruptions on the RP are likely sourced from mantle-derived melts of diverse compositions (Peate et al., 2009; Halldórsson et al., 2022; Harðardóttir et al., 2022; Caracciolo et al., 2023), including melts with variable S contents (Ranta et al., 2022), we use our MI record to estimate S contents of the local enriched and depleted end member melt components.
View in article
Ranta, E., Halldórsson, S.A., Óladóttir, B.A., Pfeffer, M.A., Caracciolo, A., Bali, E., Guðfinnsson, G.H., Kahl, M., Barsotti, S. (2024) Magmatic Controls on Volcanic Sulfur Emissions at the Iceland Hotspot. EarthArXiv Preprint v1. https://doi.org/10.31223/X51102

Sulfur release ranges between 1000 and 1770 ppm across the RP, a typical range for Icelandic rift basalts (Ranta et al., 2024), with the largest ΔCS found in lavas from Svartsengi and Brennisteinsfjöll (Table 1).
View in article
Sæmundsson, K., Sigurgeirsson, M.Á., Friðleifsson, G.Ó. (2020) Geology and structure of the Reykjanes volcanic system, Iceland. Journal of Volcanology and Geothermal Research 391, 106501. https://doi.org/10.1016/j.jvolgeores.2018.11.022

The latest magmatic period in the RP occurred ∼800 years ago (Sæmundsson et al., 2020), but knowledge about sulfur outputs during those eruptions has been lacking thus far.
View in article
Each volcanic system on the RP tends to activate during individual magmatic periods (Sæmundsson et al., 2020), and the recent 2021–2024 Fagradalsfjall and Svartsengi eruptions (Barsotti et al., 2023; Sigmundsson et al., 2024) suggest the potential initiation of a new eruptive period in an area that hosts ∼70 % of the Icelandic population.
View in article
Schmidt, A., Leadbetter, S., Theys, N., Carboni, E., Witham, C.S., Stevenson, J.A., Birch, C.E., Thordarson, T., Turnock, S., Barsotti, S., Delaney, L., Feng, W., Grainger, R.G., Hort, M.C., Höskuldsson, Á., Ialongo, I., Ilyinskaya, E., Jóhannsson, T., Kenny, P., Mather, T.A., Richards, N.A.D., Shepherd, J. (2015) Satellite detection, long-range transport, and air quality impacts of volcanic sulfur dioxide from the 2014–2015 flood lava eruption at Bárðarbunga (Iceland). Journal of Geophysical Research: Atmospheres 120, 9739–9757. https://doi.org/10.1002/2015JD023638

Among volcanic gases, sulfur species (SO2, H2S) and associated aerosols (SO4, H2SO4) are the most critical airborne hazards to human health, with short- and long-term impacts that have been recorded at variable distances from eruptive vents (e.g., Schmidt et al., 2015; Ilyinskaya et al., 2017; Stewart et al., 2022; Horwell et al., 2023).
View in article
Sigmundsson, F., Parks, M., Geirsson, H., Hooper, A., Drouin, V., Vogfjörd, K.S., Ófeigsson, B.G., Greiner, S.H.M., Yang, Y., Lanzi, C., De Pascale, G.P., Jónsdóttir, K., Hreinsdóttir, S., Tolpekin, V., Friðriksdóttir, H.M., Einarsson, P., Barsotti, S. (2024) Fracturing and tectonic stress drives ultrarapid magma flow into dikes. Science 383, 1228–1235. https://doi.org/10.1126/science.adn2838

Each volcanic system on the RP tends to activate during individual magmatic periods (Sæmundsson et al., 2020), and the recent 2021–2024 Fagradalsfjall and Svartsengi eruptions (Barsotti et al., 2023; Sigmundsson et al., 2024) suggest the potential initiation of a new eruptive period in an area that hosts ∼70 % of the Icelandic population.
View in article
Sigurgeirsson, M.Á. (2004) Þáttur úr gossögu Reykjaness. Náttúrufræðingurinn 72, 21–28. https://timarit.is/gegnir/991000816469706886.

Lava volumes for the medieval eruptions are from Einarsson et al. (1991), Jónsson (1978) and Sigurgeirsson (2004).
View in article
Smythe, D.J., Wood, B.J., Kiseeva, E.S. (2017) The S content of silicate melts at sulfide saturation: New experiments and a model incorporating the effects of sulfide composition. American Mineralogist 102, 795–803. https://doi.org/10.2138/am-2017-5800CCBY

The black dotted curve indicates SCSS along an empirical fractional crystallisation path calculated after Smythe et al. (2017), implemented in PySulfSat (Wieser and Gleeson, 2022).
View in article
In order to evaluate S saturation during magma ascent and fractional crystallisation through the crust, we calculate sulfur content at sulfide saturation (SCSS) along a FC path, which reflects the amount of S2− present in a melt in equilibrium with a sulfide phase (Smythe et al., 2017) (see Supplementary Information).
View in article
Stewart, C., Damby, D.E., Horwell, C.J., Elias, T., Ilyinskaya, E., Tomašek, I., Longo, B.M., Schmidt, A., Carlsen, H.K., Mason, E., Baxter, P.J., Cronin, S., Witham, C. (2022) Volcanic air pollution and human health: recent advances and future directions. Bulletin of Volcanology 84, 11. https://doi.org/10.1007/s00445-021-01513-9

Among volcanic gases, sulfur species (SO2, H2S) and associated aerosols (SO4, H2SO4) are the most critical airborne hazards to human health, with short- and long-term impacts that have been recorded at variable distances from eruptive vents (e.g., Schmidt et al., 2015; Ilyinskaya et al., 2017; Stewart et al., 2022; Horwell et al., 2023).
View in article
Weiser, F., Baumann, E., Jentsch, A., Medina, F.M., Lu, M., Nogales, M., Beierkuhnlein, C. (2022) Impact of Volcanic Sulfur Emissions on the Pine Forest of La Palma, Spain. Forests 13, 299. https://doi.org/10.3390/f13020299

The release of volcanic gases and aerosols during volcanic eruptions can significantly impact the air quality and climate (e.g., Ilyinskaya et al., 2017), as well as biodiversity (e.g., Weiser et al., 2022).
View in article
Wieser, P.E., Gleeson, M. (2022) PySulfSat: An open-source Python3 tool for modeling sulfide and sulfate saturation. Volcanica 6, 107–127. https://doi.org/10.30909/vol.06.01.107127

The black dotted curve indicates SCSS along an empirical fractional crystallisation path calculated after Smythe et al. (2017), implemented in PySulfSat (Wieser and Gleeson, 2022).
View in article
top
Supplementary Information
The Supplementary Information includes:
- Calculation of SO2 Emissions
- Modelling Sulfur Degassing
- The Occurrence of Degassed and Partially Degassed Melt Inclusions
- Selection of Enriched and Depleted End Member Melt Compositions
- Modelling SCSS
- Figures S-1 to S-4
- Supplementary Tables S-1 to S-4
- Supplementary Information References
Download the Supplementary Information (PDF)
Download Tables S-1 to S-4 (.xlsx)
Figures
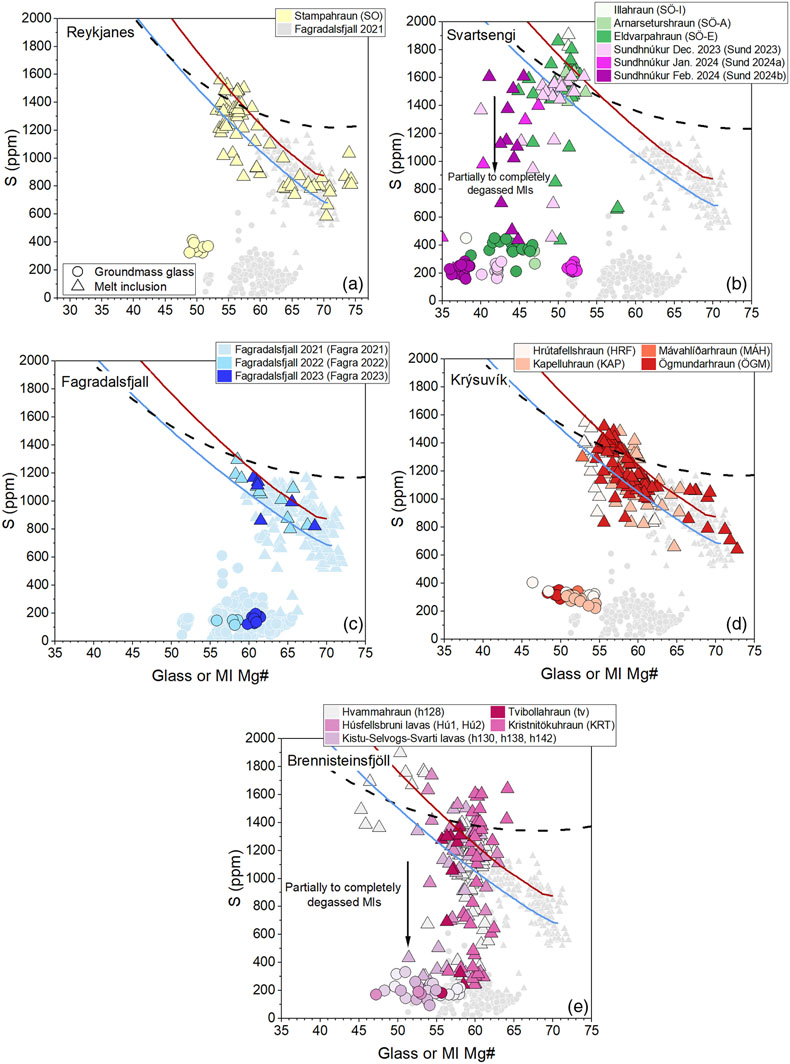
Figure 1 Variations of S contents in groundmass glasses (filled circles) and PEP-corrected MIs (filled triangles) as a function of Mg# [Mg# = 100·Mg/(Mg+Fe2+), Fe2+/Fetot = 0.9] in samples from (a,b,d,e) the 800–1240 AD Fires, (c) the 2021–2023 Fagradalsfjall eruptions and (b) the 2023–2024 eruptions at Sundhnúksgígar. Data from the 2021 Fagradalsfjall eruption are from Halldórsson et al. (2022)
Halldórsson, S.A., Marshall, E.W., Caracciolo, A., Matthews, S., Bali, E., Rasmussen, M.B., Ranta, E., Robin, J.G., Guðfinnsson, G.H., Sigmarsson, O., Maclennan, J., Jackson, M.G., Whitehouse, M.J., Jeon, H., van der Meer, Q.H.A., Mibei, G.K., Kalliokoski, M.H., Repczynska, M.M., Rúnarsdóttir, R.H., Sigurðsson, G., Pfeffer, M.A., Scott, S.W., Kjartansdóttir, R., Kleine, B.I., Oppenheimer, C., Aiuppa, A., Ilyinskaya, E., Bitetto, M., Giudice, G., Stefánsson, A. (2022) Rapid shifting of a deep magmatic source at Fagradalsfjall volcano, Iceland. Nature 609, 529–534. https://doi.org/10.1038/s41586-022-04981-x
. Red and blue solid lines indicate fractional crystallisation paths calculated for geochemically enriched and depleted initial melt compositions, respectively (see Supplementary Information). The black dotted curve indicates SCSS along an empirical fractional crystallisation path calculated after Smythe et al. (2017)Smythe, D.J., Wood, B.J., Kiseeva, E.S. (2017) The S content of silicate melts at sulfide saturation: New experiments and a model incorporating the effects of sulfide composition. American Mineralogist 102, 795–803. https://doi.org/10.2138/am-2017-5800CCBY
, implemented in PySulfSat (Wieser and Gleeson, 2022Wieser, P.E., Gleeson, M. (2022) PySulfSat: An open-source Python3 tool for modeling sulfide and sulfate saturation. Volcanica 6, 107–127. https://doi.org/10.30909/vol.06.01.107127
).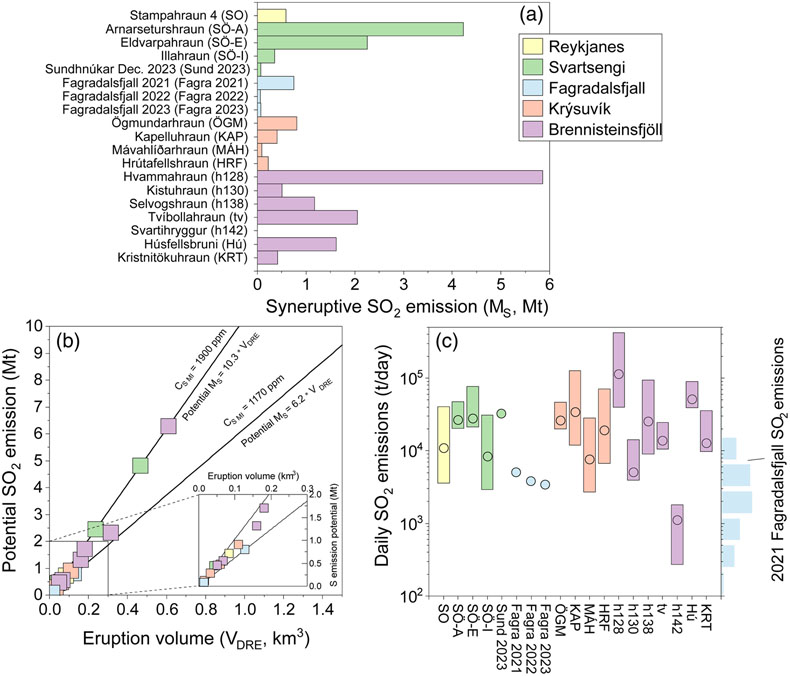
Figure 2 (a) Variation of vent MS. (b) Magnitude of potential MS as a function of eruption volume for the 800–1240 AD Fires, the 2021–2023 Fagradalsfjall eruptions and the 2023 Sundhnúkar eruption. At a given volume, straight lines allow to calculate potential MS corresponding to maximum and minimum pre-eruptive S concentrations measured across the RP. Inset plot shows most common potential MS across the RP. (c) Daily SO2 emissions are calculated using MOR values and associated uncertainties from Óskarsson et al. (2024)
Óskarsson, B.V., Askew, R.A., Guðmundsson, H. (2024) Assessing the mean output rate (MOR) of past effusive basaltic eruptions - a look at the postglacial volcanism of the Reykjanes Peninsula in Iceland. EarthArXiv Preprint v1. https://doi.org/10.31223/X5CH68
. Blue histogram indicates measured SO2 emissions during the 2021 Fagradalsfjall eruption (Esse et al., 2023Esse, B., Burton, M., Hayer, C., Pfeffer, M.A., Barsotti, S., Theys, N., Barnie, T., Titos, M. (2023) Satellite derived SO2 emissions from the relatively low-intensity, effusive 2021 eruption of Fagradalsfjall, Iceland. Earth and Planetary Science Letters 619, 118325. https://doi.org/10.1016/j.epsl.2023.118325
). Data are coloured according to the volcanic system and only lavas with known volumes or MORs are included in the plots.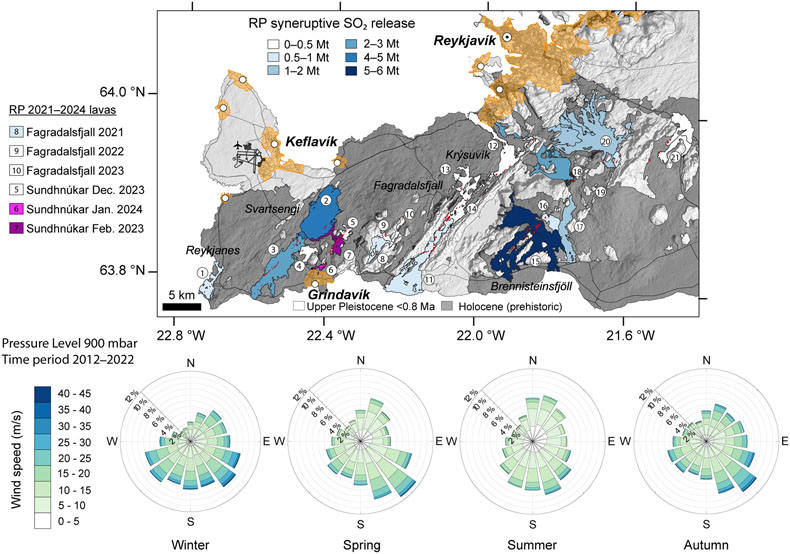
Figure 3 Simplified geological map of the RP and lava flows emplaced during the 800–1240 AD Fires. The map also illustrates the aerial extent of the 2021 (Pedersen et al., 2022)
Pedersen, G.B.M., Belart, J.M.C., Óskarsson, B.V., Gudmundsson, M.T., Gies, N., Högnadóttir, T., Hjartardóttir, Á.R., Pinel, V., Berthier, E., Dürig, T., Reynolds, H.I., Hamilton, C.W., Valsson, G., Einarsson, P., Ben-Yehosua, D., Gunnarsson, A., Oddsson, B. (2022) Volume, Effusion Rate, and Lava Transport During the 2021 Fagradalsfjall Eruption: Results From Near Real-Time Photogrammetric Monitoring. Geophysical Research Letters 49, e2021GL097125. https://doi.org/10.1029/2021GL097125
, 2022 (Gunnarson et al., 2023Gunnarson, S.R., Belart, J.M.C., Óskarsson, B.V., Gudmundsson, M.T., Högnadóttir, T., Pedersen, G.B.M., Dürig, T., Pinel, V. (2023) Automated processing of aerial imagery for geohazards monitoring: Results from Fagradalsfjall eruption, SW Iceland, August 2022 [Dataset]. Zenodo. https://doi.org/10.5281/zenodo.7871187
) and 2023 (Belart et al., 2023Belart, J.M.C., Pinel, V., Reynolds, H.I., Berthier, E., Gunnarson, S.R. (2023) Digital Elevation Models (DEMs) and lava outlines from the 2023 Litla-Hrútur eruption, Iceland, from Pléiades satellite stereoimages [Data set]. Zenodo. https://doi.org/10.5281/zenodo.10133203
) Fagradalsfjall lavas. Data from the 2023–2024 eruptions at Sundhnúksgígar are from the Landmælingar Íslands geoserver (gis.lmi.is/geoserver). When possible, lava flows are coloured according to calculated syneruptive SO2 emissions, ranging from 0.1 to 6 Mt. Orange shaded areas indicate urban areas. Numbers reflect the different erupted units as listed in Table 1. Seasonal wind roses reflect data at 900 mPa (∼1000 m a.s.l), which was the most common SO2 injection altitude during the 2021 Fagradalsfjall eruption (Esse et al., 2023Esse, B., Burton, M., Hayer, C., Pfeffer, M.A., Barsotti, S., Theys, N., Barnie, T., Titos, M. (2023) Satellite derived SO2 emissions from the relatively low-intensity, effusive 2021 eruption of Fagradalsfjall, Iceland. Earth and Planetary Science Letters 619, 118325. https://doi.org/10.1016/j.epsl.2023.118325
). Spokes indicate the direction the wind is blowing from, and the length of each spoke shows the frequency. Wind data were extracted from ERA5 (Hersbach et al., 2023Hersbach, H., Bell, B., Berrisford, P., Biavati, G., Horányi, A., Muñoz Sabater, J., Nicolas, J., Peubey, C., Radu, R., Rozum, I., Schepers, D., Simmons, A., Soci, C., Dee, D., Thépaut, J.-N. (2023) ERA5 hourly data on pressure levels from 1940 to present. Copernicus Climate Change Service (C3S) Climate Data Store (CDS). Accessed 26 October 2023. https://doi.org/10.24381/cds.bd0915c6
).